Fig. 1
Diagrammatical representation of the ascending aorta and in particular of the aortic root. (a: sinotubular junction; b: the sinuses of Valsalva; c: Ventriculo-aortic junction, also called basal ring or surgical annulus)
The aorta as an organ can be regarded as an elastic reservoir, storing kinetic energy during systole which is delivered during diastole, in order to convert the high velocity (around 1 m/s) pulsatile flow at the level of the ascending aorta to a low velocity (around 0.01 cm/s) steady flow necessary to cellular exchanges. This systolic-diastolic interplay has been defined “windkessel” effect by analogy with an old-fashioned hand-pumped fire engine (in German “windkessel” pump), that firemen used to obtain a constant water stream (Fig. 2).


Fig. 2
Through the ‘windkessel’ effect, aorta acts as an elastic reservoir, converting the pulsatile ejection of blood into a steady stream of flow (see text for further explanations)
The windkessel effect helps in damping the fluctuation in blood pressure during the cardiac cycle and assists in the maintenance of coronary perfusion during diastole when cardiac ejection ceases.
In addition to the conductance and pumping functions, the aorta plays an important role in the control of systemic vascular resistance and heart rate, via pressure-responsive receptors located in the ascending aorta and aortic arch. An increase in aortic pressure results in a decrease in heart rate and systemic vascular resistance, whereas a decrease in aortic pressure results in an increase in heart rate and systemic vascular resistance (Erbel et al. 2014).
2 Thoracic Aortic Aneurysm and Dissection
Acute dissection (AD) is one of the most devastating diseases of the aorta. This condition is often lethal, even when emergency surgery can be performed. Indeed, it is regarded as one of the most dramatic life-threatening vascular emergencies, characterized by elevated mortality despite improvements in diagnostic imaging and surgical techniques (Erbel et al. 2014; Baguet et al. 2012; Howard et al. 2013; Landenhed et al. 2015).
Previous epidemiological studies of AD have been hospital-based, have had incomplete ascertainment due to exclusion of out-of-hospital cases and predated widespread use of modern diagnostic technology, such as CT angiography. The first prospective study of acute aortic disease was conducted in a population of 92,728 in Oxfordshire, UK, during 2002–2012, as part of the Oxford Vascular Study (OXVASC) (Howard et al. 2013). The incidence of AD (6 per 100, 000 people per year) and of ruptured/symptomatic aortic aneurysms (11 per 100,000 people per year) in this study was higher than previously estimated (Howard et al. 2013), probably due to more complete inclusion of deaths prior to hospital admission. It is also possible that improvements in vascular imaging might have contributed and that the incidence of AD may have increased since previous studies. Even higher incidence rates of AD (15 per 100,000 patient-years) were recorded in the prospective cohort of 30,142 middle-aged individuals participating in the Malmö Diet and Cancer study, during up to 20 years of follow-up (Landenhed et al. 2015). In the same study hypertension was present in 86 % of individuals who subsequently developed AD. High blood pressure (BP) was strongly associated with incident AD (hazard ratio 2.64, 95 % CI 1.33–5.25), and conferred a population‐attributable risk of 54 % (Landenhed et al. 2015). Similarly, in the OXVASC study it has been observed that uncontrolled hypertension was the most significant treatable risk factor for AD. Premorbid control of BP was often poor, even though 67.3 % of patients were prescribed antihypertensive medications (Howard et al. 2013).
An abrupt, transient, severe increase in BP has been associated with acute AD through various mechanisms. Crack cocaine, which may cause transient hypertension due to catecholamine release, accounted for 37 % of dissections in a report of an inner city population (Manning and Black 2016). High-intensity weight lifting or other strenuous resistance training can also cause a transient elevation in blood pressure and has been reported as an antecedent (Manning and Black 2016). Hypertension is also the postulated mechanism when energy drinks or ergotism have been associated with aortic dissection (Manning and Black 2016).
Pathophysiology of dissection involves the separation of the tunica intima from the tunica media with subsequent penetration of blood. We have only limited understanding, however, of the mechanobiological processes that lead to this dreaded condition. Besides the link with poorly controlled hypertension, thoracic aortic aneurysms and dissections are associated with mutations in genes for extracellular matrix constituents, membrane receptors, contractile proteins, and associated signalling molecules (Humphrey et al. 2015). This grouping of factors suggests that these thoracic aorta diseases result, in part, from dysfunctional mechanosensing and mechanoregulation of the extracellular matrix by the intramural cells, which leads to a compromised structural integrity of the wall (Humphrey et al. 2015).
Some of the genetic mutations above mentioned are responsible for several syndromic and nonsyndromic genetic conditions that are associated with the development of thoracic aortic aneurysms and present with dissections at smaller diameters than usual. These conditions include Marfan syndrome, bicuspid aortic valve, Turner syndrome, Loeys-Dietz syndrome, and other less common diseases (Erbel et al. 2014; Baguet et al. 2012; Manning and Black 2016).
To prevent dissection of the aorta, timely operation on a patient with a known dilatation of the ascending aorta is advised, along with other supportive measures (Erbel et al. 2014; Baguet et al. 2012).
The wall tension, given by the product of the circumferential stress and wall thickness, is directly proportional to BP and vessel diameter, thus explaining why both hypertension and aortic dilatation are risk factors for aortic dissection (Erbel et al. 2014; Baguet et al. 2012). Indeed, an increased aortic root diameter (ARD) represents the main risk factor for thoracic aortic dissection and rupture and for aortic valve regurgitation (Erbel et al. 2014; Baguet et al. 2012).
It should be emphasized that thoracic aortic dimension alone seems not to be sufficient to detect subjects at high risk of aortic rupture and dissection. In fact, the aging process of the proximal elastic arteries, characterized by the progressive fracture of the elastic lamellae, account for both dilation (from the stretch after the fracture of a load-bearing material) and stiffening (through the transfer of stresses to more rigid collagenous components of the arterial wall) (Erbel et al. 2014; Baguet et al. 2012).
Although aortic surgery is usually recommended when the ascending aortic diameter reaches 5.5 cm in non-Marfan patients and 4.5 cm in Marfan patients (Erbel et al. 2014; Baguet et al. 2012), it has been recently shown that dissecting aortas are often sized well below the diameters defined by these surgical guidelines (Pape et al. 2007; Davies et al. 2006).
The International Registry of Acute Aortic Dissection study demonstrated that among patients with acute type A aortic dissection, aortic diameter at presentation was < 5.5 cm in most cases and < 5.0 cm in 40 % of the cases (Pape et al. 2007).
The usual underlying histopathologic changes in aortic tissue associated with aortic AD and often also with thoracic aneurysms are defined as “cystic medial degeneration or necrosis”. These changes are known to occur to some extent with aging, but are accelerated by hypertension (Erbel et al. 2014; Baguet et al. 2012).
Although transthoracic echocardiography (TTE) is not the technique of choice for full assessment of the thoracic aorta, it is an excellent screening tool to evaluate aortic root morphology and dimensions, being widely available, cost effective, and safe. For these reasons, TTE is the most frequently used technique for measuring proximal aortic segments in clinical practice. It suffices to quantify maximum aortic root and proximal ascending aorta diameters when the acoustic window is adequate. Nevertheless, the technique is more limited for measuring the remaining aortic segments, for which transoesophageal echocardiography, computed tomography or magnetic resonance imaging are needed (Erbel et al. 2014; Baguet et al. 2012; Lang et al. 2015).
Measurements of aortic diameters are not always straightforward and some limitations inherent to all imaging techniques need to be acknowledged. No imaging modality has perfect resolution and reliable detection of aortic diameter at the same aortic segment over time requires standardized measurement; this includes similar determination of edges (inner-to-inner, or leading edge-to-leading edge, or outer-to-outer diameter measurement, according to the imaging modality). Whether the measurement should be done during systole or diastole has not yet been accurately assessed, but diastolic images give the best reproducibility (Erbel et al. 2014; Lang et al. 2015).
The available evidence regarding the prognostic role of aortic root size we will describe subsequently is mostly based on aortic root size measurements obtained by M-Mode echocardiography, under 2-dimensional control, at a single level (that is the widest point of Valsalva’s sinuses). It is now recognized (Erbel et al. 2014; Lang et al. 2015) that this approach yields measurements that are 1–2 mm smaller than that recorded by 2-dimensional echocardiography and is biased by translational movement of the aortic root. Moreover, it has been observed that the correlates of aortic root may differ if its measurement is taken at different sites (i.e. annulus, supra-aortic ridge and ascending aorta) (Milan et al. 2013; Kim et al. 1996; Vriz et al. 2013; Campens et al. 2014).
3 Aortic Root and Hypertension
Intuitively, remodelling of the aortic root may be expected to occur in hypertensive subjects as a consequence of increased stress on the aortic wall due to the repeated hemodynamic overload.
For this reason arterial hypertension is commonly regarded as a predisposing condition for the development of thoracic aorta aneurysms (Erbel et al. 2014; Baguet et al. 2012). Even though linking dilatation of aortic root to high BP seems straightforward, the role of hypertension as determinant of aortic root enlargement remains controversial (Erbel et al. 2014; Baguet et al. 2012; Milan et al. 2013; Kim et al. 1996; Vriz et al. 2013; Campens et al. 2014; Vasan et al. 1995; Lam et al. 2010; O’Rourke and Nichols 2005; Mitchell et al. 2003; 2008; Ingelsson et al. 2008; Farasat et al. 2008; Roman et al. 1987; Tell et al. 1994; Palmieri et al. 2001; Bella et al. 2002; Agmon et al. 2003; Savage et al. 1979; Biaggi et al. 2009).
Vasan et al., using two-dimensionally guided M-mode measurement of the sinuses of Valsalva in the Framingham Heart Study, found a small direct relation between diastolic pressure and ARD, whereas both systolic and pulse pressures (PP) were inversely related to ARD after adjustment for age, height, and weight (Vasan et al. 1995). These results were confirmed in a longitudinal analysis of the same study, where each 10-mmHg increase in diastolic BP was associated with a larger predicted ARD in men (0.39 mm) than in women (0.19 mmHg), after adjustment for all other clinical covariates. Moreover, 10-mmHg increase in PP was related to a smaller predicted aortic root diameter in men (0.19 mm) and women (0.08 mm), after adjustment for age, BMI, and antihypertensive therapy (Lam et al. 2010).
These observations are in contrast with the classic notion that age-related Elastinc fragmentation, passive aortic dilatation, wall stiffening, and premature wave reflection lead directly to increasing PP with age (O’Rourke and Nichols 2005). In line with this traditional view, aortic root enlargement and increase in pulse pressure are two closely related phenomena, both linked to vascular aging (O’Rourke and Nichols 2005). On the contrary, these findings have led to the hypothesis that a smaller aortic root may play a key role in the pathogenesis of systolic hypertension by introducing a mismatch between ARD and blood flow so that forward wave amplitude is increased (Mitchell et al. 2003). Indeed, direct measurements of pulsatile hemodynamics in patients with systolic hypertension showed that although aortic root dilatation and stiffening occurred with increasing age, higher PP was associated with increased characteristic impedance and reduced rather than increased aortic root diameter (Mitchell et al. 2003). However, the only prospective analysis testing this hypothesis, performed in 3195 Framingham study participants with normal BP values at baseline, failed to show an association between incidence of hypertension and aortic root size (Ingelsson et al. 2008). The hypothesis that the association between aortic root size and BP may be related to specific subtypes of hypertension (isolated diastolic, isolated systolic, or systolic–diastolic hypertension) was not confirmed in a cross-sectional evaluation of 1256 Taiwanese patients (Farasat et al. 2008). In this study, after accounting for age and body surface area, ARD does not differ between normotensive and hypertensive individuals, even when the various patterns of hypertension are examined separately (Farasat et al. 2008), highlighting the importance of correctly matching cases and controls for those parameters which are known to be the main determinants of aortic size, namely sex, age and body size. Kim et al. (Kim et al. 1996) evaluated the size of ascending aorta in 110 normotensive individuals and 110 hypertensive patients matched for age and sex: after indexing aortic size for BSA, no significant difference was found between the two groups at the aortic annulus or at the SoV. In a study involving 102 patients with severe aortic regurgitation, Roman et al. found similar mean M-mode aortic diameters in normotensive and hypertensive groups (Roman et al. 1987).
Tell et al. in the Cardiovascular Health Study found a relation between diastolic but not systolic pressure and M-mode echocardiographic dimensions when the entire elderly cohort was analysed; however, when the “healthier” subgroup (no coronary heart disease or antihypertensive therapy) was examined, aortic diameter was not associated with blood pressure (Tell et al. 1994).
In a cross-sectional analysis of the 2096 hypertensive and 361 normotensive participants in the The Hypertension Genetic Epidemiology Network (HyperGEN) study (Palmieri et al. 2001), the prevalence of aortic root dilatation was similar between hypertensive and normotensive individuals and only a weak direct relation between ARD and office DBP was observed. In the Losartan Intervention for Endpoint reduction (LIFE) study (Bella et al. 2002) examining 947 hypertensive patients with electrocardiographic LVH no significant relationship between BP values and aortic dilatation was found. Other imaging evaluations (Vriz et al. 2013; Campens et al. 2014; Bella et al. 2002; Agmon et al. 2003; Mitchell et al. 2008; Savage et al. 1979) and autopsy series (Sawabe et al. 2011; Virmani et al. 1991) examined this relation with mixed results. Nonetheless, recent data suggest a high prevalence of echocardiographic aortic root dilatation in the hypertensive population (Cipolli et al. 2009; Cuspidi et al. 2011; Covella et al. 2014).
In order to assess the prevalence of ARD dilatation in arterial hypertension, Covella and co-workers performed a meta-analysis including eight studies and a total of 10,791 hypertensive patients (Covella et al. 2014). Prevalence of ARD in the pooled population was 9.1 % with a marked difference between men and women (12.7 vs. 4.5 %). Hypertensive patients with ARD enlargement and those with normal aortic root size had similar office BP values (Covella et al. 2014).
On the contrary, in the PAMELA (Pressioni Arteriose Monitorate E Loro Associazioni) study (Cuspidi et al. 2014), conducted in 2051 individuals randomly selected from the population of Monza (Italy), and with a low global cardiovascular risk profile, office, home and 24-h ambulatory SBP and DBP showed a direct, significant correlation with ARD (Cuspidi et al. 2014).
Additionally, the Healthy Coronary Artery Risk Development in Young Adults (CARDIA) study provides longitudinal data on clinical correlates of aortic root size and dilatation through a 20-year period of early adulthood, in 3051 young adults aged 23–35 years (Teixido-Tura et al. 2015). Aortic root diameter measured by M-mode echocardiography at the end of the period of observation was positively correlated with the 20-year increase in diastolic, systolic, and mean arterial pressure and inversely related to pulse pressure (Teixido-Tura et al. 2015).
Very recently, in a large group of hypertensive individuals characterized by a high prevalence of chronic kidney disease (CKD), we found only a weak association, close to statistical significance (p = 0.08), between clinic diastolic BP and ARD either not indexed or normalized for height (Mulé et al. 2016).
Several reasons could explain the inconsistent findings of the studies above described: differences in study design, failure of cross-sectional studies to match hypertensive patients and normotensive individuals for all the potential confounding factors, definition of the aortic phenotype, and site and types of BP measurement. Whereas in the majority of studies only clinical BP measurements were available, out-of-office BP and central hemodynamics might be closer correlates of ARD enlargement (Kim et al. 1996; Cipolli et al. 2009; Cuspidi et al. 2014; Cuspidi et al. 2007).
Besides the aforementioned PAMELA study, that is the first one linking elevated out-of-office BP, as assessed either by home and ambulatory measurements, to aortic dilatation (Cuspidi et al. 2014), in a previous report of the same research group, average night-time diastolic BP, but not clinic or average 48 h BP, showed an independent association with ARD in 519 never-treated hypertensive patients (Cuspidi et al. 2007).
However, in the previously cited study of Kim et al. 24 h ambulatory BP readings were less strongly correlated to aortic diameters than casual blood pressure in the normotensive group, whereas ambulatory blood pressure was more correlated to aortic size in the hypertensive group (Kim et al. 1996). The physiological significance of this difference remains unclear.
A more accurate measurement of hemodynamic load may be achieved by non-invasive estimation of central arterial pressure and vascular compliance or by quantitative analysis of the arterial pressure waveform. Despite similar mean pressures, systolic and diastolic pressures may vary considerably with the degree of wave amplification, being maximal in the young, normotensive individual with a compliant vasculature (Erbel et al. 2014; Vasan et al. 1995).
Indeed, Milan and co-workers documented that, in a total of 190 untreated and treated essential hypertensive patients, central hemodynamic variables (aortic augmentation index and central pulse pressure) estimated by an applanation tonometry applied to the radial artery, were significantly associated with an increased ARD, whereas peripheral BP was not (Milan et al. 2011).
Moreover, assuming a relation between BP and aortic root diameter exists, the impact of the duration of high BP must be taken into account, as seem to suggest the relatively low prevalence of aortic root dilatation (3.7 %) among the untreated newly diagnosed hypertensive subjects examined by Cuspidi et al. (Cuspidi et al. 2007).
It is also possible that antihypertensive medications, taken by most of the patients enrolled in the investigations above reported, may have concealed the relation between BP and aortic root size. Last, but not least, the use of different methods for indexation of ARD may have in part contributed to the heterogeneous findings obtained in the investigations exploring the relationships between ARD and blood pressures.
Indeed, the best method for ARD indexation, as well as the normal values of aortic root size, are still a matter of debate (Table 1) (Erbel et al. 2014; Baguet et al. 2012; Pape et al. 2007; Davies et al. 2006; Lang et al. 2015; Vriz et al. 2013; Campens et al. 2014; Vasan et al. 1995; Palmieri et al. 2001; Bella et al. 2002; Cuspidi et al. 2007, 2011, 2014; Milan et al. 2011; Devereux et al. 2012). In the past years absolute measures were used to assess the size of aortic root, but the absolute aortic diameter is not a good enough marker of risk for aortic dissection (Erbel et al. 2014; Baguet et al. 2012; Pape et al. 2007; Davies et al. 2006; Lang et al. 2015). It is noteworthy that, as above reported, the majority of patients with acute type A aortic dissection had an aortic diameter less than 5.5 cm, a measure that is considered the cut-off value for suggesting aortic replacement by surgical guidelines (Pape et al. 2007).
Table 1
Cut-off values of aortic root size, measured by echocardiography at the sinuses of Valsalva, as suggested by different authors
Author, year | Not indexed (cm) | Indexed for BSA (cm/m2) | Indexed for height (cm/m) |
---|---|---|---|
Roman et al., 1989 | M: 4.0; F: 3.6 | M: 2.1; F: 2.1 | – |
Vasan et al., 1995 (Framingham study) | – | 95th percentiles that can be calculated by sex-specific regression equations | 95th percentiles that can be calculated by sex-specific regression equations |
Bella et al., 2002 (LIFE study) | – | >2SD above the regression line with BSA in a reference population | – |
Palmieri et al., 2005 (HYPERGEN study) | – | 97.5th percentiles that can be calculated by sex-specific regression equations | – |
Cuspidi et al., 2006 (ETODH registry) | M: 4.0; F: 3.8 | – | – |
Cuspidi et al., 2007 | M: 4.0; F: 3.7 | – | – |
Cipolli et al., 2009 | M: 4.0; F: 3.7 | – | – |
Milan et al., 2011 | – | 2.0 | – |
Cuspidi et al., 2011 | M: 3.9; F: 3.7 | – | – |
Baguet et al., 2012 (ESH newletter) | M: 2.1; F: 2.1 | ||
Devereux et al., 2012 | – | Nomograms based on age, sex and BSA | Nomograms based on age, sex and height |
Milan et al., 2013 | M: 3.9; F: 3.7 | – | – |
Cuspidi et al., 2014 (PAMELA study) | M: 3.8; F: 3.4 | M: 2.1; F: 2.2 | M: 2.3; F: 2.2 |
Campens et al., 2014 | – | Gender-, age- and BSA- specific upper limits of normal and Z-score equations | – |
For the great influence of BSA on aortic root dimensions, it has been proposed to normalize the absolute values of ARD for BSA (ARD/BSA) (Erbel et al. 2014; Baguet et al. 2012; Lang et al. 2015; Campens et al. 2014; Devereux et al. 2012; Hiratzka et al. 2010), considering also that such an indexation predicted the risk of rupture, dissection and death in patients with thoracic aortic aneurysms, better than did unadjusted aortic root size (Erbel et al. 2014; Davies et al. 2006). Furthermore, ARD/BSA showed a significant association with mortality (Lai et al. 2010).
On the other hand, some authors have suggested that the use of body surface area as a means of adjustment for differences in body size is mathematically incorrect (Nidorf et al. 1992) and carries the potential risk of an under diagnosis in obese subjects, which are a substantial proportion of hypertensive patients, and of an over diagnosis in the individuals with lower BSA (e.g. women).
For these reasons, they advocate indexation of aortic diameter for height that is considered a more linear and mathematically sound way of comparing measurements. (Nidorf et al. 1992). Finally, some evidence suggests that ARD would have a better prognostic value when normalized to height (Cuspidi et al. 2014).
Regardless of the method used to measure BP, overall the contribution of it to aortic root dilatation has been shown to be substantially inferior than that of some non-hemodynamic factors.
4 Non-hemodynamic Determinants of Aortic Root Size
Besides BP, several factors influence aortic root dimension, including age, gender and anthropometric variables, such as height, weight and their derivatives body surface area and body mass index (BMI) (Erbel et al. 2014; Kim et al. 1996; Bella et al. 2002; Teixido-Tura et al. 2015; Devereux et al. 2012; Hiratzka et al. 2010; Lai et al. 2010; Nidorf et al. 1992; Reed et al. 1993; Roman et al. 1989). Of these factors, anthropometric variables have the greatest impact.
In some studies, height is the most important determinant of aortic root size compared with other anthropometric indexes, such as weight or body surface area (Lai et al. 2010; Nidorf et al. 1992; Reed et al. 1993). Original observations in Framingham study (Vasan et al. 1995) showed that height is the most important predictor, with every 10-cm increment in height associated with increment in aortic root size of 0.24 mm in men and 0.38 mm in women.
Weight also influences aortic dimensions with a 10-kg increase in weight associated with an increment in aortic root size of 0.87 in males and 0.68 mm in females (Vasan et al. 1995).
More recently, Campens et al. in a cohort of 849 children and adults (Campens et al. 2014) demonstrated that ARD measured by TTE at the sinuses of Valsalva correlated closely with height in the children, whereas in subjects > 15 years of age, height was less strongly correlated with aortic dimensions compared to BSA.
An association between age and aortic root size has been consistently reported in autopsy series and clinical studies (Milan et al. 2011, 2013; Kim et al. 1996; [12]; Vriz et al. 2013; Campens et al. 2014; Vasan et al. 1995; Lam et al. 2010; Agmon et al. 2003; Savage et al. 1979; Biaggi et al. 2009; Sawabe et al. 2011; Virmani et al. 1991; Cipolli et al. 2009; Cuspidi et al. 2007, 2011, 2014; Covella et al. 2014; Teixido-Tura et al. 2015; Mulé et al. 2016; Devereux et al. 2012; Hiratzka et al. 2010). In the most recent Framingham analysis (Lam et al. 2010) is reported a 0.9-mm increase of aortic dimension in men and 0.7 mm in women, for each successive decade of life, after adjustment for other determinants. The age-related increase of ARD is considered a consequence of fatigue and fracture of elastin fibers with subsequent remodelling (O’Rourke and Nichols 2005). This view applies the principles of material fatigue that relate fracture of non-living components with extent of pulsatile strain and the number of applied cycles of strain (i.e., heart beats). This theory explains both aortic dilation (from fracture of elastic components) and stiffening (from transfer of tension from elastin to collagenous fibers in the wall) (O’Rourke and Nichols 2005).
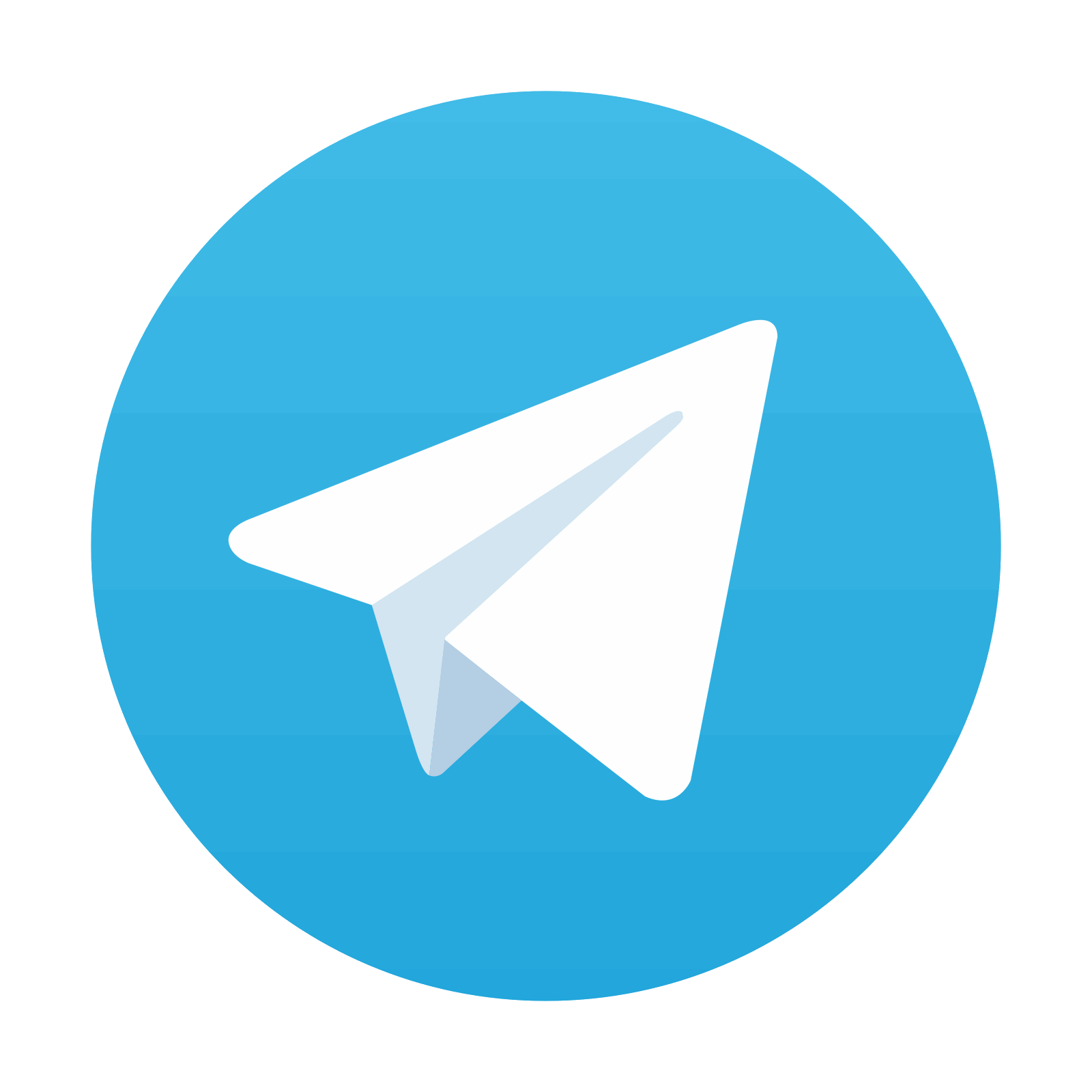
Stay updated, free articles. Join our Telegram channel

Full access? Get Clinical Tree
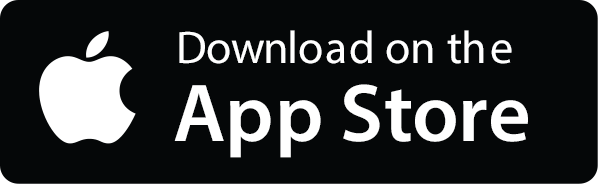
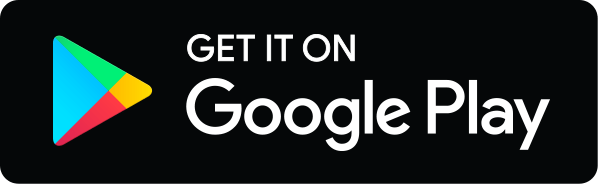