Natriuretic peptides have important roles in the regulation of vasomotor tone, salt homeostasis, and ventricular remodeling. Lower natriuretic peptide levels observed in obese individuals may underlie the greater cardiovascular risk associated with obesity. Thus the aim of this study was to determine whether lower natriuretic peptide levels in obesity are attributable to differences in regional fat distribution. We investigated the relation of plasma N-terminal pro–B-type natriuretic peptide (NT–pro-BNP) to regional adiposity in 1,873 community-based individuals (46% women, mean age 45 years). Subcutaneous adipose tissue (SAT) and visceral adipose tissue (VAT) volumes were measured by multidetector computed tomography. In gender-specific multivariable analyses adjusting for age and blood pressure, log NT–pro-BNP was inversely associated with VAT in men (beta −0.11 per standard deviation increment, p <0.001) and women (beta −0.19, p <0.001). Log NT–pro-BNP was inversely associated with SAT in women only (beta −0.14, p <0.001). In models containing VAT and SAT, only VAT was significantly associated with log NT–pro-BNP (men, beta −0.137, p <0.001; women, beta −0.184, p <0.001). VAT remained associated with log NT–pro-BNP even after adjustment for body mass index and waist circumference (beta −0.119, p <0.001) and in analyses restricted to nonobese patients (beta −0.165, p <0.001). Adjustment for insulin resistance attenuated the associations of NT–pro-BNP with VAT and SAT. In conclusion, this study demonstrates that circulating NT–pro-BNP is related to variations in regional and particularly visceral adiposity. These findings suggest that excess visceral adiposity and concomitant hyperinsulinemia may contribute to the natriuretic peptide “deficiency” observed in obesity.
It is uncertain whether the link between obesity and natriuretic peptides is attributable to differences in fat mass because most studies have used anthropometric measurements to characterize obesity. One previous study has noted that B-type natriuretic peptide (BNP) levels are inversely correlated with lean body mass as measured by dual energy x-ray absorptiometry. Other studies have proposed that factors such as increased renal clearance of BNP or confounding by clinical characteristics may account for the low natriuretic peptide levels in obesity. Furthermore, it is unknown whether differences in regional fat distribution are related to circulating natriuretic peptide levels. The 2 major fat compartments are subcutaneous and visceral, with the latter recognized as the more metabolically active and, hence, pathogenic fat depot. Thus we investigated cross-sectional relations between plasma N-terminal pro-BNP (NT–pro-BNP) and visceral and subcutaneous adiposities in a large community-based sample. Regional adiposity was assessed using multidetector computed tomography, which can reliably characterize subcutaneous adipose tissue (SAT) and visceral adipose tissue (VAT) volumes.
Methods
Beginning in 2002 third-generation study participants (n = 4,095) of the Framingham Heart Study who had ≥1 parent in the Framingham Offspring Study were enrolled and underwent standard clinic examinations. A subset of 2,111 third-generation participants also underwent multidetector computed tomographic scanning from 2002 through 2005 at their first examination. Inclusion in this subset was weighted toward Framingham Heart Study participants who resided in the greater New England area. Participants were eligible if they weighed <159 kg (350 lb) and were men ≥35 years of age or women ≥40 years of age and not pregnant. Of third-generation participants who underwent computed tomographic scanning 1,873 (89%) had abdominal SAT and VAT volumes measured, were free of diabetes, had no history of cardiovascular disease or atrial fibrillation, had a serum creatinine level ≤106 μmol/L (1.2 mg/dl), and had NT–pro-BNP measured. This sample was used in analyses relating NT–pro-BNP to measurements of regional adiposity for this study.
All study participants underwent routine physical examination with medical history and laboratory assessment of cardiovascular disease risk factors. Body mass index (BMI) was calculated as weight in kilograms divided by height in meters squared. Hypertension was defined as systolic blood pressure ≥140 mm Hg, diastolic blood pressure ≥90 mm Hg, or use of antihypertensive treatment. Renal function was estimated using the estimated glomerular filtration rate. Metabolic syndrome was defined according to 2005 American Heart Association criteria as the presence of ≥3 of the following clinical risk factors: waist circumference ≥40 inches in men or ≥35 inches in women, serum triglycerides ≥150 mg/dl, high-density lipoprotein cholesterol <40 mg/dl in men or <50 mg/dl in women or taking medication for low high-density lipoprotein cholesterol, systolic blood pressure ≥130 mm Hg or diastolic blood pressure ≥85 mm Hg or taking antihypertensive medication, and fasting glucose level ≥100 mg/dl. Alcohol use was defined as >14 drinks/week for men or >7 drinks/week for women. Institutional review boards of the Boston University School of Medicine and Massachusetts General Hospital approved this study protocol. All participants provided written informed consent.
Plasma samples were obtained in the morning after an overnight fast and frozen at −70°C. Plasma NT–pro-BNP was determined using a commercially available immunoassay (Elecsys proBNP, Roche Diagnostics, Indianapolis, Indiana) on an Elecsys 1010 analyzer according to established methods. Mean interassay coefficient of variation was 2.7%. Serum insulin was measured by radioimmunoassay (human insulin enzyme-linked immunosorbent assay kit, Linco Research, St. Charles, Missouri) that had minimal cross reactivity with other insulin-related molecules and an intra-assay coefficient of variation of 2.7%. Homeostasis model assessment of insulin resistance (HOMA-IR) was estimated using fasting insulin and glucose values, where HOMA-IR = (fasting insulin [microunits per milliliter] × fasting blood glucose [millimoles per liter])/22.5. Patients with HOMA-IR in the top quartile were considered to have insulin resistance. Free testosterone was calculated using the Law of Mass Action equation and using measurements of serum total testosterone concentrations quantified by liquid chromatographic/tandem mass spectrometric assay.
Imaging was performed with an 8-slice multidetector computed tomographic scanner (Lightspeed Ultra, General Electric, Milwaukee, Wisconsin) using a standard protocol that has been reported previously. Briefly, 25 contiguous 5-mm-thick slices were acquired above the level of S1. The abdominal muscular wall separating visceral from subcutaneous fat compartments was manually traced on acquired images and volumetric assessments of SAT and VAT were made. Intra- and inter-reader reproducibilities were high with interclass correlations of 0.997 for SAT and 0.992 for VAT.
The regression dependent variable, NT–pro-BNP, was logarithmically transformed because of its skewed distribution. Correlations among BMI, SAT, and VAT were assessed in the study sample using unadjusted Spearman correlation analyses.
Multivariable linear regression was performed in the total sample to examine age- and gender-adjusted associations of NT–pro-BNP (dependent variable) with adiposity measurements (SAT and VAT). In multivariable models further adjusting for systolic blood pressure and antihypertensive treatment, which are clinical variables known to be associated with NT–pro-BNP, we used multiplicative interaction terms to test for effect modification by gender or age on the relation of adiposity measurements to plasma NT–pro-BNP. Gender-specific analyses were performed in instances when a positive gender interaction was noted. Further adjustment for BMI and waist circumference was included in analyses of VAT but not in analyses of SAT. Adjustment for BMI or waist circumference in analyses of SAT is generally avoided because of known colinearity between anthropometric measurements (BMI and waist circumference) and subcutaneous adiposity.
In secondary analyses we assessed the relation of plasma NT–pro-BNP to adiposity measurements and accounted for estimated glomerular filtration rate, metabolic traits that included metabolic syndrome and HOMA-IR ≥75th percentile, and circulating androgens (total testosterone and free testosterone). In multivariable models we also included additional covariates known to correlate with computed tomographic measurements of adiposity: hormone replacement therapy (in women) and alcohol use. We repeated all analyses using generalized estimating equations linear regression to account for correlations in related patients (siblings) in the study sample (SAS PROC GENMOD).
All analyses were performed using SAS 9.1.3 (SAS Institute, Cary, North Carolina), and a 2-tailed p value <0.05 was considered statistically significant.
Results
Characteristics of the study sample are listed in Table 1 . Mean age was 45 years and 46% of participants were women. Overall, 25% of participants were obese (BMI ≥30 kg/m 2 ) and 39% were overweight (BMI ≥25 to <30 kg/m 2 ). Spearman correlation coefficients for relations of BMI to SAT and VAT were 0.81 and 0.77, respectively. Correlation coefficients between SAT and VAT were 0.60 in men and 0.78 in women.
Variable | Entire Sample (n = 1,873) | Men (n = 1,007) | Women (n = 866) |
---|---|---|---|
Clinical and anthropometric measurements | |||
Age (years) | 44.8 ± 6.1 | 43.7 ± 6.2 | 46.1 ± 5.6 |
Body mass index (kg/m 2 ) | 27.3 ± 5.2 | 28.0 ± 4.4 | 26.4 ± 5.8 |
Waist circumference (inches) | 37.3 ± 5.5 | 39.0 ± 4.6 | 35.4 ± 5.9 |
Systolic blood pressure (mm Hg) | 119 ± 14 | 121 ± 13 | 116 ± 16 |
Diastolic blood pressure (mm Hg) | 77 ± 9 | 79 ± 9 | 74 ± 9 |
Hypertension | 20% | 23% | 16% |
Smoker | 14% | 15% | 14% |
Postmenopausal ⁎ | 9% | — | 18% |
Hormone replacement therapy ⁎ | 5% | — | 10% |
Alcohol use † | 15% | 16% | 14% |
Metabolic syndrome | 24% | 29% | 17% |
Biochemical measurements | |||
N-terminal pro–B-type natriuretic peptide (pg/ml) ‡ | 27.7 (13.3–52.5) | 16.6 (8.4–30.3) | 46.5 (27.3–78.0) |
Homeostasis model assessment of insulin resistance ‡ | 1.0 (0.7–1.5) | 1.1 (0.8–1.7) | 0.9 (0.7–1.3) |
Computed tomographic measurements | |||
Subcutaneous adipose tissue (cm 3 ) | 2,761 ± 1,404 | 2,571 ± 1,240 | 2,982 ± 1,545 |
Visceral adipose tissue (cm 3 ) | 1,565 ± 898 | 1,958 ± 864 | 1,109 ± 699 |
⁎ Data are from women only (n = 866).
† Defined as >14 drinks/week for men or >7 drinks/week for women.
‡ Median (interquartile range) presented for continuous variables that are not normally distributed.
In age- and gender-adjusted analyses log NT–pro-BNP was inversely associated with SAT (beta −0.074 change in log NT–pro-BNP per SD increment in SAT, p <0.001) and VAT (beta −0.124, p <0.001). In multivariable analyses adjusting for age, gender, systolic blood pressure, and antihypertensive treatment there was no significant interaction of age with VAT or SAT (p >0.10). However, there was evidence of a gender interaction for the association of log NT–pro-BNP with SAT (p = 0.02) but not with VAT (p = 0.10). Therefore, analyses of SAT were performed in men and women separately ( Table 2 ). In these gender-specific analyses the multivariable-adjusted association of higher SAT with lower log NT–pro-BNP was significant in women but not in men ( Table 2 ); a 1-SD increment in SAT was associated with a 13% lower NT–pro-BNP level in women.
Independent Variables | Model Including SAT | Model Including VAT | Model Including SAT and VAT | |||
---|---|---|---|---|---|---|
Beta ± SE ⁎ | p Value | Beta ± SE | p Value | Beta ± SE | p Value | |
Total Sample: adjusting for age, sex, SBP, and anti-hypertensive treatment | ||||||
Subcutaneous adipose tissue | NA † | NA † | ||||
Visceral adipose tissue | −0.143 ± 0.021 | <0.001 | −0.152 ± 0.028 | <0.001 | ||
Men Only: adjusting for age, SBP, and anti-hypertensive treatment | ||||||
Subcutaneous adipose tissue | −0.032 ± 0.028 | 0.25 | 0.043 ± 0.034 | 0.20 | ||
Visceral adipose tissue | −0.111 ± 0.029 | <0.001 | −0.137 ± 0.036 | <0.001 | ||
Women Only: adjusting for age, SBP, and anti-hypertensive treatment | ||||||
Subcutaneous adipose tissue | −0.141 ± 0.029 | <0.001 | −0.009 ± 0.043 | 0.83 | ||
Visceral adipose tissue | −0.191 ± 0.030 | <0.001 | −0.184 ± 0.044 | <0.001 |
⁎ Values represent change in log N-terminal pro–B-type natriuretic peptide (dependent variable) per SD increase in value of independent continuous variables shown. For example, a 1 SD increase in visceral adipose tissue was associated with an e −0.143 = 0.87 change in N-terminal pro–B-type natriuretic peptide in the total sample.
† Only gender-specific analyses were performed for subcutaneous adipose tissue because of the presence of a significance gender interaction with subcutaneous adipose tissue on variation in log N-terminal pro–B-type natriuretic peptide.
In multivariable analyses log NT–pro-BNP was inversely associated with VAT in the total sample; thus a 1-SD increment in VAT was associated with a 13% lower NT–pro-BNP level in men and women. In gender-specific multivariable analyses higher VAT was significantly associated with lower NT–pro-BNP in men and women ( Table 2 ). Further, in models containing SAT and VAT, NT–pro-BNP was significantly associated with VAT but not with SAT ( Table 2 ).
In analyses adjusting for BMI or waist circumference VAT remained significantly associated with NT–pro-BNP ( Table 3 ). The multivariable-adjusted relation of lower NT–pro-BNP with higher VAT, but not with SAT, remained significant in models that included metabolic syndrome ( Table 3 ). However, when adjusting instead for HOMA-IR, the inverse association between BNP and VAT was attenuated ( Table 3 ).
Independent Variables | Model Including SAT | Model Including VAT | ||
---|---|---|---|---|
Beta ± SE ⁎ | p Value | Beta ± SE | p Value | |
Adjusting for age, gender, systolic blood pressure, antihypertensive treatment, body mass index | ||||
Subcutaneous adipose tissue † | N/A | N/A | ||
Visceral adipose tissue | −0.105 ± 0.031 | 0.0008 | ||
Adjusting for age, gender, systolic blood pressure, antihypertensive treatment, waist circumference | ||||
Subcutaneous adipose tissue | N/A | N/A | ||
Visceral adipose tissue | −0.130 ± 0.033 | <0.0001 | ||
Adjusting for age, gender, systolic blood pressure, antihypertensive treatment, metabolic syndrome | ||||
Subcutaneous adipose tissue | −0.036 ± 0.023 | 0.11 | N/A | |
Adjusting for age, gender, systolic blood pressure, antihypertensive treatment, body mass index, metabolic syndrome | ||||
Visceral adipose tissue | −0.073 ± 0.033 | 0.028 | ||
Adjusting for age, gender, systolic blood pressure, antihypertensive treatment, homeostasis model assessment of insulin resistance | ||||
Subcutaneous adipose tissue | −0.012 ± 0.023 | 0.59 | N/A | |
Adjusting for age, gender, systolic blood pressure, antihypertensive treatment, body mass index, homeostasis model assessment of insulin resistance | ||||
Visceral adipose tissue | −0.057 ± 0.033 | 0.081 |
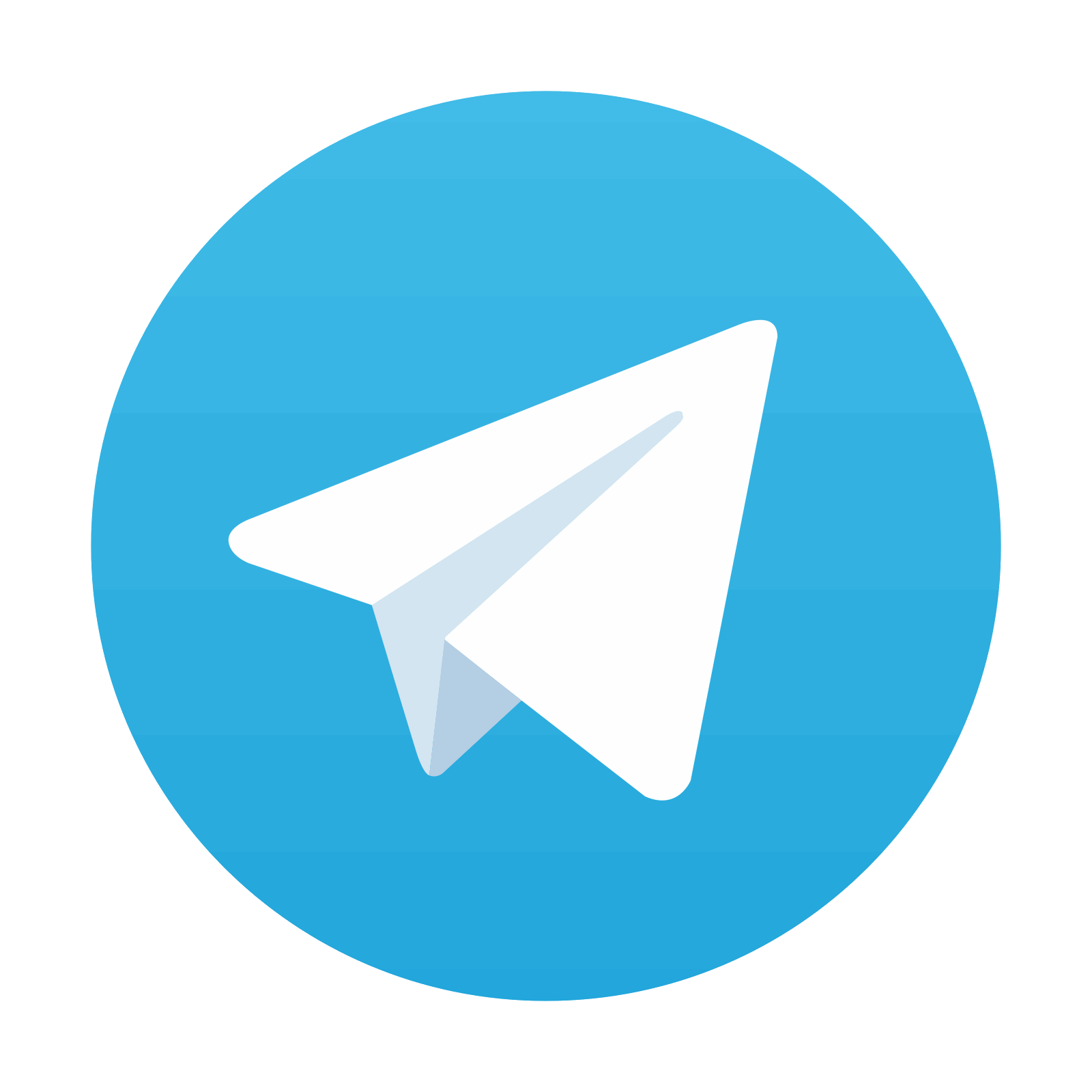
Stay updated, free articles. Join our Telegram channel

Full access? Get Clinical Tree
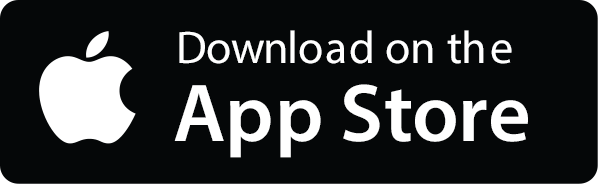
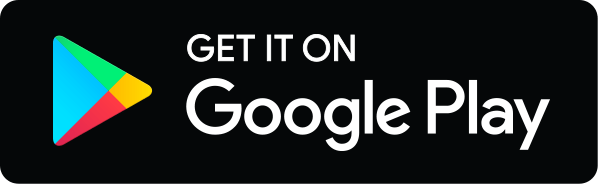
