Background
Previous studies have shown that delayed hyperenhancement (HE) by cardiac magnetic resonance (CMR) is related to left ventricular (LV) stiffness in a variety of cardiac diseases. However, it is not known whether this relation is also present in dilated cardiomyopathy (DCM), in which replacement fibrosis is less extensive.
Methods
Consecutive patients with DCM (n = 50, age 56 ± 13 years, 20 females) were studied with CMR and two-dimensional and Doppler echocardiography. CMR images with breath-hold segmented inversion-recovery sequence were acquired 10 minutes after an intravenous bolus of gadolinium. The scar tissue distribution and extent were calculated in the 17 LV segments. LV diastolic function was assessed using mitral inflow and tissue Doppler velocities at the septal and lateral sides of the mitral annulus.
Results
The group had an ejection fraction of 30% ± 11% and left atrial volume of 103 ± 50 mL by CMR. HE was present in 31 patients with a mean scar burden of 4% ± 7.6%. Patients with DCM without HE had a significantly higher septal E/e’ ratio than patients with scar ( P = .05), and there was no significant correlation between deceleration time and extent of HE. Lateral late diastolic velocity (a’) had a weak inverse relation with the number of segments with HE ( r = −0.44, P = .005) and with scar burden ( r = −0.31, P = .05).
Conclusion
Patients with DCM without HE have higher LV filling pressures than patients with HE. Our findings suggest that other factors influence LV stiffness to a larger extent than replacement fibrosis in this population.
Diastolic dysfunction is an important determinant of dyspnea in patients with dilated cardiomyopathy (DCM) and relates better with exercise tolerance and clinical events in this population than left ventricular (LV) ejection fraction (EF).
There are several mechanisms that account for diastolic dysfunction in DCM. These include impaired LV relaxation due to intrinsic myocyte abnormalities and increased wall stiffness related to changes in sarcomeric proteins and the interstitial matrix. Cardiac magnetic resonance (CMR) has been successfully applied to evaluate replacement fibrosis using delayed enhancement-CMR (DE-CMR). More recently, a significant association was reported between replacement fibrosis by DE-CMR and LV stiffness by Doppler echocardiography in patients with various grades of diastolic dysfunction and encompassing a wide spectrum of cardiac pathology. However, it is unknown if the contribution of replacement fibrosis is similar across different diseases, particularly in patients with DCM in whom replacement fibrosis is usually less extensive than in patients with post-infarction cardiomyopathy. We therefore undertook this study to evaluate the relation between the extent and distribution of scar by DE-CMR and Doppler indices of LV diastolic function in patients with DCM.
Materials and Methods
The study population was composed of consecutive patients referred for CMR who also underwent two-dimensional and Doppler echocardiography. There were 50 patients (20 female) who were diagnosed with idiopathic DCM, using clinical criteria and based on the exclusion of other causes of LV dysfunction, such as acute myocarditis (dynamic state with potential for further injury or recovery), coronary artery disease, and severe valvular disease (stenosis or regurgitation). Patients with a prosthetic mitral valve were excluded because prosthetic mitral valves pose a limitation to the assessment of diastolic function by Doppler echocardiography. Patients were imaged in a stable hemodynamic state and were able to lie supine during the imaging sessions. Clinical status as judged by heart rate and blood pressure was similar during CMR and Doppler echocardiography.
CMR Acquisition and Analysis
All images were acquired on a clinical 1.5-Tesla magnetic resonance scanner (MAGNETOM Avanto or Sonata, Siemens Medical Solutions, Inc., Erlangen, Germany) using a phased-array receiver coil during repeated breath-holds as described previously. Briefly, cine-CMR images were acquired in multiple short-axis and long-axis views using an electrocardiogram-gated steady-state free precession sequence. Short-axis views were obtained every 1 cm from the atrioventricular ring to the apex to cover the entire LV (slice thickness, 6 mm; interslice gap, 4 mm), approximately 10 minutes after administration of an intravenous gadolinium-based contrast agent. Most patients received either gadoversetamide or gadopentetate dimeglumine at a dose of 0.15 mmol/kg, whereas few patients received gadobenate dimeglumine at a dose of 0.1 mmol/kg. DE-CMR images were acquired, in the same views as cine-CMR, using a T1-weighted segmented inversion-recovery sequence with inversion time continuously adjusted to null normal myocardium, typical in plane resolution was 2.0 × 1.5 cm with a slice thickness of 6.0 to 7.0 mm. No patients were excluded on the basis of CMR image quality.
Magnetic resonance examinations were analyzed by an experienced CMR physician blinded to the patient echocardiographic data. LV volumes (normal range 35–75 mL/m 2 for end-diastolic volume and 12–30 mL/m 2 for end-systolic volume), LV mass, and EF were measured by planimetry of contiguous short-axis cine images using endocardial and epicardial contours at end systole and end diastole. Left atrial volumes were measured using the biplane area-length method.
The presence and location of tissue with hyperenhancement (HE), which is assumed to represent replacement fibrosis, was determined by visual inspection using the American Heart Association 17-segment model without knowledge of the Doppler results. Regional replacement fibrosis was scored according to the spatial extent of HE within each segment (0 = no HE; 1 = 1%–25% HE; 2 = 26%–50% HE; 3 = 51%–75% HE; and 4 = 76%–100% HE). This qualitative method was chosen because it has been used in prior studies of patients with DCM, and threshold methods are difficult to apply because of the patchy nature of HE in DCM. The basal and mid-segments were each divided into anterior, anteroseptal, anterolateral, inferior, inferoseptal, and inferolateral walls. The apical segments were divided into anterior, septal, inferior, lateral, and true apex. The number of segments with HE was noted. The total scar burden was expressed as a percentage of LV myocardial volume and derived by averaging the score for all 17 segments.
Echocardiographic Acquisition and Analysis
The patients studied in the echocardiography laboratory were imaged in the left lateral decubitus position with an ultrasound system equipped with a multifrequency transducer. A complete echocardiographic study was performed per standard views and guidelines. From the apical window, pulsed-wave Doppler was used to record mitral inflow for three to five cardiac cycles at the level of the mitral valve annulus (to quantify mitral inflow and mitral regurgitation) and at the tips level for diastolic function assessment. Tissue Doppler (TD) was applied to record mitral annular velocities at the septal and lateral sides of the annulus. The resulting annular velocities by pulsed-wave Doppler were recorded for five cardiac cycles at a sweep speed of 100 mm/s. Echocardiographic measurements were performed by an observer on a computerized off-line analysis station (Digisonics EC, Houston, TX) without knowledge of cardiac MR findings.
Mitral inflow from tips level was analyzed for peak early (E), and late (A) diastolic velocities, E/A ratio, and deceleration time of mitral E velocity. Mitral annulus early (e’) and late (a’) diastolic velocities were measured at septal and lateral mitral annulus, and e’/a’ and E/e’ ratios were computed. Measurements were averaged over three cardiac cycles. Of note, mitral inflow velocities and time intervals relate well with invasive measurements of LV stiffness and filling pressures in patients with DCM and were recommended along with the TD velocities in the recent American Society of Echocardiography/European Association of Echocardiography guidelines for clinical application in this group of patients. Patients with increased stiffness usually have an increase in LV filling pressures. Because mitral E/A and E/e’ ratios are higher in patients with increased LV filling pressures, both ratios are also of potential value in drawing conclusions about LV stiffness. In addition, some studies have reported significant correlations between both ratios and LV stiffness. For mitral E/A ratio, the significant correlation was noted in patients with depressed EF. For the E/e’ ratio, the correlation was noted in patients with normal EF.
Statistics
Data are presented as mean and SD or median (25th–75th percentile) for continuous variables and numbers (percent) for categoric variables. Comparisons between mitral annulus velocities and patients with and without late gadolinium enhancement were performed using t tests or Mann–Whitney rank-sum test based on whether the velocities and ratios had a normal distribution or not. Regression analysis was applied to evaluate the relation between scar burden/number of segments with scar by CMR and LV volumes and EF, as well as Doppler measurements. The sample size was selected such that the study would have > 80% power to detect a correlation coefficient of 0.44 between the E/e’ ratio and scar burden by DE-CMR (n = 50 with an alpha level of 0.05). This correlation coefficient was based on previous studies that evaluated the relation between HE and E/e’ ratio. A P value < .05 was used to define statistical significance for the different comparisons.
Results
The 50 patients had a mean age of 56 ± 13 years and a BSA of 1.93 ± 0.25 m 2 . LV dilatation (end-diastolic volume: 219 ± 79 mL; end-systolic volume: 159 ± 76 mL) was present with a moderately severe depressed EF (30% ± 11%). LV mass was 203 ± 66 g. The left atrium was dilated with a maximum volume of 103 ± 50 mL. None of the patients had more than mild mitral regurgitation. The median difference between the CMR and echocardiography studies was 1 day (25th percentile: 0, 75th percentile: 6 days).
DE-CMR Findings
HE was noted in 31 of 50 patients (62%). The average scar burden was 4% ± 7.6% of LV myocardial volume. A total of 150 segments (150/850 or 17.6%) had HE. HE was noted mostly in a mid mural and patchy pattern. There were no cases of transmural HE. HE was most frequently noted in the septal segments (71/150 or 47%) but was also present in 28 lateral wall segments (anterolateral and inferolateral grouped together), 19 inferior segments, 23 apical segments, and 9 anterior segments. On average, HE was present in 3 ± 4 segments per patient.
Echocardiographic Doppler Findings
Table 1 summarizes Doppler velocity measurements. Satisfactory pulmonary venous flow signals could be recorded in only 10 patients and therefore were not used for further analysis. Septal e’ velocity was significantly lower than lateral e’ velocity ( P < .001), and septal E/e’ ratio was significantly higher than lateral E/e’ ratio ( P = .006) in the whole group of 50 patients. There was no significant difference between septal and lateral a’ velocity, but septal e’/a’ ratio showed a trend to lower values in comparison with lateral e’/a’ ratio ( P = .06).
Heart rate (/min) | 80 ± 16 |
Systolic blood pressure (mm Hg) | 124 ± 18 |
Diastolic blood pressure (mm Hg) | 77 ± 13 |
Peak mitral E velocity (cm/s) | 84 ± 31 |
Peak mitral A velocity (cm/s) | 60 ± 24 |
Mitral E/A ratio | 1.7 ± 1.4 |
Deceleration time (ms) | 195 ± 84 |
Tissue Doppler measurements | |
Septal e’ velocity (cm/s) | 5.6 ± 2 |
Septal a’ velocity (cm/s) | 6.4 ± 2.5 |
Septal e’/a’ ratio | 1 ± 0.6 |
Septal E/e’ ratio | 17.4 ± 9.5 |
Lateral e’ velocity (cm/s) | 7.7 ± 3.4 |
Lateral a’ velocity (cm/s) | 6.3 ± 2.3 |
Lateral e’/a’ ratio | 1.46 ± 1.5 |
Lateral E/e’ ratio | 12.7 ± 7 |
Relation Between HE and LV Diastolic Function
Table 2 shows the results of two-dimensional and Doppler findings in the study sample divided according to the presence or absence of HE. There were no significant differences between the two groups in age, heart rate, and blood pressure ( P > .3). Peak mitral E and A velocities were higher in the 19 patients without HE, but this did not reach the level of statistical significance when adjusted for multiple comparisons. There were no significant differences in deceleration time or E/A ratio between the two groups. Septal e’ velocity was lower in the group without HE, although the difference was not statistically significant. Septal E/e’ ratio was significantly higher ( P < .05) in patients without HE. Patients with DCM without HE had higher lateral a’ velocity and E/e’ ratio, but neither reached statistical significance when adjusted for multiple comparisons.
DCM with HE ( n = 31) | DCM without HE ( n = 19) | |
---|---|---|
1 Age (y) | 55 ± 15 | 57 ± 11 |
2 Heart rate (/min) | 81 ± 17 | 77 ± 14 |
3 Systolic blood pressure (mm Hg) | 122 ± 17 | 128 ± 19 |
4 Diastolic blood pressure (mm Hg) | 76 ± 15 | 79 ± 9 |
5 End diastolic volume (mL) | 216 ± 75 | 225 ± 89 |
6 End systolic volume (mL) | 157 ± 68.5 | 161 ± 90 |
7 EF (%) | 29 ± 10 | 31 ± 12 |
8 LV mass (g) | 206 ± 70 | 197 ± 61 |
9 LA volume index (mL/m 2 ) | 53.6 ± 25 | 51 ± 25 |
10 Peak mitral E velocity (cm/s) | 77 ± 26 | 98 ± 37 |
11 Peak mitral A velocity (cm/s) | 54 ± 21 | 71 ± 28 |
12 E/A ratio | 1.26 (0.79–2.3) | 1.24 (0.65–2.3) |
13 Deceleration time (ms) | 187 (142−235) | 165 (127–198) |
14 Septal e’ velocity (cm/s) | 6.1 ± 2.1 | 4.7 ± 1.7 |
15 Septal a’ velocity (cm/s) | 5.6 ± 2 | 7.3 ± 2.5 |
16 Septal e’/a’ ratio | 1.1(0.64–1.45) | 0.57 (0.41−0.94) |
17 Septal E/e’ ratio | 13.3 (8.6−16) | 19 (14–28) ∗ |
18 Lateral e’ velocity (cm/s) | 7.6 (5–10) | 7.7 (5.6–9.5) |
19 Lateral a’ velocity (cm/s) | 5.6 ± 2 | 7.4 ± 2.6 |
20 Lateral e’/a’ ratio | 1.1 (0.8–1.7) | 1 (0.8–1.8) |
21 Lateral E/e’ ratio | 10.6 (7.9–13.9) | 12.6 (9–18) |
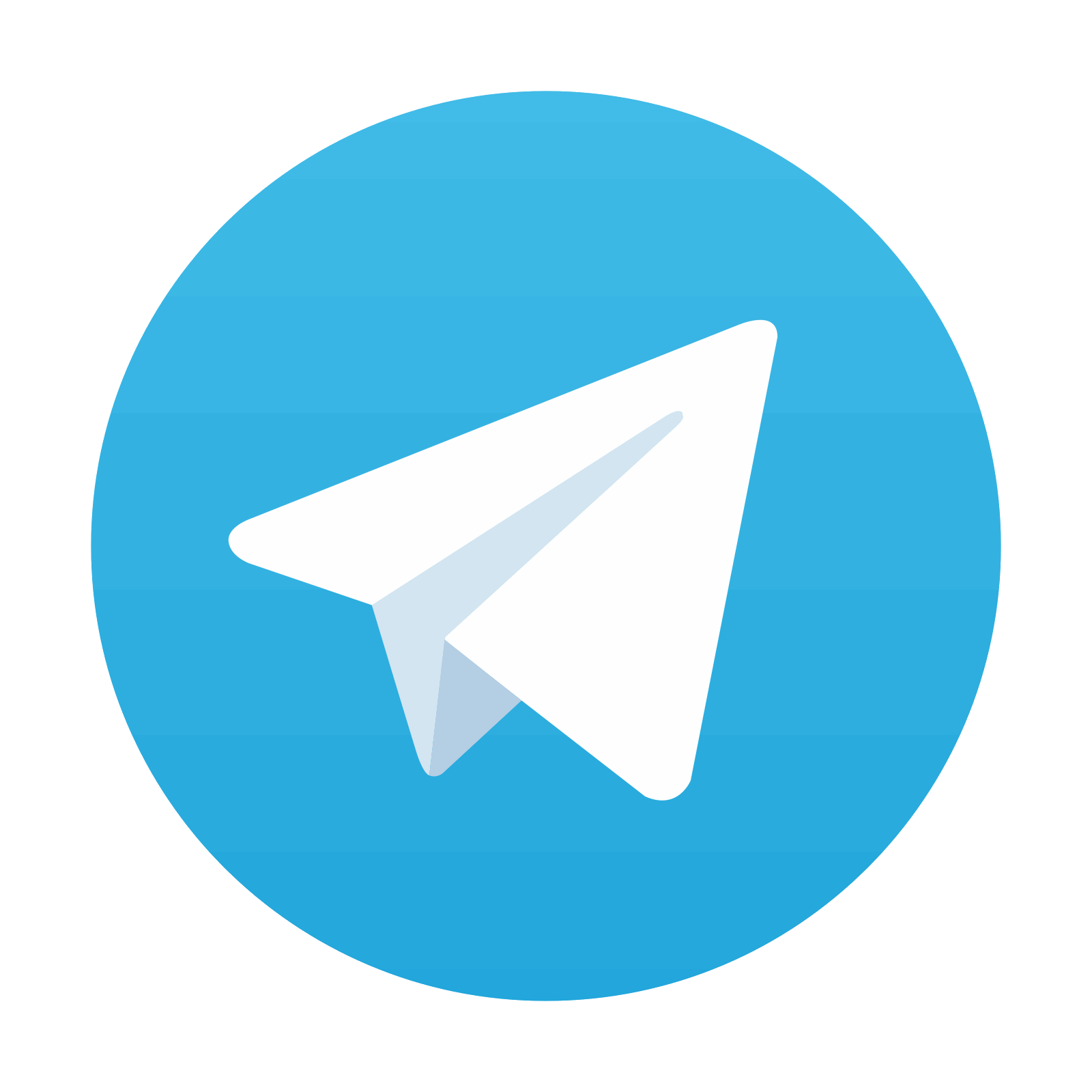
Stay updated, free articles. Join our Telegram channel

Full access? Get Clinical Tree
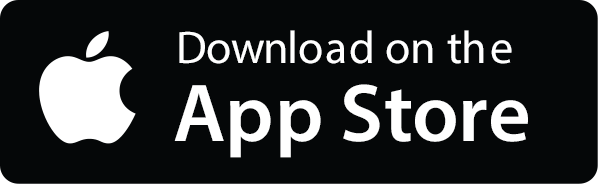
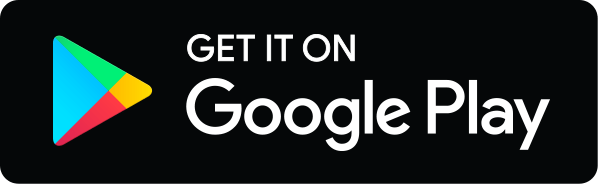
