Left ventricular (LV) diastolic dysfunction is associated with poor outcomes after tetralogy of Fallot (TOF) repair, although its cause is not known, and its relation to right ventricular (RV) performance has never been examined. The aim of this study was to test the hypothesis that RV dilation leads to LV diastolic dysfunction after TOF repair. Patients with repaired TOF who underwent cardiac catheterization and cardiac magnetic resonance imaging within 6 months from January 2003 and April 2011 were reviewed to assess the relation of LV end-diastolic pressure (LVEDP) and indexed RV end-diastolic volume (RVEDVi). Thirty-eight patients were included at a median age of 10.1 years (range 0.6 to 54.7). There was a significant linear association between RVEDVi and LVEDP (p = 0.05). RV end-diastolic pressure (p <0.001), right pulmonary artery systolic pressure (p = 0.009), left pulmonary artery systolic pressure (p = 0.02), and total cardiopulmonary support time (p = 0.04) during TOF repair were also significantly associated with LVEDP. Compared to patients with LVEDP <12 mm Hg, those with LVEDP ≥12 mm Hg had significantly higher mean RVEDVi (135.2 ± 47.8 vs 98.6 ± 28 ml/m 2 , p = 0.007) and mean RV end-diastolic pressure (11.7 ± 1.6 vs 8.5 ± 2.8 mm Hg, p = 0.0003). In conclusion, after TOF repair, LVEDP is significantly associated with RVEDVi. Furthermore, mean RVEDVi is significantly higher in patients with LVEDP ≥12 mm Hg. These findings support the theory that RV dilation may impair LV diastolic function and that LV parameters may also be important to consider in determining timing of pulmonary valve replacement.
Historically, right ventricular (RV) dilation and dysfunction have been the focus in the management of patients late after tetralogy of Fallot (TOF) repair. However, left ventricular (LV) performance can also be abnormal in these patients. LV systolic dysfunction has been shown to correlate with RV systolic dysfunction, and abnormal ventricular-ventricular interaction has been hypothesized to underlie this correlation. Recently, LV diastolic performance was shown to be an important risk factor for ventricular arrhythmias in patients with repaired TOF. In patients with implantable cardioverter-defibrillators for primary prevention of ventricular arrhythmias, LV end-diastolic pressure (LVEDP) ≥12 mm Hg was the strongest predictor of appropriate implantable cardioverter-defibrillator discharges. The mechanism of LV diastolic dysfunction in patients after TOF repair is not known, and the relation between LVEDP and RV dilation has never been examined. Similar to LV systolic dysfunction, we hypothesized that progressive RV dilation causes LV diastolic dysfunction via ventricular-ventricular interaction. To test this hypothesis, we examined whether indexed RV end-diastolic volume (RVEDVi) measured by magnetic resonance imaging (MRI) correlates with LVEDP measured at catheterization in patients with repaired TOF. In addition, we sought to determine if other clinical, catheter, and MRI-based variables were associated with LVEDP.
Methods
We retrospectively identified consecutive patients with repaired TOF who underwent cardiac catheterization within 6 months of cardiac MRI at The Children’s Hospital of Philadelphia from January 1, 2003, to April 1, 2011. To be included, the patient’s TOF repair had to include ventricular septal defect closure and relief of RV outflow tract obstruction. Inclusion also required that the patient’s cardiac catheterization involve direct measurement of LVEDP. We excluded patients with TOF and pulmonary atresia and major aortopulmonary collateral vessels, those with significant residual ventricular septal defects (defined as Qp/Qs >1.5 on oximetry at catheterization), patients with greater than mild aortic insufficiency (defined as >1+ on angiography or >20% aortic regurgitant fraction on MRI), patients with significant mitral regurgitation (defined on angiography as >1+ or >20% regurgitant fraction on MRI), and patients with significant LV outflow tract obstruction (defined as a gradient >20 mm Hg on catheterization). Patients with TOF and pulmonary atresia with ductal-dependent pulmonary blood flow but no major aortopulmonary collateral vessels were included. We also excluded patients who underwent transcatheter or surgical intervention between the measurement of LVEDP and cardiac MRI. If a patient underwent several catheterizations within 6 months of MRI, the catheterization done closest to the MRI study was chosen for review. This retrospective review was approved by the institutional review board of The Children’s Hospital of Philadelphia.
We reviewed the medical record of each included patient and extracted the following: date of birth, gender, age at catheterization, intracardiac anatomy, the presence of any type of coronary artery abnormality, the presence of any type of Pre-repair palliation (e.g., shunt, RV outflow tract stent, ductal stent), age at definitive repair, the use of transannular patch at repair, total support and cardiopulmonary bypass time during repair, and length of QRS interval on the most recent electrocardiogram before reviewed catheterization. We also recorded the dates of the catheterization and MRI under review and calculated the time between the 2 examinations.
All cardiac MRI studies were performed on either a 1.5-T Siemens Sonata or Avanto system (Siemens Medical Systems, Erlangen, Germany) using a standard protocol for studying patients with TOF. This includes the acquisition of phase-contrast magnetic resonance flow data for quantification of the forward and reverse flows measured at the main pulmonary artery and ventricular cine volume data for measurement of the ventricular end-diastolic and end-systolic volumes, stroke volume, and ejection fraction. Flow and ventricular volume data were analyzed using Argus software (Siemens Medical Systems). The MRI reports of included patients were reviewed, and the following data were extracted: the RV ejection fraction, RVEDVi, the LV ejection fraction, indexed LV end-diastolic volume (LVEDVi), and pulmonary valve regurgitant fraction.
All cardiac catheterizations were clinically indicated at the discretion of the patient’s referring cardiologist. Procedures were performed under general endotracheal anesthesia or conscious sedation at the discretion of the interventional cardiologist. All catheterizations involved the collection of comprehensive right- and left-sided oximetric and hemodynamic data under 21% inhaled oxygen using standard techniques. The cardiac catheterization reports of included patients were reviewed, and the following data extracted: LVEDP, RV end-diastolic pressure (RVEDP), RV systolic pressure, cardiac index, right pulmonary artery systolic and diastolic pressures, left pulmonary artery systolic and diastolic pressures, pulmonary vascular resistance, and Qp/Qs.
Demographic, clinical, MRI-based, and catheter-based variables were summarized using standard descriptive statistics and are expressed as mean ± SD for normally distributed continuous variables, as medians with ranges for skewed continuous variables, and as counts with percentages of the total for nominal variables. To test the associations of demographic, clinical, MRI-based, and catheter-based variables with LVEDP, univariate and multivariate linear regression models were created. Variables with p values <0.20 in univariate testing were considered for inclusion in the multivariate model and retained if p <0.05 or if they showed evidence of significant confounding. Variables highly collinear with the outcome variable and those with >20% missing observations were not included in the final multivariate model. We also divided patients into those with LVEDP <12 mm Hg and those with LVEDP ≥12 mm Hg and performed an unpaired Student’s t test or Wilcoxon’s rank-sum test to determine if mean or median demographic, clinical, MRI-based, and catheter-based variables significantly differed between the 2 groups. Analysis of variance was performed to determine if LVEDP differed significantly within categorical variables. Statistical significance was established at p <0.05. All statistical analyses were performed using Stata version 10 (StataCorp LP, College Station, Texas).
Results
Thirty-eight patients met the inclusion criteria, and their demographic, clinical, catheter-derived, and MRI-derived variables are listed in Table 1 . All patients had undergone definitive repair, with a median time from repair of 9.5 years (range 0.3 to 40.9). The median number of days between catheterization and MRI was 10.5 (range 0 to 182). Most of the patients had the usual form of TOF (58%), but some had TOF with pulmonary atresia (26%), TOF with absent pulmonary valve syndrome (11%), or TOF with complete common atrioventricular canal (5%). Only 3 patients (8%) had coronary anomalies; in all 3, the left anterior descending coronary artery arose from the right coronary artery. Definitive repair for each of these patients included conduit placement. Thirty-four patients had data available regarding the nature of the definitive repair; 23 of these (68%) had transannular patches placed, and 11 (32%) had conduits placed. Nine of the patients who underwent conduit replacement had TOF with pulmonary atresia, 1 patient had TOF with absent pulmonary valve syndrome, and 1 patient had TOF.
Variable | Value |
---|---|
Demographic | |
Age at catheterization (years) | 10.1 (0.6–54.7) |
Men | 26 (68%) |
Weight (kg) | 26.7 (5.3–95.8) |
Height (cm) | 123.5 (61–182.5) |
Body surface area (kg/m 2 ) | 0.95 (0.3–2.1) |
Time between catheterization and surgical repair (years) | 9.5 (0.3–40.9) |
Time between catheterization and MRI (days) | 10.5 (0–182) |
Anatomic | |
TOF | 22 (58%) |
TOF with pulmonary atresia | 10 (26%) |
TOF with absent pulmonary valve | 4 (11%) |
TOF with complete common atrioventricular canal | 2 (5%) |
Coronary anomaly | 3 (8%) |
Surgical | |
Pre-repair palliation | 10 (26%) |
Age at repair | 127 days (3 days to 13.8 years) |
Transannular patch (n = 34) | 23 (68%) |
Conduit (n = 34) | 11 (32%) |
Total support time (minutes) (n = 25) | 79 (48–185) |
Cardiopulmonary bypass time (minutes) (n = 25) | 54 (21–185) |
Electrocardiography | |
QRS duration (ms) (n = 37) | 124 (74–180) |
Catheterization | |
LVEDP (mm Hg) | 10.3 ± 3.0 |
RVEDP (mm Hg) | 9.7 ± 2.8 |
Cardiac index (L/min/m 2 ) | 3.0 ± 0.9 |
Right pulmonary artery systolic pressure (mm Hg) (n = 37) | 28.7 ± 9.6 |
Left pulmonary artery systolic pressure(mm Hg) (n = 33) | 24.5 ± 8.7 |
Indexed pulmonary vascular resistance (Wood units) (n = 32) | 2.1 ± 0.9 |
MRI | |
RVEDVi (ml/m 2 ) (n = 36) | 112.8 ± 40.6 |
RV ejection fraction (%) (n = 36) | 54.7 ± 10.1 |
LVEDVi (ml/m 2 ) (n = 36) | 59.5 ± 14.8 |
LV ejection fraction (%) (n = 36) | 65.6 ± 8.1 |
Main pulmonary artery regurgitant fraction (%) (n = 37) | 33 ± 16 |
For the 36 patients in whom RVEDVi was available, there was a significant linear association between catheterization-derived LVEDP and MRI-derived RVEDVi ( Figure 1 ) . Table 2 lists the univariate linear regression analysis of the relation between LVEDP and other patient variables. LVEDP was found to be significantly associated with RVEDP (p <0.001), right pulmonary artery systolic pressure (p = 0.009), left pulmonary artery systolic pressure (p = 0.02), and total cardiopulmonary support time during TOF repair (p = 0.04). When RVEDVi and RVEDP were included in a multivariate linear regression model, RVEDP remained statistically associated with LVEDP (p <0.001), while the association between RVEDVi and LVEDP no longer reached statistical significance (p = 0.12). Right pulmonary artery and left pulmonary artery systolic pressure were collinear with LVEDP and not included in the multivariate model. Total support time was missing >20% of observations (n = 25) and thus was not included in the multivariate model.
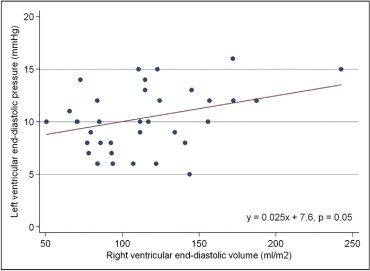
Variable | n | Coefficient | 95% Confidence Interval | p Value |
---|---|---|---|---|
MRI | ||||
RVEDVi | 36 | 0.025 | 0.00004 to 0.05 | 0.05 |
RV ejection fraction | 36 | −0.02 | −0.12 to 0.09 | 0.77 |
Main pulmonary artery regurgitant fraction | 37 | 0.05 | −0.02 to 0.11 | 0.17 |
LV ejection fraction | 36 | 0.02 | −0.11 to 0.15 | 0.79 |
LVEDVi | 36 | 0.04 | −0.04 to 0.1 | 0.32 |
Catheterization | ||||
RVEDP | 38 | 0.74 | 0.48 to 0.99 | <0.001 |
RV systolic pressure | 38 | 0.02 | −0.04 to 0.08 | 0.53 |
Right pulmonary artery systolic pressure | 37 | 0.13 | 0.04 to 0.23 | 0.009 |
Left pulmonary artery systolic pressure | 33 | 0.14 | 0.02 to 0.26 | 0.02 |
Indexed pulmonary vascular resistance | 32 | −0.13 | −1.39 to 1.11 | 0.83 |
Cardiac index | 38 | 0.62 | −0.5 to 1.74 | 0.27 |
Clinical/surgical | ||||
QRS duration | 37 | 0.02 | −0.01 to 0.05 | 0.19 |
Age at repair | 38 | 0.0005 | −0.0003 to 0.001 | 0.19 |
Time from repair | 38 | 0.06 | −0.03 to 0.15 | 0.19 |
Cardiopulmonary bypass time | 25 | 0.01 | −0.02 to 0.05 | 0.43 |
Total support time | 25 | 0.04 | 0.0019 to 0.07 | 0.04 |
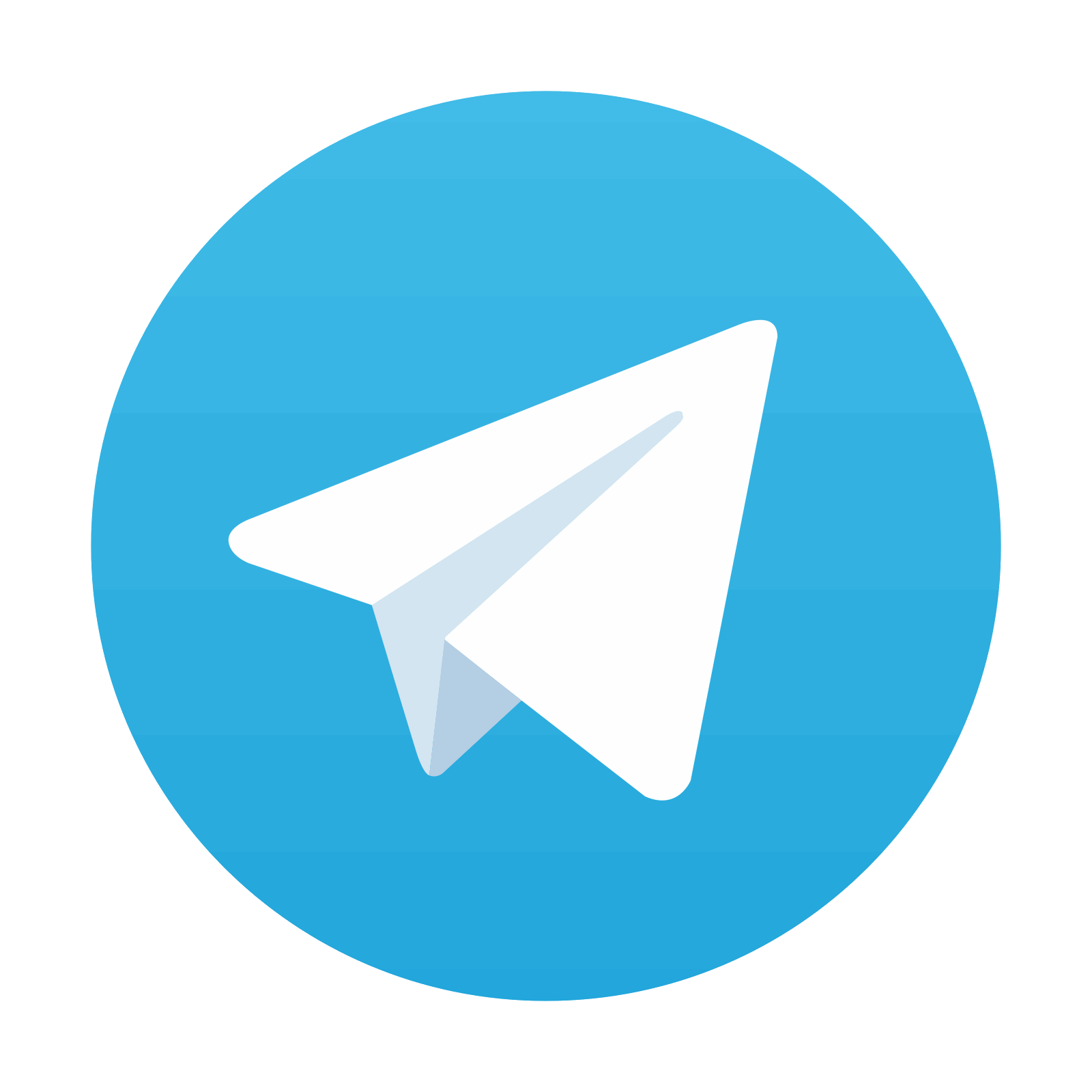
Stay updated, free articles. Join our Telegram channel

Full access? Get Clinical Tree
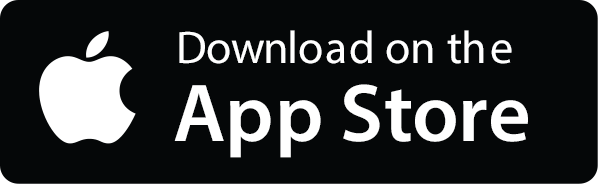
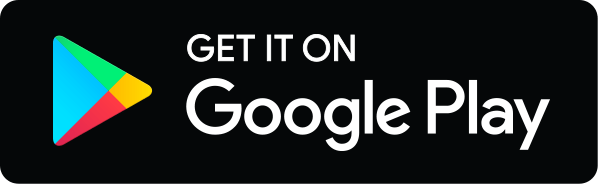
