This study aims to characterize flow, volume, and strain of the left atrium in hypertrophic cardiomyopathy (HC) with atrial fibrillation (AF) using cine cardiovascular magnetic resonance (CMR) imaging. Cine CMR data for 144 patients with HC, including 29 patients with episodes of paroxysmal AF and 13 patients with persistent AF, were retrospectively analyzed. The vortex flow of the left atrial (LA, %) was measured using a vortex flow map and was used as an estimate of flow. The LA end-systolic volume index (ml/m 2 ), LA ejection fraction (%) and global peak longitudinal LA strain (%) derived from a feature-tracking method were used as estimates of volume and strain. Vortex flow of the LA in patients with paroxysmal AF was significantly smaller than in patients without AF (vertical long-axis view; 26.7 ± 10.8% vs 33.2 ± 12.2%, p <0.005). The patients with paroxysmal AF had greater LA end-systolic volume index and global peak longitudinal LA strain and lower LA ejection fraction compared with those without AF. In conclusion, patients with HC with paroxysmal AF are characterized by small vortex flow, large volume, and decreased strain of LA on cine CMR.
Atrial fibrillation (AF) is significantly more common in patients with hypertrophic cardiomyopathy (HC) than in the general population. Its prevalence depends on the severity of the disease, with 22% in a general cohort and up to 32% in a cohort before implantable cardioverter defibrillator and pacemaker implantation. The incidence for de novo AF in a general HC cohort is about 2% per year. AF carries a higher risk of stroke and can be considered an independent predictor of cardiac death. AF is often asymptomatic and is detected by chance as a cause of heart failure or a stroke. , Recently, left atrial (LA) imaging studies that used cardiovascular magnetic resonance (CMR) imaging and echocardiography reported that increased LA size and LA late gadolinium enhancement (LA-LGE) are strong predictors of AF in patients with HC. , However, detecting LA-LGE is challenging because of the thin LA wall that requires high spatial resolution for optimal imaging. Thus, the tissue characterization of the LA is limited, unlike that of the myocardium of the left ventricle (LV). Recently, the development of functional analysis software such as flow image and strain analysis in CMR has been remarkable. , This study aimed to characterize the LA that develops paroxysmal AF in HC according to flow, volume, and strain, calculating these values from the same cine CMR images with different approaches. In addition, the study aimed to determine the best indicator of AF onset by combining the resulting indexes.
This retrospective cohort study was approved by the institutional research ethics board of our institute with a waiver of written informed consent which was obtained from all patients. This study consisted of 144 consecutive patients with HC who had been diagnosed with HC by CMR imaging during their first clinic visit or had already been diagnosed with HC by echocardiography and underwent CMR imaging for follow-up between March 2014 and January 2020. HC is diagnosed according to the European Society of Cardiology clinical practice guideline. The primary diagnostic criterion is LV wall thickening of ≥15 mm at end-diastole in 1 or more segments by echocardiography or CMR. In cases with a wall thickness ≥13 mm, the criterion is patients with confirmed familial HC or diffuse LGE in the hypertrophic myocardium. Patients were divided into 3 groups: non-AF, paroxysmal AF, and persistent AF groups. Paroxysmal AF was defined as AF that terminated either spontaneously or with medical intervention within 7 days of AF onset. , History of paroxysmal AF before CMR imaging was confirmed by the examination of electrocardiographic and Holter recordings. Patients with paroxysmal AF received Holter monitoring or an electrocardiogram (ECG) to confirm the resolution of AF within the 7-day window. Patients without AF who underwent pulmonary vein isolation before CMR were excluded from this study. If ECG dysynchrony or artifacts because of cardiac devices were seen in the LA, the image quality was considered unacceptable. Patients with these image degradations were excluded.
Among patients with suspected cardiomyopathy who underwent CMR, controls were defined as those with normal cardiac function, negative LGE, and normal myocardial T1 values.
All patients were examined using a 3.0T magnetic resonance (MR) imaging (MRI) scanner (Ingenia, Philips Medical Systems, Best, The Netherlands) with a 4-element phased array coil in the supine position with breath-holds during expiration and ECG gating. Short-axis (SA), vertical long-axis (VLA) and horizontal long-axis (HLA) cine images were acquired using cine-balanced turbo field echo sequences (repetition time, 2.8 ms; echo time, 1.4 ms; flip angle, 45°; slice thickness, 8 mm; field of view, 380 mm; matrix size, 176 × 193; SENSE Factor 2). The slice thickness is 10 mm and the gap is 0. There were 20 phases per cardiac cycle, resulting in a mean temporal resolution of 45 ms. Late gadolinium enhancement (LGE) imaging was performed using Look-Locker sequence approximately 12 to 15 minutes after 0.1 mmol/kg gadoteric meglumine (Magnescope, Guerbet, Japan) had been administered. The LV end-diastolic volume and LV end-systolic volume were analyzed semiautomatically at the basal to apical levels using LV SA cine images, followed by manual correction with available software (Ziostation 2, Ziosoft, Tokyo, Japan). End-diastolic and end-systolic phases were identified visually on those images showing the largest and smallest LV cavity areas, respectively.
Based on the pixel and feature-tracking methods, we modified the myocardial strain analysis for cine-tagging using existing software (Ziostation 2) and developed a vortex flow mapping (VFM) that enables quantification and visualization of turbulent flow. Cine-tagging MRI which is a cine image with a grid of tags of the heart has a unique ability to qualify myocardial motion using tag patterns that deform according to the motion of the heart, which aids in tracking motion during contraction and dilation for a series of MR images. Therefore, turbulent flow with nonuniform magnetic fields are more emphasized and laminar flows with equal magnetic fields are less visualized. VFM is configured by tracking each pixel, followed by automatic extraction of the pixel movement occurring throughout a cardiac cycle. First, the cine image with the maximum cross section of the LA on VLA and HLA views was selected. Second, we delineated the LA cavity contour as a region of interest (ROI) and determined the center of the long and short diameter of the LA on the initial frame of the cine image. Third, VFM automatically tracked all pixels in the ROI and calculated the pixel movement for the ROI at each phase during the total pixel movement throughout a cardiac cycle. The vector of pixel movement is resolved into circumferential and radial components. Movement in the circumferential direction is defined as VF. VF % meant the percentage of the distance moved in the circumferential direction. It was obtained from the equation:
- (1)
VF (%) = D(t) ÷ D
D(t) was the distance moved by the pixel at time t. T indicated 1 phase arbitrarily selected from the phases obtained by dividing 1 cardiac cycle into 20 phases. D(1) was the total distance moved by the pixel throughout a cardiac cycle. One cardiologist (RN) and 1 radiologist (MN) evaluated the image quality and performed VFM analysis. The highest magnitude of VF was used as the representative value for each case. On the VFM, high VF is shown in hot colors and low VF or stagnation in cold colors ( Figure 1 ).

CMR-feature tracking (CMR-FT) derived strain analysis was performed using dedicated software (Vitrea, Canon Medical Systems, Tochigi, Japan). The same VLA and HLA cine images used for VFM were used for strain and LA volume measurements. First, the endocardial and epicardial borders were manually drawn in the end-diastolic frame. The LA appendage and pulmonary veins were excluded from the endocardial contour. These were then automatically propagated through the cardiac cycle by matching individual patterns representing anatomic structures. Second, LA volume curves were generated during each cardiac cycle. VLA and HLA cine images were divided into 6 myocardial segments. In the HLA images, the anterior and posterior walls are divided equally into 3 segments each, and in the VLA images, the anterior and inferior walls are divided equally into 3 segments each. This process is done automatically by the CMR-FT software. Global peak longitudinal LA strain (GLAS) was measured from the longitudinal strain curves in all LA segments obtained in the 2 views. Longitudinal strain rate curves were used to compute LA strain rates during late ventricular diastole (SR-ld) represented the LA booster pump function, respectively. Measurements for LA end-diastolic volume index (LAEDVI) and left atrial end-systolic volume index (LAESVI) were extracted from the volume and/or time curves. Figure 2 shows an example of CMR-FT.

Data are expressed as mean ± SD for normally distributed continuous variables, medians (interquartile ranges) for skewed continuous variables, and counts (percentage of total) for categoric variables. Testing of differences in demographic and clinical data based on AF and non-AF was accomplished with either the unpaired t test or the Wilcoxon rank-sum test for continuous variables and with either chi-square test or Fisher’s exact test for categoric variables, as appropriate. Statistical significance was established using a 2 tailed p <0.05. Spearman’s correlation test was used to test for correlation between numeric continuous variables and vortex flow of the left atrial vortex flow (LA-VF) percentage. Univariate and multivariate Cox proportional hazard regression models were used to evaluate predictive factors for AF. All statistical analyses were performed using the JMP statistical program package (version 15.0; JMP, Inc., Cary, North Carolina).
The interstudy variability was assessed by intraclass correlation coefficient (ICC). Agreement was considered excellent for ICC >0.74, good for ICC = 0.60 to 0.74, fair for ICC = 0.40 to 0.59, and poor for ICC <0.40.
Patients with HC were divided into a persistent AF group of 13 patients, paroxysmal AF group of 29 patients, and a non-AF group of 102 patients according to the AF documentation. All 29 patients in the paroxysmal AF group had at least 1 episode of paroxysmal AF within 12 months of CMR (median 2.5 episodes). A total of 24 patients were diagnosed with paroxysmal AF before CMR imaging. Previous pulmonary vein isolation before CMR was performed in 10 patients in the paroxysmal AF group. A total of 7 patients had cerebrovascular disease, 1 in the persistent AF group and 2 in the paroxysmal AF group. The frequency of obstructive HC did not differ between the 2 groups. Patients with paroxysmal AF had significantly larger body surface area (BSA) than patients without AF (1.81 ± 0.04 vs 1.68 ± 0.02, p <0.05). Body mass index (BMI) for paroxysmal AF group was significantly higher than that for non-AF group (25.8 ± 0.7 vs 22.9 ± 0.4, p <0.001). There was no significant difference in brain natriuretic peptide among non-AF, paroxysmal AF, and persistent AF group. Antiarrhythmic therapy included amiodarone, β blockers, sodium channel blockers, non-dihydropyridine calcium channel blockers, and digitalis. Amiodarone was used in 9 patients, of which 2 were in the persistent AF group, 5 in the paroxysmal AF group, and 2 in the non-AF group, with a significant difference in the rate of use (p = 0.0040). Also, patients on amiodarone had a significant decrease in LA function (increased LA size and lower LA ejection fraction [LA-EF]) compared with patients who did not take amiodarone. Beta blockers were administered to 86 patients, sodium channel blockers to 9 patients, non-dihydropyridine calcium channel blockers to 3 patients, and digitalis to 1 patient. There was no significant difference in the use of these medicines among the 3 groups. In addition, there was no association between taking these medications and LA functional parameters. The patients’ characteristics are summarized in Table 1 .
Variable | Non-AFgroup(n=102) | ParoxysmalAF group(n=29) | PersistentAF group(n=13) | p Value(paroxysmal AFvs non-AF) | p Value(paroxysmal AFvs persistent AF) |
---|---|---|---|---|---|
Age (years) | 59.0 [51.0-69.0] | 63.0 [50.8-70.0] | 70.0 [65.0-77.0] | 0.36 | <0.01 |
Men | 69 (65.7%) | 21 (70.0%) | 11 (84.6%) | 0.68 | 0.31 |
Time since diagnosis of HC (months) | 76.9 ±118.0 | 93.6±158.6 | 68.2±121.7 | 0.53 | 0.57 |
BSA (m 2 ) | 1.68±0.02 | 1.81±0.04 | 1.74±0.05 | <0.005 | 0.30 |
BMI (kg/m 2 ) | 22.9±0.4 | 25.8±0.7 | 24.6±1.25 | <0.001 | 0.42 |
HOCM | 19 (18.1%) | 3 (10.0%) | 4 (30.8%) | 0.29 | 0.09 |
APH | 46 (43.8%) | 18 (60.0%) | 7 (53.9%) | 0.12 | 0.71 |
dHCM | 6 (5.7%) | 4 (13.3%) | 2 (15.4%) | 0.16 | 0.86 |
NYHA class Ⅰ | 86 (81.9%) | 19 (63.3%) | 6 (46.2%) | <0.05 | 0.22 |
NYHA class Ⅱ | 19 (18.1%) | 11 (36.7%) | 6 (46.2%) | <0.05 | 0.21 |
NYHA class Ⅲ, Ⅳ | 0 (0.0%) | 0 (0.0%) | 1 (8.0%) | – | – |
Previous CVA | 5 (4.8%) | 2 (6.7%) | 1 (7.7%) | 0.68 | 0.90 |
BNP | 87.0 [37.0-191.2] | 102.9 [49.0-158.0] | 299.8 [189.7-572.0] | 0.89 | <0.005 |
Medications | |||||
Amiodarone | 2 (2.0%) | 5 (17.2%) | 2 (15.4%) | 0.0012 | 0.74 |
B blocker | 60 (57.1%) | 19 (63.3%) | 11 (84.6%) | 0.54 | 0.16 |
Na blocker | 4 (3.9%) | 4 (13.8%) | 1 (7.7%) | 0.05 | 0.69 |
ACEI/ARB | 49 (46.7%) | 14 (46.7%) | 7 (53.9%) | 1.00 | 0.67 |
Diuretics | 8 (7.6%) | 6 (20.0%) | 5 (38.5%) | 0.05 | 0.20 |
Statin | 33 (31.4%) | 4 (13.3%) | 6 (46.2%) | 0.05 | <0.05 |
Ca blocker | 28 (26.7%) | 9 (30.0%) | 4 (30.8%) | 0.72 | 0.95 |
DOAC | 1 (1.0%) | 18 (60.0%) | 4 (30.8%) | <0.0001 | 0.08 |
Warfarin | 5 (4.8%) | 5 (16.7%) | 7 (53.9%) | <0.05 | <0.05 |
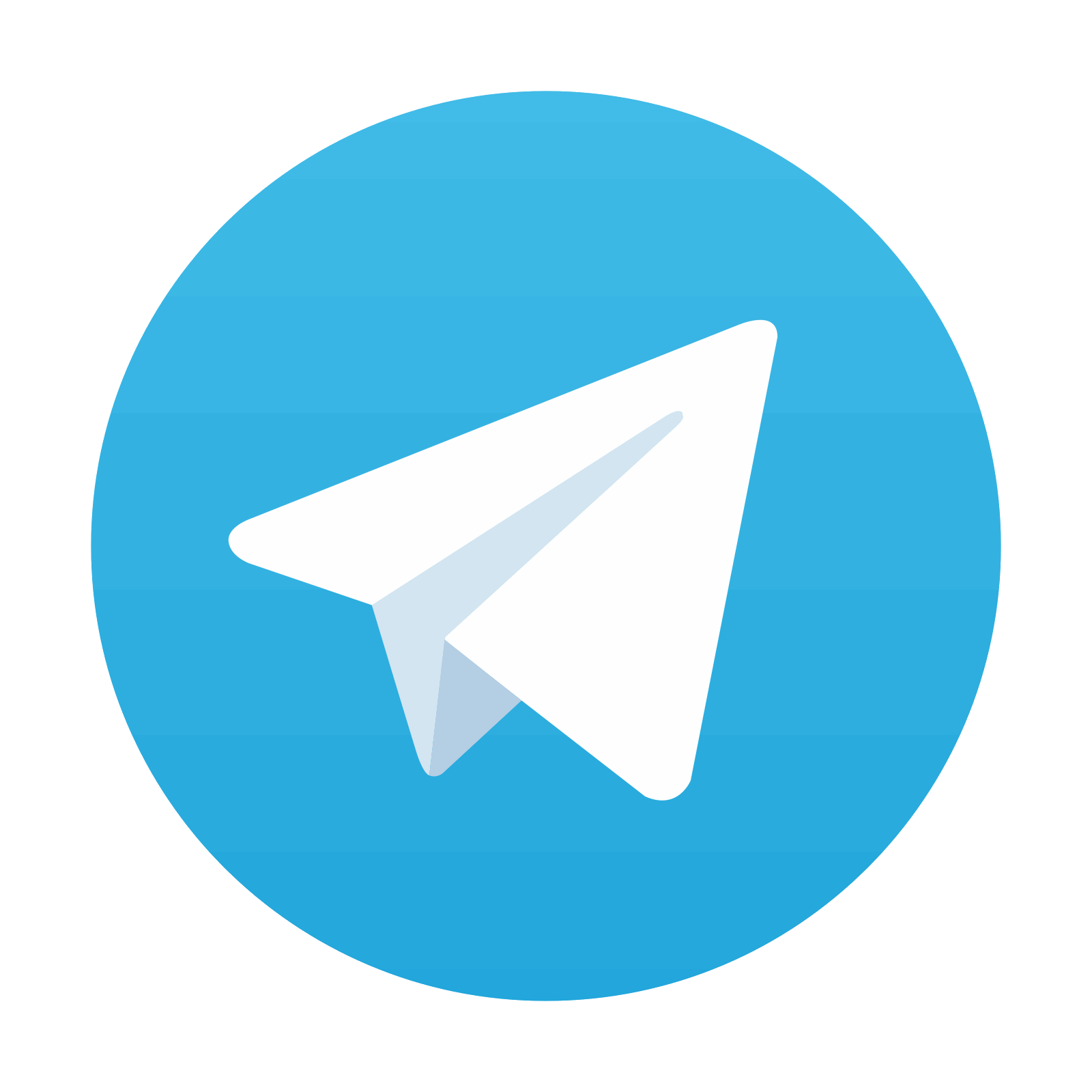
Stay updated, free articles. Join our Telegram channel

Full access? Get Clinical Tree
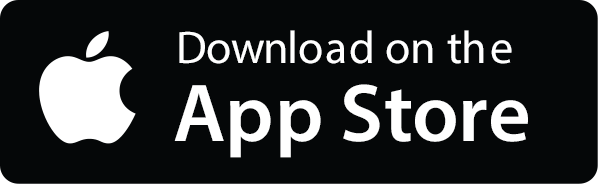
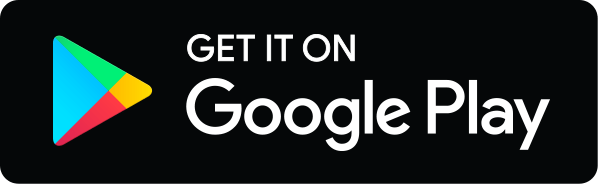
