Inflammation is a crucial element in the development of cardiovascular disease. Serum measurements of inflammation through high-sensitivity C-reactive protein (hsCRP) can lead to improved risk stratification of patients for risk for hard cardiovascular events. There is evidence that anthropomorphic measurements of obesity are important determinants of systemic inflammation. Online databases (e.g., PubMed and Medline) were searched for original research reports published in English from June 1990 to June 2009 examining the relations between hsCRP; anthropomorphic measurements of obesity including body mass index, waist-to-hip ratio, waist circumference, and visceral adiposity; and cardiovascular disease. When possible, correlation coefficients were used to compare data among studies. Measurements of abdominal obesity are associated with systemic inflammation as measured by hsCRP (r = 0.40 to 0.61). The association between hsCRP and abdominal adiposity persists when taking into account body mass index. Elevation of hsCRP might be reversible with weight loss and exercise. In conclusion, clinical measurements of abdominal adiposity readily provide data elucidating the systemic inflammatory state of patients and can help guide intensity of lifestyle modifications, thus leading to reduction of this inflammation.
Increasing evidence suggests that inflammation is a key element of atherosclerosis. Identifying patients with subclinical inflammation appears useful in identifying cardiovascular risk not otherwise accounted for in traditional risk scoring. C-reactive protein (CRP) is the best-studied marker of subclinical inflammation and has been shown to predict cardiovascular outcomes as well as response to statin therapy, independent of low-density lipoprotein cholesterol level. CRP is rarely elevated in the absence of the metabolic syndrome, which is a constellation of interrelated risk factors that arise from insulin resistance and abdominal obesity. In this editorial review, we demonstrate that anthropomorphic measures of visceral adiposity are closely correlated with CRP, are simple to perform, and thus may be used to improve cardiovascular risk stratification and to direct therapy in patients who might not otherwise be candidates for aggressive pharmacologic and lifestyle treatment.
The Emerging Role of C-Reactive Protein in Clinical Practice
High-sensitivity CRP (hsCRP) is a simple, relatively inexpensive, and widely available blood test used to detect inflammation, including subclinical inflammation. In case-control and prospective studies, hsCRP levels have been shown to consistently improve risk prediction for cardiovascular disease (CVD) in various populations, even when lipid values are less than the National Heart, Lung, and Blood Institute’s guidelines for optimal lipid control.
Despite the association with CVD, CRP may not play a direct causal role in its pathophysiology. In a recent Mendelian genetics study of the CRP gene locus, CRP gene variants were closely associated with CRP levels but did not predict the development of coronary artery disease. Across the entire population however, CRP levels were closely associated with the development of coronary artery disease.
Currently, measurement of hsCRP is recommended for adults at “intermediate risk” by the Centers for Disease Control and Prevention as well as the American College of Cardiology. By adding hsCRP to traditional risk factors, 30% to 40% of intermediate-risk patients (those with 10% to 20% risk for hard coronary artery disease events in 10 years) can be reclassified as either high or low risk. This reclassification may enable more refined treatment decisions, for example, more judicious use of aspirin and statin therapy. The inclusion of hsCRP as well as family history in global risk assessment has been shown to contribute to prediction of cardiovascular events at least as much as high-density lipoprotein cholesterol and low-density lipoprotein cholesterol.
The role of pharmacotherapy to reduce inflammation is an active area of investigation. Statins lower hsCRP levels as well as low-density lipoprotein cholesterol. The landmark Justification for the Use of Statins in Primary Prevention: An Intervention Trial Evaluating Rosuvastatin (JUPITER) study showed that treating patients aged >60 years old with normal low-density lipoprotein cholesterol but hsCRP ≥2 mg/dl can dramatically reduce cardiovascular event rates in men, women, and nearly all ethnic groups (cardiovascular events were defined as myocardial infarction, stroke, arterial revascularization, hospitalization for unstable angina, or death from cardiovascular causes).
JUPITER raised many questions about the use of hsCRP. Is lowering hsCRP a key mechanism by which statins decrease cardiovascular events? Are statins simply lowering event rates in patients with elevated metabolic risk as identified by subclinical inflammation? Are there other, simpler ways of identifying patients at risk for subclinical inflammation, such as with routine anthropomorphic measures of obesity and estimates of insulin resistance?
Physiology of Obesity and Inflammation
Obesity is increasingly recognized as an inflammatory disorder. Research from the past decade has elucidated many of the mechanistic associations between obesity, particularly highly metabolically active visceral adiposity, and inflammation. A driving factor in this process is caloric excess, which causes distinct morphologic and metabolic changes in adipose tissue as well as other organ systems, including the pancreas, hypothalamus, skeletal muscle, and liver. These processes lead to an inflammatory infiltration of adipose tissue, aberrant endocrine secretion, and cytokine secretion, all of which promote an increase in systemic inflammation.
At the adipose tissue level, an abundance of triacylglycerol creates large adipocytes that initiate cellular stress responses, leading to the activation of inflammatory signaling pathways. Increased serum levels of free fatty acid and free fatty acid breakdown products trigger proinflammatory cascades, which in turn yield elevated cytokine secretion, promoting an inflammatory milieu. Release of these cytokines (so-called adipokines) promotes the recruitment of monocytes, which are then shunted down the inflammatory pathway to become inflammatory macrophages.
Activated macrophages themselves are essential to the local production of nitric oxide synthase and tumor necrosis factor–α and the expression of interleukin-6, stimulating further expression of CRP. An intriguing finding is that the levels of macrophage invasion of adipose tissue are directly related to the level of obesity and that this process can be reduced with exercise.
It has also been suggested that the association between abdominal obesity and subclinical inflammation may have an anatomic cause. The portal vein drains visceral adipose tissue and terminates at the liver, supplying 80% of its blood supply. It is possible that the direct exposure of cytokines from the adipose tissue to the liver induces greater inflammation. Furthermore, in a nutrient excess state, accumulation of triacylglycerol and free fatty acids overwhelms cellular respiratory machinery in the liver, leading to the accumulation diacylglycerol and ceramide. These molecules are powerful stimulators of the serine kinases IκB kinase and c-Jun-aminoterminal kinase cascades.
Activation of the c-Jun-aminoterminal kinase in liver and adipose tissues leads to increased activated protein 1, whereas increased IκB kinase in liver promotes the activation of nuclear factor κB. These inflammatory cascades, as well as inflammation induced by microhypoxic conditions found in obesity, yield the expression of a broad range of cytokines, including tumor necrosis factor–α, monocyte chemoattractant protein–1, chemokine (C-C motif) ligand 2, interleukin-6, and interleukin-1B, which leads to increased expression of CRP in the liver ( Figure 1 ).
Adiponectin levels provide another link between abdominal adiposity and the metabolic syndrome. Adiponectin, produced in adipose tissue, increases in lean individuals and decreases with increasing abdominal adiposity. Adiponectin affects insulin sensitivity by increasing fatty acid oxidation and improving glucose homeostasis. Specifically, adiponectin activates the peroxisome proliferator-activated receptor–α and adenosine monophosphate-activated protein kinase. The activation of peroxisome proliferator-activated receptor–α and monophosphate-activated protein kinase in liver and skeletal muscle yields increased fatty acid oxidation and decreased triglyceride tissue content, which leads to increased insulin sensitivity. Monophosphate-activated protein kinase activity decreases gluconeogenesis in the liver and increases glucose uptake in skeletal muscle, thus improving glucose homeostasis.
Obesity can be assessed by anthropomorphic measures; imaging modalities including computed tomography, magnetic resonance imaging, and dual-energy x-ray absorptiometry; and bioimpedance and skin-fold thickness. Imaging studies offer a direct measure of subcutaneous and visceral adipose tissue, but they are as of yet investigational and not used routinely in clinical practice. Clinical measures of obesity include body mass index (BMI), waist circumference (WC), and waist-to-hip ratio (WHR). BMI is calculated by dividing the weight in kilograms by the height in meters squared. BMI does not provide any information about body fat distribution or differentiate between fat and lean mass.
According to National Institutes of Health guidelines, WC is best measured at the top of the right iliac crest using an inelastic tape measuring parallel to the floor at the end of normal expiration, with obesity being defined as >40 inches in men and >35 inches in women. Hip circumference is measured as the widest point over the buttocks and below the iliac crest. The WHR found by dividing the WC by the hip circumference. There is some evidence that WC has a stronger association with visceral adiposity compared to WHR when measured by direct computed tomographic imaging. A large meta-analysis comparing WC and WHR measures to future CVD events found WHR measures to be less reliable in repeated measurements, likely because of technical problems with the need for disrobing and proper positioning of the measuring tape. There are not yet accurate and reliable methods for measuring bioimpedance and skin-fold thickness that have been broadly applied at the clinical level.
Physiology of Obesity and Inflammation
Obesity is increasingly recognized as an inflammatory disorder. Research from the past decade has elucidated many of the mechanistic associations between obesity, particularly highly metabolically active visceral adiposity, and inflammation. A driving factor in this process is caloric excess, which causes distinct morphologic and metabolic changes in adipose tissue as well as other organ systems, including the pancreas, hypothalamus, skeletal muscle, and liver. These processes lead to an inflammatory infiltration of adipose tissue, aberrant endocrine secretion, and cytokine secretion, all of which promote an increase in systemic inflammation.
At the adipose tissue level, an abundance of triacylglycerol creates large adipocytes that initiate cellular stress responses, leading to the activation of inflammatory signaling pathways. Increased serum levels of free fatty acid and free fatty acid breakdown products trigger proinflammatory cascades, which in turn yield elevated cytokine secretion, promoting an inflammatory milieu. Release of these cytokines (so-called adipokines) promotes the recruitment of monocytes, which are then shunted down the inflammatory pathway to become inflammatory macrophages.
Activated macrophages themselves are essential to the local production of nitric oxide synthase and tumor necrosis factor–α and the expression of interleukin-6, stimulating further expression of CRP. An intriguing finding is that the levels of macrophage invasion of adipose tissue are directly related to the level of obesity and that this process can be reduced with exercise.
It has also been suggested that the association between abdominal obesity and subclinical inflammation may have an anatomic cause. The portal vein drains visceral adipose tissue and terminates at the liver, supplying 80% of its blood supply. It is possible that the direct exposure of cytokines from the adipose tissue to the liver induces greater inflammation. Furthermore, in a nutrient excess state, accumulation of triacylglycerol and free fatty acids overwhelms cellular respiratory machinery in the liver, leading to the accumulation diacylglycerol and ceramide. These molecules are powerful stimulators of the serine kinases IκB kinase and c-Jun-aminoterminal kinase cascades.
Activation of the c-Jun-aminoterminal kinase in liver and adipose tissues leads to increased activated protein 1, whereas increased IκB kinase in liver promotes the activation of nuclear factor κB. These inflammatory cascades, as well as inflammation induced by microhypoxic conditions found in obesity, yield the expression of a broad range of cytokines, including tumor necrosis factor–α, monocyte chemoattractant protein–1, chemokine (C-C motif) ligand 2, interleukin-6, and interleukin-1B, which leads to increased expression of CRP in the liver ( Figure 1 ).
Adiponectin levels provide another link between abdominal adiposity and the metabolic syndrome. Adiponectin, produced in adipose tissue, increases in lean individuals and decreases with increasing abdominal adiposity. Adiponectin affects insulin sensitivity by increasing fatty acid oxidation and improving glucose homeostasis. Specifically, adiponectin activates the peroxisome proliferator-activated receptor–α and adenosine monophosphate-activated protein kinase. The activation of peroxisome proliferator-activated receptor–α and monophosphate-activated protein kinase in liver and skeletal muscle yields increased fatty acid oxidation and decreased triglyceride tissue content, which leads to increased insulin sensitivity. Monophosphate-activated protein kinase activity decreases gluconeogenesis in the liver and increases glucose uptake in skeletal muscle, thus improving glucose homeostasis.
Obesity can be assessed by anthropomorphic measures; imaging modalities including computed tomography, magnetic resonance imaging, and dual-energy x-ray absorptiometry; and bioimpedance and skin-fold thickness. Imaging studies offer a direct measure of subcutaneous and visceral adipose tissue, but they are as of yet investigational and not used routinely in clinical practice. Clinical measures of obesity include body mass index (BMI), waist circumference (WC), and waist-to-hip ratio (WHR). BMI is calculated by dividing the weight in kilograms by the height in meters squared. BMI does not provide any information about body fat distribution or differentiate between fat and lean mass.
According to National Institutes of Health guidelines, WC is best measured at the top of the right iliac crest using an inelastic tape measuring parallel to the floor at the end of normal expiration, with obesity being defined as >40 inches in men and >35 inches in women. Hip circumference is measured as the widest point over the buttocks and below the iliac crest. The WHR found by dividing the WC by the hip circumference. There is some evidence that WC has a stronger association with visceral adiposity compared to WHR when measured by direct computed tomographic imaging. A large meta-analysis comparing WC and WHR measures to future CVD events found WHR measures to be less reliable in repeated measurements, likely because of technical problems with the need for disrobing and proper positioning of the measuring tape. There are not yet accurate and reliable methods for measuring bioimpedance and skin-fold thickness that have been broadly applied at the clinical level.
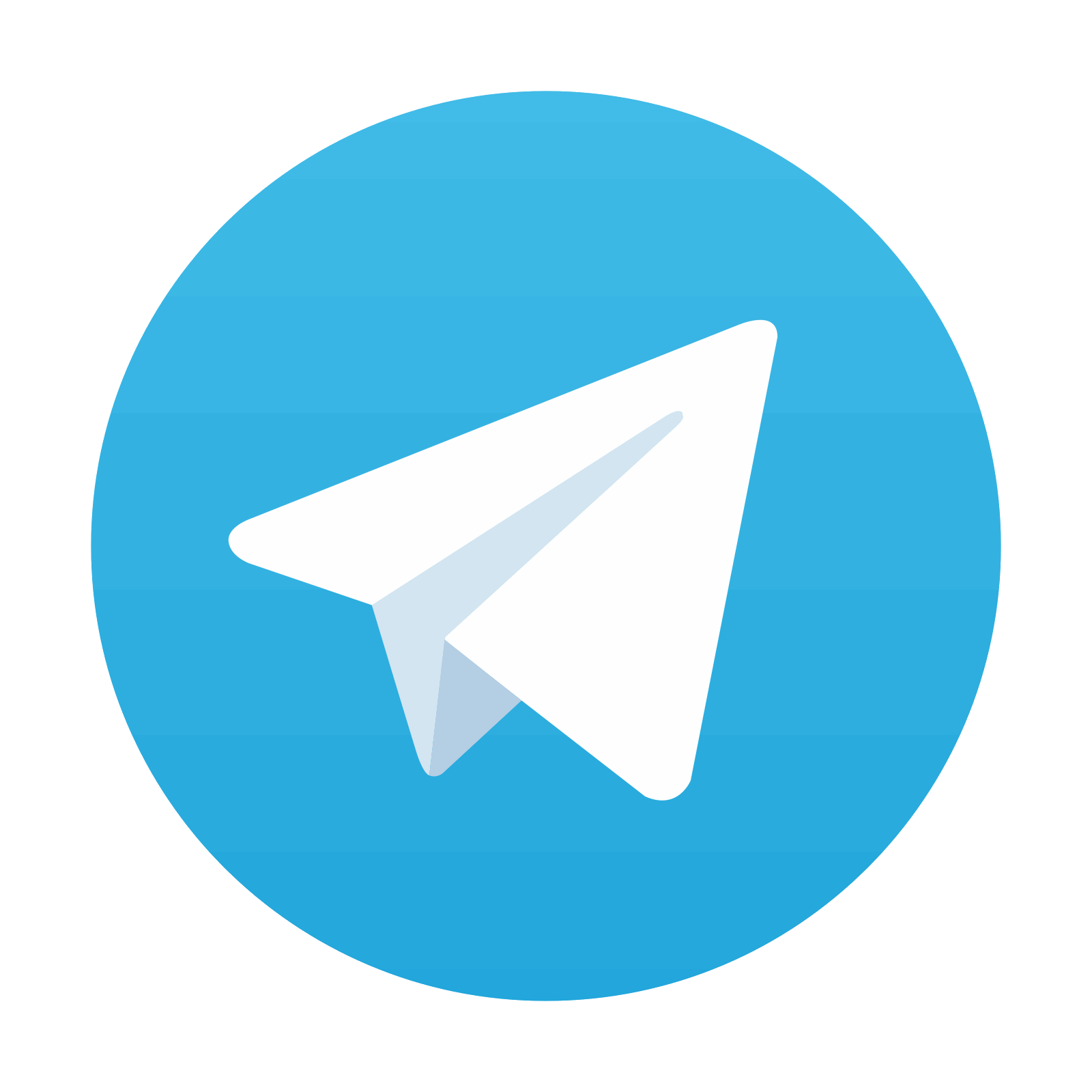
Stay updated, free articles. Join our Telegram channel

Full access? Get Clinical Tree
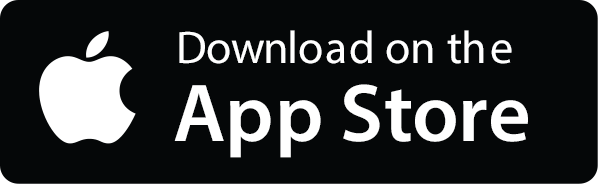
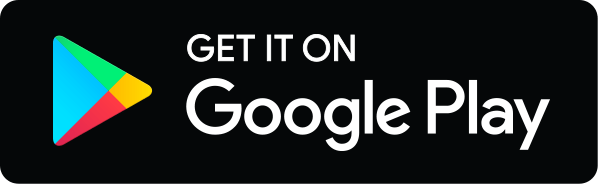