Strain dyssynchrony index (SDI), which was a marker of dyssynchrony and residual myocardial contractility, can predict left ventricular reverse remodeling short-term after cardiac resynchronization therapy (CRT). We investigated SDI-predicted long-term outcome after CRT in patients with heart failure (HF). We studied 74 patients with HF who underwent CRT. SDI was calculated as the average difference between peak and end-systolic strain from 6 segments for radial and circumferential SDIs and 18 segments for longitudinal SDI using 2-dimensional speckle-tracking strain. Based on our previous findings, the predefined cutoff for significant dyssynchrony and residual myocardial contractility was a radial SDI ≥6.5%, a circumferential SDI ≥3.2%, and a longitudinal SDI ≥3.6%. The predefined principal outcome variable was the combined end point of death or hospitalization owing to deteriorating HF. Long-term follow-up after CRT was tracked over 4 years. The primary end point of prespecified events occurred in 14 patients (19%). An association with a favorable long-term outcome after CRT was observed in patients with significant radial, circumferential, and longitudinal SDIs (p <0.001, <0.005, and 0.010 vs patients without significant SDIs, respectively). Furthermore, cardiovascular event-free rate after CRT in patients with positivity of 3 for the 3 SDIs was 100% better than that in patients with positivity of 1 (52%, p <0.005) or 0 (31%, p <0.001) for the 3 SDIs. In conclusion, SDIs can successfully predict long-term outcome after CRT in patients with HF. Moreover, the approach combining the 3 types of SDI leads to a more accurate prediction than the use of individual parameters. These findings may have clinical implications in patients with CRT.
Cardiac response to cardiac resynchronization therapy (CRT) has been defined most commonly in terms of left ventricular (LV) functional response, such as an increase in ejection fraction or a decrease in end-systolic volume at midterm (i.e., 6 months) after CRT. Although LV reverse remodeling at midterm after CRT may be associated with improvements in outcome in many clinical scenarios, it is clear that clinical long-term outcomes are of major importance when determining the benefit of CRT. We previously reported that the strain dyssynchrony index (SDI) obtained with 2-dimensional speckle-tracking strains is coupled with 2 factors, LV dyssynchrony and residual myocardial contractility, and can predict short-term LV reverse remodeling after CRT. Therefore, the objective of our study was to test the hypothesis that baseline SDI is associated with long-term outcome after CRT in patients with advanced heart failure (HF) and that an assessment of combined radial, circumferential, and longitudinal SDIs can further increase the predictive capability of this method for long-term response to CRT.
Methods
This study included 78 consecutive patients with HF and New York Heart Association functional class III or IV despite optimal pharmacologic therapy, ejection fraction ≤35%, and QRS duration ≥120 ms who underwent CRT. Four of these patients (5%) were excluded from all subsequent analyses because of inadequate echocardiographic image quality, so that 74 patients were enrolled in this study ( Table 1 ). Twenty-two patients (30%) had ischemic cardiomyopathy, defined as the presence of ≥75% stenosis in ≥1 major epicardial coronary artery and/or previous coronary revascularization, 51 patients (69%) were diagnosed with sinus rhythm, and 8 (11%) with atrial fibrillation. In addition, 15 patients (23%) previously underwent implantation of a permanent right ventricular pacing ≥1 year before enrollment and featured predominantly right ventricular pacing, which was defined as ≥90% paced when the device was interrogated at time of enrollment. A biventricular pacing system was implanted with a right ventricular apical lead and an LV lead through the coronary sinus in 72 patients. The LV lead was placed in the lateral vein of 31, the posterolateral vein of 30, the anterolateral vein of 9, and the middle vein of 2 patients. An epicardial surgical approach was required for the remaining 2 patients. The atrioventricular interval was adjusted for optimal diastolic filling by Doppler echocardiographic assessment of mitral inflow, and the interventricular interval was adjusted by Doppler echocardiographic assessment of LV outflow 8 ± 2 days after implantation. Written informed consent was obtained from all patients.
Age (years) | 67 ± 12 |
Men/women | 56/18 |
New York Heart Association functional class III/IV | 66/8 |
QRS duration (ms) | 166 ± 26 |
Sinus rhythm/atrial fibrillation/paced | 51/8/15 |
End-diastolic volume (ml) | 170 ± 72 |
End-systolic volume (ml) | 129 ± 64 |
Ejection fraction (%) | 25 ± 7 |
Heart failure cause | |
Ischemic | 22 (30%) |
Nonischemic | 52 (70%) |
Medications | |
Angiotensin-converting enzyme inhibitors/angiotensin receptor blockers | 67 (91%) |
β blockers | 64 (86%) |
Diuretics | 64 (86%) |
Dyssynchrony measurements (ms) | |
Yu index | 46 ± 16 |
Interventricular mechanical delay | 46 ± 25 |
Radial dyssynchrony by speckle-tracking strain | 238 ± 157 |
Strain dyssynchrony index (%) | |
Radial | 8.0 ± 4.5 |
Circumferential | 3.7 ± 1.8 |
Longitudinal | 4.0 ± 1.3 |
All echocardiographic studies were performed with a 3.5-MHz transducer using a commercially available echocardiographic system (Vivid 7; GE Healthcare, Horten, Norway). Digital routine gray-scale 2-dimensional cine loops were obtained, including a mid-LV short-axis view at the level of the papillary muscle and standard apical views (4-chamber, 2-chamber, and long-axis views), and LV and right ventricular outflow tracts were interrogated by pulse-wave Doppler. Sector width was optimized to allow for complete myocardial visualization and to maximize the frame rate. Mean frame rates were 61 ± 7 frames/s in the standard apical view and 64 ± 4 frames/s in the short-axis view for gray-scale imaging used for speckle-tracking analysis. For patients with atrial fibrillation, measurements of standard echocardiographic and speckle-tracking parameters were obtained as averages of ≥10 cardiac cycles. Digital data were transferred to dedicated software (EchoPAC 110.1.3; GE Healthcare) for subsequent off-line analysis. LV end-diastolic volume, end-systolic volume, and ejection fraction were obtained with the modified biplane Simpson’s method.
Routine pulse-wave Doppler was used to determine interventricular dyssynchrony as previously described. Interventricular mechanical delay was determined as the time difference from the onset of right ventricular ejection velocity to that of LV ejection velocity. An interventricular mechanical delay of ≥40 ms was considered to constitute significant dyssynchrony.
Longitudinal dyssynchrony was determined using tissue Doppler cine loops from 3 consecutive beats that were obtained in 3 standard apical views as described elsewhere in detail. Regions of interest (6 × 8 mm) were placed on the basal and mid-LV segments for each of the 3 standard views for a 12-site time-to-peak velocity analysis. Longitudinal tissue Doppler dyssynchrony was determined as the SD of time to peak systolic velocities from the onset of the QRS complex of the 12 sites (Yu index). A longitudinal tissue Doppler dyssynchrony of ≥32 ms was considered to constitute significant dyssynchrony.
For radial dyssynchrony analysis by speckle-tracking strain, routine gray-scale mid-LV short-axis images were used as described elsewhere in detail. Briefly, an end-diastolic circular region of interest was traced on the endocardial cavity with a point-and-click approach. A second larger concentric circle was then automatically generated and manually adjusted near the epicardium. The software automatically divided the mid-LV short-axis and apical images into 6 standard segments and provided the radial, circumferential, and longitudinal speckle-tracking strain curves throughout the cardiac cycle. Radial dyssynchrony by speckle-tracking strain was defined as the time difference from the anteroseptal to the posterior wall segmental peak strains. A radial dyssynchrony of ≥130 ms was considered to constitute significant dyssynchrony.
SDI represents the average of the energy wasted from LV dyssynchrony. Briefly, SDI analysis was performed with speckle-tracking strain using routine gray-scale images. Radial and circumferential SDIs were evaluated from 6 standard segments using mid-LV short-axis views. Longitudinal SDI was assessed at basal, mid, and apical levels in apical 4-chamber, 2-chamber, and long-axis views, producing 18 sites ( Figure 1 ) . Peak of the Q wave on electrocardiogram was used as the reference time point for end-diastole. Timing of the aortic valve closure (AVC), defined as the end of the LV outflow tract as detected by pulse-wave Doppler, was used as the reference time point for end-systole. Energy wasted per segment because of dyssynchrony was expressed as the difference between peak strain and AVC strain ( Figure 1 ). This difference increases as the degree of dyssynchrony increases because an increase in dyssynchrony leads to a decrease in AVC strain. SDI was then calculated as the average of the absolute difference between peak strain and AVC strain from 6 segments for radial and circumferential SDIs and 18 segments for longitudinal SDI. Three consecutive cardiac cycles were recorded and averaged for each measurement. If a segment showed a negative radial strain or a positive circumferential and longitudinal strain during the entire cardiac cycle, the difference between peak strain and AVC strain was assumed to be 0. Based on our previous observations of a group of patients undergoing CRT, our predefined cut-off values of radial SDI ≥6.5%, circumferential SDI ≥3.2%, and longitudinal SDI ≥3.6% were considered significant for predicting a response to CRT.
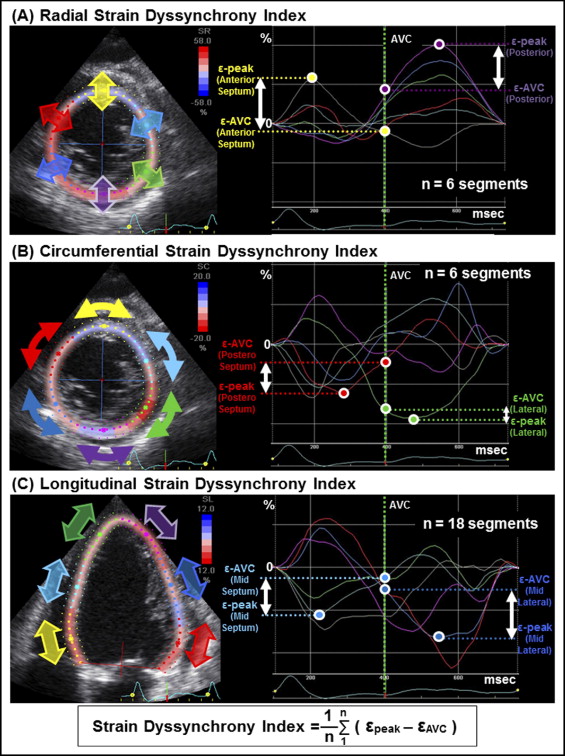
Long-term unfavorable outcome events were prespecified as primary end points of death or hospitalization from deteriorating HF. Long-term follow-up after CRT was tracked over 4 years.
Continuous variables were expressed as mean ± SD. Receiver operating characteristics curves were constructed for each parameter individually to test predefined cutoffs and to determine sensitivities and specificities. Areas under the curve based on receiver operating characteristics analysis were compared by logistic regression analysis. Event-free survival curves were determined with the Kaplan–Meier method and comparisons of cumulative event rates with log-rank test. When any potential influence of covariates was suspected, a Cox proportional hazard model was used. The intraclass correlation coefficient was then used to determine inter- and intraobserver reproducibilities from 10 randomly selected patients. For all tests a p value <0.05 was considered statistically significant. All analyses were performed with commercially available software (MedCalc 10.4.0.0, MedCalc Software, Inc., Mariakerke, Belgium). The authors had full access to the data and took full responsibility for its integrity. All authors have read and agreed to the report as written.
Results
Fourteen patients (19%) reached the primary end point of a prespecified clinical event; 4 died from deteriorating HF and 10 were hospitalized for worsening HF over 4 years. Radial SDI ≥6.5% predicted the probability of event-free survival with 70% sensitivity, 79% specificity, and an area under the curve equal to 0.79 ( Figure 2 ) . Circumferential SDI ≥3.2% or longitudinal SDI ≥3.6% also predicted the probability of event-free survival with 63% or 65% sensitivity, 79% or 71% specificity, and areas under the curve equal to 0.73 or 0.69, respectively. The difference in predicting event-free survival among these 3 types of SDIs was not statistically significant. Of conventional dyssynchrony measurements, only radial dyssynchrony by speckle-tracking strain ≥130 ms predicted the probability with statistical significance. Interventricular mechanical delay and the Yu index failed to predict the probability of event-free survival.
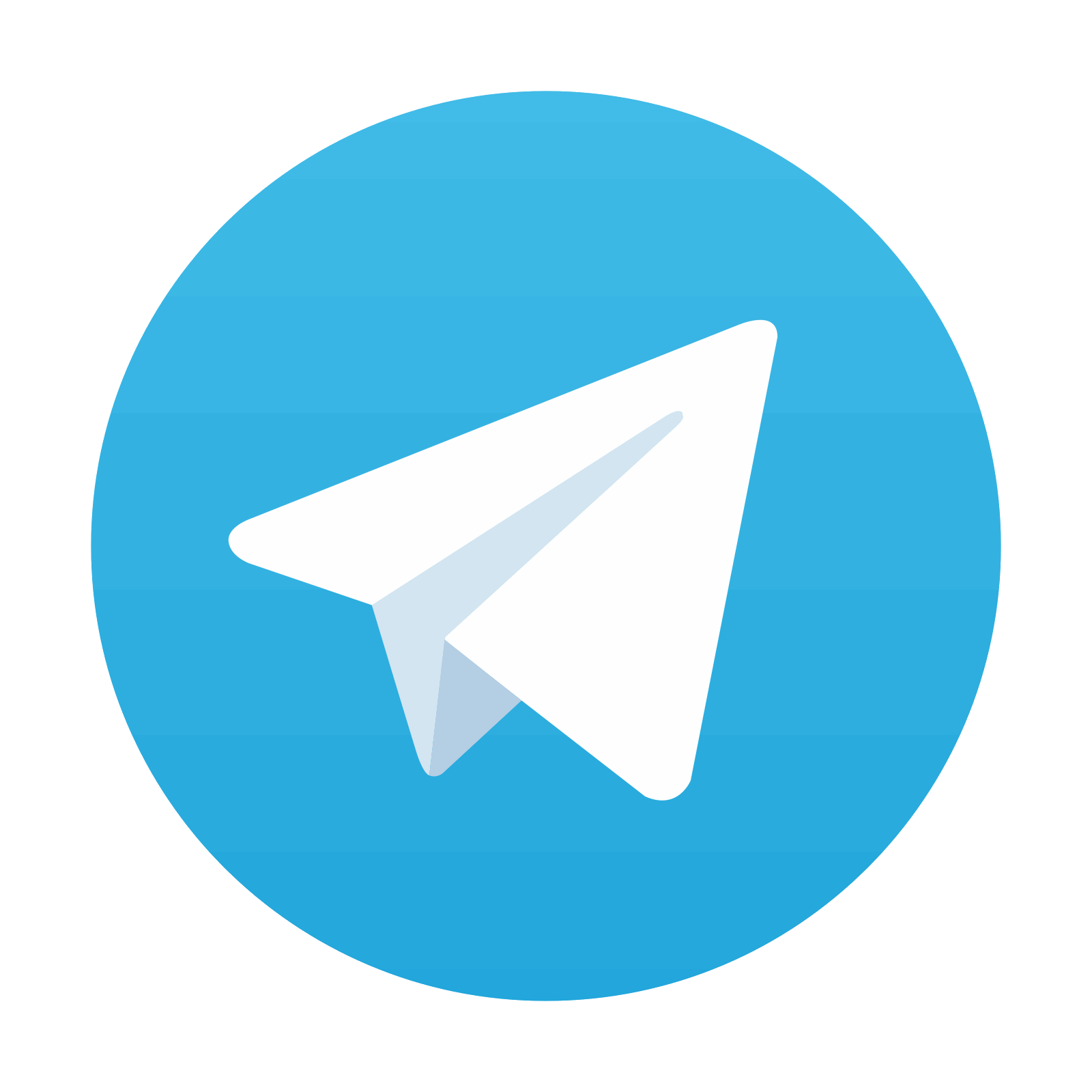
Stay updated, free articles. Join our Telegram channel

Full access? Get Clinical Tree
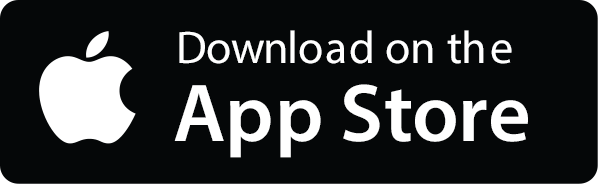
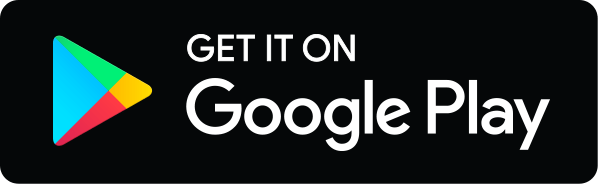
