Digital peripheral arterial tonometry (PAT) is an emerging, noninvasive method to assess vascular function. The physiology underlying this phenotype, however, remains unclear. Therefore, we evaluated the relation between digital PAT and established brachial artery ultrasound measures of vascular function under basal conditions and after reactive hyperemia. Using a cross-sectional study design, digital PAT and brachial artery ultrasonography with pulsed wave Doppler were simultaneously completed at baseline and after reactive hyperemia in both those with established coronary artery disease (n = 99) and healthy volunteers with low cardiovascular disease risk (n = 40). Under basal conditions, the digital pulse volume amplitude demonstrated a significant positive correlation with the brachial artery velocity-time integral that was independent of the arterial diameter, in both the healthy volunteer (r s = 0.64, p <0.001) and coronary artery disease (r s = 0.63, p <0.001) cohorts. Similar positive relations were observed with the baseline brachial artery blood flow velocity and blood flow. In contrast, no relation between the reactive hyperemia-evoked digital PAT ratio and either brachial artery flow-mediated dilation or shear stress was observed in either cohort (p = NS). In conclusion, these findings demonstrate that the digital PAT measures of vascular function more closely reflect basal blood flow in the brachial artery than reactive hyperemia-induced changes in the arterial diameter or flow velocity, and the presence of vascular disease does not modify the physiology underlying the digital PAT phenotype.
Endothelial dysfunction is integral to the pathogenesis and progression of coronary artery disease (CAD). Brachial artery flow-mediated dilation (FMD) is the most widely used noninvasive method to assess endothelial function; however, the technical complexity and lack of standardization limit its clinical application. Digital peripheral arterial tonometry (PAT) quantifies the reactive hyperemia-induced changes in pulse volume amplitude (PVA) in the fingertip and is an emerging, automated method to noninvasively assess endothelial function. Digital PAT is predictive of the presence of cardiovascular disease risk factors, impaired vasodilator responses to acetylcholine in coronary arteries, and adverse cardiovascular events in a single study. Although previous studies have demonstrated a direct contribution of nitric oxide to the brachial artery FMD and the digital PAT ratio, conflicting results regarding their correlation have been reported. Despite growing interest in the utility of digital PAT, the physiology underlying the digital PVA signal and its relation with established vascular function phenotypes remains unclear. In particular, the relation between digital PVA and brachial artery blood flow velocity, and the effect of vascular disease on these phenotypes, has not been rigorously evaluated. Therefore, we characterized the relation between digital PAT and brachial artery ultrasound vascular function phenotypes, under both basal and reactive hyperemia-evoked conditions, in healthy volunteers (HVs) and patients with established CAD.
Methods
Patients with established CAD (defined as ≥50% stenosis in ≥1 major epicardial coronary artery by coronary angiography) were identified in the University of North Carolina Cardiac Catheterization Laboratory (Chapel Hill, North Carolina). The exclusion criteria included pregnancy, atrial fibrillation, Raynaud’s disease, left ventricular ejection fraction ≤35%, the current use of insulin or long-acting nitrates, active autoimmune disease, history of severe aortic stenosis, history of solid organ transplant or dialysis, or a history of cancer within the previous 5 years.
A parallel cohort of HVs was identified by advertisement from the local Chapel Hill, North Carolina community. After a detailed history and a fasting serum chemistry and cholesterol panel, the potential participants were excluded if they had a history of cardiovascular disease, had risk factors for CAD (including physician-diagnosed hypertension or diabetes, cigarette smoking within the previous 6 months, high cholesterol [defined as total cholesterol >240 mg/dl, triglycerides >200 mg/dl, or low-density lipoprotein cholesterol >160 mg/dl), or body mass index >30 kg/m 2 ), or were currently taking any medication for a chronic medical condition.
The University of North Carolina at Chapel Hill Biomedical Institutional Review Board approved the study protocol. Eligible participants (108 with CAD and 41 HVs) provided written informed consent and returned to the clinical research unit for a single morning study visit after fasting overnight and withholding their morning medications. The participants were instructed to refrain from tobacco products, caffeine, and vigorous exercise the morning of the study visit and from the use of vitamin C, vitamin E, fish oil, niacin, or arginine supplements, oral decongestants, nonsteroidal anti-inflammatory drugs (other than low-dose aspirin), and erectile dysfunction medications for ≥7 days before the study visit. Those who experienced a respiratory tract infection within 4 weeks before the study visit were not eligible to participate.
The endothelial function measurements were completed in a quiet, dimly lit, temperature-controlled room and were obtained with the participant in the supine position. After 10 minutes of rest, brachial artery reactivity was assessed by quantifying the arterial diameter and blood flow velocity at baseline and after reactive hyperemia using a 12.5-MHz linear-array transducer (Philips HDI 5000 system) with pulsed wave Doppler, as previously described. Reactive hyperemia was induced by inflating a blood pressure cuff around the right forearm for 5 minutes to a pressure of ≥70 mm Hg greater than the systolic blood pressure. All images were electrocardiogram gated and obtained by the same sonographer throughout the study. The data were analyzed using Brachial Tools software (Medical Imaging Applications, Coralville, Iowa).
The brachial artery diameter was measured at end-diastole from the lumen–intimal interface of the proximal and distal walls. The baseline diameter was averaged from 10 consecutive frames. The arterial diameter was assessed for 90 seconds after cuff deflation, and the diameter during peak dilation was averaged from 3 consecutive frames. The peak change in arterial dilation was observed a mean ± SD of 51 ± 12 seconds after cuff deflation. The brachial artery FMD (endothelium-dependent) was calculated as the percentage of change in brachial artery diameter from baseline [brachial artery FMD = 100 × (diameter Peak − diameter Baseline )/(diameter Baseline )]. The reproducibility of this method in our laboratory has been previously reported. After 10 minutes of rest, a second baseline image was acquired, which correlated highly with the initial baseline measurement (r = 0.99, p <0.001). Endothelial-independent vasodilation was assessed 5 minutes after sublingual administration of nitroglycerin (0.4 mg) as the percentage of change in the arterial diameter [100 × (diameter Nitroglycerin − diameter Baseline )/(diameter Baseline )].
The brachial artery blood flow velocity was measured using pulsed wave Doppler at baseline and for 15 seconds after cuff deflation. The velocity-time integral was measured by automated planimetry of the velocity profile and averaged for 3 consecutive cardiac cycles at baseline and during peak reactive hyperemia. The reactive hyperemia-induced increase in the velocity-time integral relative to baseline was calculated as a ratio (velocity-time integral following reactive hyperemia/velocity-time integral at baseline). The mean flow velocity (V) was calculated by dividing the velocity-time integral (in cm) by the duration of the cycle (in seconds). The mean flow velocity was converted to local shear stress (8 × μ × V x /diameter Baseline ) and blood flow [V x × π × (diameter Baseline /2) 2 ], as previously reported, each of which normalize the flow velocity to the arterial diameter. The subscript x indicates either baseline or peak reactive hyperemia, and μ indicates blood viscosity at an assumed value of 0.035 dyne · s/cm 2 . Because the reactive hyperemia-induced flow velocity changes in the conduit arteries are regulated by dilation of the distal resistance vessels, brachial shear stress after reactive hyperemia is as an index of microvascular function.
The digital PVA was measured on the index finger of each hand using plethysmographically based probes and the Endo-PAT 2000 device (Itamar Medical, Caesarea, Israel) simultaneously with the brachial artery ultrasound measurements. The measurements were obtained continuously during baseline (5 minutes), cuff occlusion (5 minutes), and postcuff deflation (5 minutes), and the data were automatically derived using the Endo-PAT, version 3.0.4, software. The digital PAT ratio was calculated as the ratio of postcuff deflation PVA (in 30-second intervals) to the baseline PVA in the arm undergoing cuff occlusion (occluded) and then normalized to the contralateral (control) arm. The digital PAT ratio in the 90- to 120-second postcuff deflation interval [(PVA occluded,90–120seconds /PVA occluded,Baseline )/(PVA control,90–120seconds /PVA control,Baseline )] is presented, as described previously, unless otherwise noted. The digital PAT test was unsuccessful in 10 study participants (9 with CAD and 1 HV), consistent with previous reports. The reproducibility of this automated method has been previously established.
The data are presented as the mean ± SD or the median (interquartile range). The study population characteristics were compared using 1-way analysis of variance or Wilcoxon rank sum test, as appropriate. The relations between each digital PAT and brachial artery ultrasound phenotype were evaluated using Spearman’s rank correlation (r s ). Partial correlations were also evaluated to account for the potential effect of the demographic (age, gender, race) and vascular (baseline brachial artery diameter, baseline brachial artery velocity-time integral, baseline digital PVA, brachial shear stress after reactive hyperemia) variables on these relations. The parameters that were not normally distributed, including the digital PVA and PAT ratio, were log 10 -transformed before graphic presentation. Because the units of each digital PAT and brachial artery ultrasound measure were not the same, Bland-Altman plots could not be constructed to evaluate the agreement between each phenotype. The analyses were performed using SAS, version 9.2 (SAS Institute, Cary, North Carolina). Statistical significance was set at p <0.05.
Results
The cohort characteristics are provided in Table 1 . The participants in the CAD cohort returned for their study visit 61 ± 33 days after cardiac catheterization. High rates of statin, renin-angiotensin system inhibitor, β-blocker, aspirin, and clopidogrel use were reported, consistent with management of the CAD cohort according to current clinical practice guidelines.
Characteristic | CAD (n = 99) | HV (n = 40) |
---|---|---|
Age (years) | 59 ± 9 | 51 ± 8 ⁎ |
Women | 33 (33%) | 23 (58%) ⁎ |
Black race | 18 (18%) | 7 (18%) |
Body mass index (kg/m 2 ) | 30 ± 6 | 26 ± 3 ⁎ |
Body mass index ≥30 kg/m 2 | 53 (54%) | 0 † |
Current smoker | 23 (23%) | 0 † |
Diabetes | 24 (24%) | 0 † |
Hypertension | 82 (83%) | 0 † |
Disease severity | ||
One-vessel disease | 35 (35%) | — |
Two-vessel disease | 36 (36%) | — |
Three-vessel disease | 28 (28%) | — |
Revascularization procedure ‡ | 65 (66%) | — |
Systolic blood pressure (mm Hg) | 136 ± 17 | 122 ± 14 ⁎ |
Diastolic blood pressure (mm Hg) | 80 ± 10 | 74 ± 8 ⁎ |
Total cholesterol (mg/dl) | 159 (49) | 192 (30) ⁎ |
Low-density lipoprotein cholesterol (mg/dl) | 85 (41) | 117 (30) ⁎ |
High-density lipoprotein cholesterol (mg/dl) | 48 (17) | 65 (23) ⁎ |
Triglycerides (mg/dl) | 97 (79) | 69 (32) ⁎ |
Total/high-density lipoprotein cholesterol | 3.4 (1.6) | 3.1 (1.4) |
High-sensitivity C-reactive protein (mg/L) | 1.6 (3.3) | 0.7 (1.7) ⁎ |
Statin use | 92 (93%) | 0 † |
Renin-angiotensin system inhibitor use § | 61 (62%) | 0 † |
β-Blocker use | 82 (83%) | 0 † |
Aspirin use | 96 (97%) | 0 † |
Clopidogrel use | 79 (80%) | 0 † |
⁎ p <0.05 versus coronary artery disease.
† Exclusion criteria in HV cohort.
‡ In CAD cohort, 55 of 99 underwent percutaneous coronary intervention and 10 of 99 underwent coronary artery bypass grafting between screening and the study visit.
§ Either angiotensin-converting enzyme inhibitor or angiotensin receptor blocker.
Under basal conditions, the digital PVA and brachial artery diameter correlated modestly in both cohorts ( Figure 1 ); however, these correlations were not statistically significant when adjusting for age, gender, and race (HVs, r s = 0.21, p = 0.208; CAD group, r s = 0.12, p = 0.260). The baseline digital PVA demonstrated a significant positive correlation with basal brachial artery velocity-time integral that was similar in both cohorts ( Figure 1 ). After stratifying by gender, significant relations were observed in women (HVs, r s = 0.80, p <0.001; CAD group, r s = 0.65, p <0.001) and men (HVs, r s = 0.55, p = 0.023; CAD group, r s = 0.66, p <0.001). Similar relations were also observed after adjusting for age, gender, race, and brachial artery diameter (HVs, r s = 0.71, p <0.001; CAD group, r s = 0.72, p <0.001), and when the data were expressed as the mean flow velocity (HVs, r s = 0.56, p <0.001; CAD group, r s = 0.62, p <0.001), blood flow (HVs, r s = 0.78, p <0.001; CAD group, r s = 0.72, p <0.001), or shear stress ( Figure 1 ), further demonstrating a positive relation between the basal digital PVA and brachial artery blood flow velocity that is independent of arterial size.
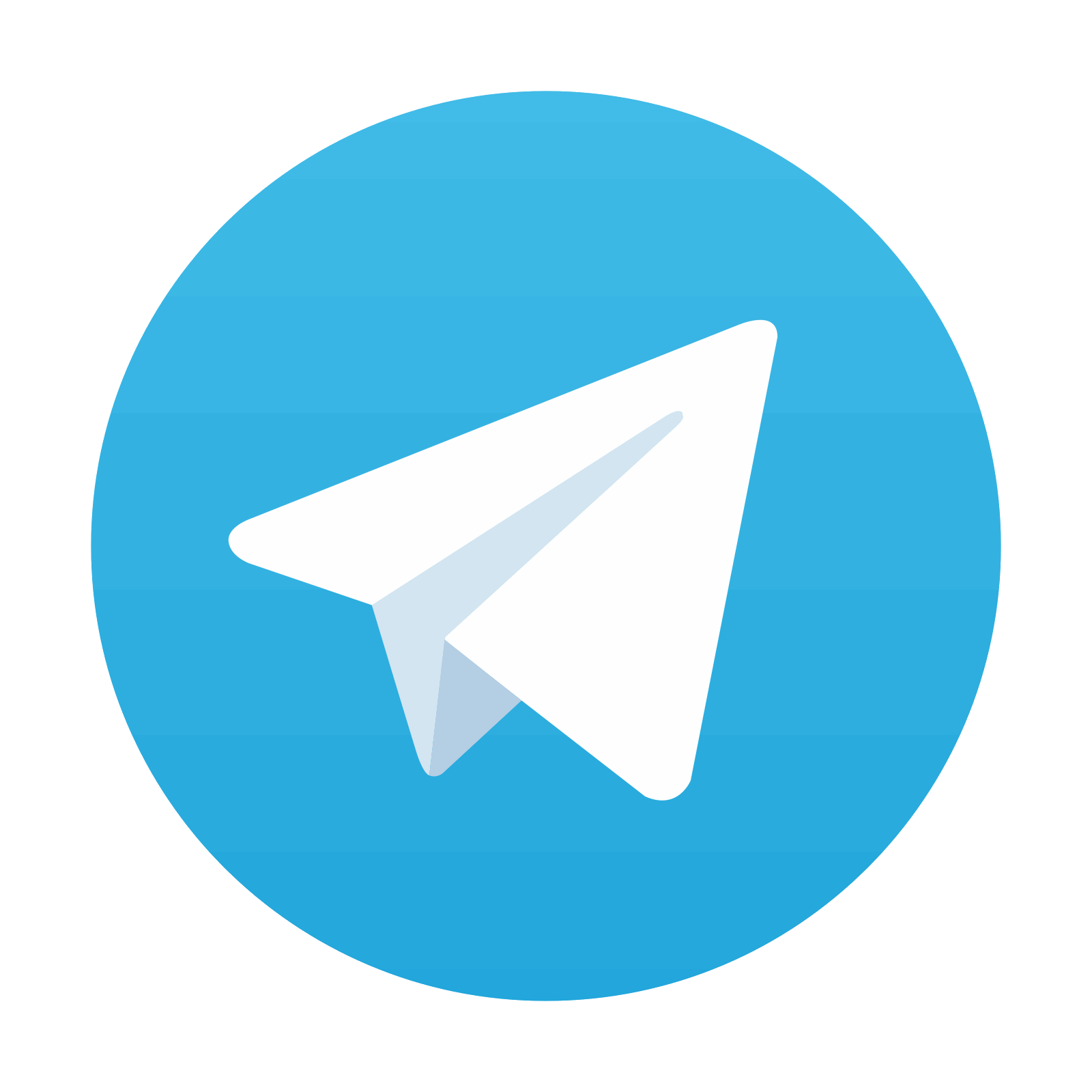
Stay updated, free articles. Join our Telegram channel

Full access? Get Clinical Tree
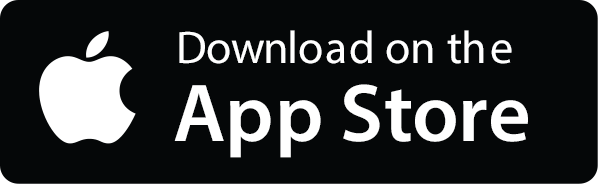
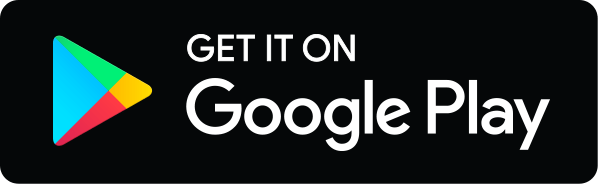
