Previous angiographic studies have suggested that the future risk for major adverse cardiovascular events (MACEs) is related to coronary stenosis severity. The aim of this study was to use the grayscale and virtual histology (VH)–intravascular ultrasound (IVUS) data from the Providing Regional Observations to Study Predictors of Events in the Coronary Tree (PROSPECT) study to identify underlying lesion morphologic characteristics that might explain these findings. In PROSPECT, patients presenting with acute coronary syndromes in whom percutaneous coronary intervention was successful underwent 3-vessel grayscale and VH-IVUS and were followed for a median of 3.4 years for the incidence of MACEs. Overall, 3,115 nonculprit lesions detected by IVUS were divided into quartiles according to baseline angiographic diameter stenosis. From the first to fourth quartiles, there were increases in the prevalence of lesions with IVUS minimum luminal areas ≤4 mm 2 , IVUS plaque burden ≥70%, and VH-IVUS thin-cap fibroatheroma (13.4%, 22.0%, 24.2%, and 30.3%, respectively, p <0.001), along with an increased frequency of plaque ruptures and greater necrotic core volumes. The incidence of lesions with plaque burden ≥70%, minimum luminal area ≤4 mm 2 , and VH thin-cap fibroatheroma was highest in the fourth quartile (0%, 0.4%, 0.4%, and 2.8% in the first through fourth quartiles, respectively, p <0.001). Three-year MACE rates were also highest in the fourth quartile (0.3%, 0.7%, 1.3%, and 5.1%, respectively, p <0.001). In conclusion, increasing angiographic diameter stenosis was associated with an increased frequency of grayscale and VH-IVUS lesion morphologic features that have been associated with adverse events and that may, in part, explain why future MACEs were related to baseline lesion severity.
Virtual histology (VH)–intravascular ultrasound (IVUS) correlates well with histopathology and potentially classified plaques into phenotypical subtypes. Patients enrolled in the Providing Regional Observations to Study Predictors of Events in the Coronary Tree (PROSPECT) study underwent 3-vessel coronary angiography and grayscale and VH-IVUS after culprit lesions were successfully treated. Although nonculprit lesions that subsequently resulted in future adverse cardiac events showed angiographically mild stenosis, by multivariate analysis, the independent predictors of nonculprit lesion–related events at 3 years of follow-up were the IVUS parameters of plaque burden >70%, minimum luminal area (MLA) <4 mm 2 , and VH-IVUS thin-cap fibroatheroma (VH-TCFA). In the present study, we compared residual nonculprit angiographic stenosis severity to grayscale and VH-IVUS lesion morphology. Our hypothesis was that residual nonculprit lesions with increasing angiographic diameter stenoses would have larger plaque burdens, smaller MLAs, and more VH-TCFAs and would be associated with a higher rate of future major adverse cardiac events (MACEs).
Methods
The PROSPECT study design, major inclusion and exclusion criteria, end points, and definitions have been previously described in detail. In brief, 697 patients with acute coronary syndromes (ST-segment elevation myocardial infarction >24 hours, non–ST-segment elevation myocardial infarction, or unstable angina) underwent angiography and multimodality intracoronary imaging of the proximal 6 to 8 cm of all 3 coronary arteries after the performance of successful percutaneous coronary intervention of all coronary lesions responsible for the index event (culprit lesions) and other severe angiographic stenoses. Of the 697 patients enrolled in PROSPECT, angiographic and IVUS data were available in 659 patients, thus constituting the study population. Intracoronary imaging, grayscale and VH-IVUS, was obtained using a synthetic-aperture-array, 20-MHz, 3.2Fr catheter (Eagle Eye, In-Vision Gold; Volcano Corporation, Rancho Cordova, California) with motorized catheter pullback (0.5 mm/s). Patients were then followed for a median of 3.4 years to relate subsequent events to the morphologies of the lesions detected at baseline. The study was approved by the institutional review board or medical ethics committee at each participating center, and all patients gave written informed consent.
Baseline and follow-up angiograms and baseline grayscale and VH-IVUS studies were analyzed at separate core laboratories that were blinded to clinical outcomes (Cardiovascular Research Foundation, New York, New York). Quantitative coronary angiography was performed using Medis CMS software version 7.0 (Medis Medical Imaging, Leiden, The Netherlands). All culprit and nonculprit lesions (defined as ≥30% angiographic visual angiographic diameter stenosis) were identified and located in relation to the corresponding coronary artery ostium and nearby side branches. All 3 epicardial vessels as well as all side branches ≥1.5 mm in diameter were divided into 29 Coronary Artery Surgery Study (CASS) segments. Each CASS segment was then subdivided into subsegments 1.5 mm in length and analyzed. Measurements of each 1.5-mm subsegment included minimum luminal diameter, reference vessel diameter, and diameter stenosis.
Grayscale and VH-IVUS analyses were performed using QCU-CMS software (Medis Medical Imaging), pcVH version 2.1 software (Volcano Corporation), and proprietary qVH software (Cardiovascular Research Foundation). The external elastic membrane and luminal borders were contoured for each frame. Quantitative grayscale IVUS measurements included the cross-sectional areas of the external elastic membrane, lumen, and plaque and media (external elastic membrane minus lumen) and plaque burden (plaque and media divided by external elastic membrane). The remodeling index was calculated as the external elastic membrane cross-sectional area at the MLA slice divided by the average of the proximal and distal reference external elastic membrane cross-sectional area. Qualitative grayscale IVUS morphology included plaque rupture (intraplaque cavity that communicated with the lumen with an overlying residual fibrous cap fragment) and echolucent plaque (a plaque containing a non-echo-reflective dark zone). An IVUS nonculprit lesion was defined by a plaque burden of >40% in ≥3 consecutive frames. Lesions were considered separate if there was a ≥5-mm-long segment with <40% plaque burden between them. On the basis of VH-IVUS, plaque components were categorized as dense calcium, fibrous tissue, fibrofatty plaque, or necrotic core (NC) and reported percentages of total plaque areas and volumes. Lesions were then classified by VH-IVUS as 1 of the following: VH-TCFA, thick-cap fibroatheroma (ThCFA), pathologic intimal thickening, fibrotic plaque, or fibrocalcific plaque, as previously reported. Fibrotic plaque had mainly fibrous tissue with <10% confluent NC, <10% confluent dense calcium, and <15% fibrofattty plaque. Fibrocalcific plaque had mainly fibrous tissue with >10% confluent dense calcium but <10% confluent NC. Pathologic intimal thickening was a mixture of all plaque components, but dominantly fibrofatty plaque with <10% confluent NC and <10% confluent dense calcium. Fibroatheroma (VH-TCFA and ThCFA) was defined as >10% confluent NC (spotty red color was not considered as confluent NC). Because the resolution of VH-IVUS is 150 to 250 μm, it was not possible to detect fibrous cap thickness <65 μm (the typical pathologic definition of a a thin fibrous cap). Therefore, if there was >30° of NC abutting to the lumen in 3 consecutive slices, the fibroatheroma was classified as VH-TCFA; otherwise, it was classified as ThCFA. Fibroatheromas were considered multiple and distinct if they were separated by ≥3 consecutive slices containing a different fibroatheroma subtype (VH-TCFA or ThCFA) or nonfibroatheroma phenotype, and the length of each fibroatheroma was analyzed and summed per lesion. The total NC abutting the lumen was measured in degrees and analyzed at the maximum arc of NC abutting to the lumen within each VH-TCFA. Fibroatheromas were subclassified as having single or multiple confluent NCs. Each IVUS lesion was coregistered to the angiographic road map using fiduciary branches for alignment as previously described.
The primary end point was the incidence of MACEs (the composite of death from cardiac causes, cardiac arrest, myocardial infarction, or rehospitalization for unstable or progressive angina according to the Braunwald unstable angina classification and the Canadian Cardiovascular Society angina classification). The primary end point was adjudicated by an independent clinical events committee. On the basis of follow-up angiography, MACEs were attributed to a nonculprit lesion site if the site associated with an event was previously untreated. If follow-up angiography was not performed, the lesion site was classified as indeterminate and excluded from this analysis.
All statistical analyses were performed using SAS version 9.2 (SAS Institute Inc., Cary, North Carolina). Categorical variables were summarized using percentages and counts and were compared using chi-square tests or Fischer’s exact tests as appropriate. Lesions were divided into quartiles on the basis of the angiographic diameter stenosis. For fibroatheroma- and lesion-level data, a model with a generalized estimating equation approach was used to compensate for potential cluster effects of multiple lesions in the same patient and presented as least square means with 95% confidential intervals. Continuous variables were compared using analysis of variance and unpaired Student’s t tests. Time-to-event data are presented as Kaplan-Meier estimates and were compared using generalized estimating equation adjusted log-rank tests. A p value <0.05 was considered statistically significant.
Results
Patient characteristics are described in Table 1 . The median patient age was 58.1 years, 17.2% of the patients had diabetes mellitus, and 66.2% of the patients presented with non-ST-segment elevation myocardial infarctions and 30.0% with ST-segment elevation myocardial infarctions.
Characteristic | Value |
---|---|
Age (years) | 58.1 (50.7–66.7) |
Men | 507/659 (76.9%) |
Diabetes mellitus | 113/656 (17.2%) |
Hypertension | 305/653 (46.7%) |
Current smokers | 310/650 (47.7%) |
Previous myocardial infarction | 70/655 (10.7%) |
Clinical presentation | |
ST-segment elevation myocardial infarction | 198/659 (30.0%) |
Non–ST-segment elevation myocardial infarction | 436/659 (66.2%) |
Unstable angina pectoris | 25/659 (3.8%) |
Total cholesterol (mg/dl) | 172.0 (149.5–200.0) |
Low-density lipoprotein cholesterol (mg/dl) | 100.8 (79.2–127.4) |
High-density lipoprotein cholesterol (mg/dl) | 38.6 (34.0–46.0) |
Triglycerides (mg/dl) | 125.0 (88.6–177.1) |
Estimated creatinine clearance ≤60 ml/min | 61/622 (9.8%) |
High-sensitivity C-reactive protein (mg/l), day 0 | 7.3 (2.4–19.0) |
Worst nonculprit lesion on quantitative coronary angiography (%) | 46.2 (37.6–57.9) |
IVUS nonculprit lesion per patient | 5.0 (4.0–6.0) |
By quantitative angiographic analysis, the median diameter stenosis of the worst untreated nonculprit lesion in each patient was 46.2% (interquartile range 37.6% to 57.9%, range 1.1% to 96.0%), and 41.6% of patients had ≥1 lesion with a >50% diameter stenosis. When lesions were divided into quartiles on the basis of the lesion with the worst diameter stenosis, the least square means angiographic diameter stenoses among the 4 quartiles were 2.8%, 10.0%, 17.7%, and 33.5% in the first through fourth quartiles, respectively (p <0.001; Table 2 ). The incidence of impaired renal function (estimated creatinine clearance ≤60 ml/min) was significantly higher in the fourth quartile than in the other 3 quartiles (6.5%, 6.3%, 9.8%, and 16.7% in the first through fourth quartiles, respectively, p = 0.006). However, other characteristics such as age, incidence of diabetes, and level of low-density lipoprotein cholesterol were similar among the 4 patient groups (data not shown).
Variable | Quartile 1 (n = 778) | Quartile 2 (n = 779) | Quartile 3 (n = 779) | Quartile 4 (n = 779) | p Value |
---|---|---|---|---|---|
Diameter stenosis (%) | 2.8 (2.6–3.1) | 10.0 (9.8–10.1) | 17.7 (17.5–17.9) | 33.5 (32.9–34.1) | <0.001 ⁎ |
Reference vessel diameter (mm) | 3.25 (3.20–3.30) | 3.07 (3.02–3.13) | 3.01 (2.96–3.06) | 3.00 (2.95–3.06) | <0.001 ⁎ |
Minimal luminal diameter (mm) | 3.15 (3.10–3.21) | 2.76 (2.72–2.81) | 2.47 (2.43–2.51) | 2.01 (1.97–2.05) | <0.001 ⁎ |
Overall, there were 3,115 nonculprit lesions detected by IVUS. The IVUS MLA decreased across the first to the fourth quartiles of the quantitative angiographic diameter stenosis; thus, the frequency of lesions with MLAs ≤4 mm 2 was highest in the fourth angiographic quartile (10.0%, 11.9%, 20.2%, and 34.8% in the first through fourth quartiles, respectively, p <0.001; Table 3 ). The magnitude of plaque burden at the MLA site (61.6% in the fourth quartile) and the prevalence of lesions with plaque burden ≥70% at the MLA site were also highest in the fourth angiographic quartile (1.7%, 3.6%, 9.9%, and 20.5% in the first through fourth quartiles, respectively, p <0.001). The frequency of echolucent plaques and plaque ruptures also increased from the first through the fourth angiographic quartiles ( Table 3 ).
Variable | Quartile 1 (n = 778) | Quartile 2 (n = 779) | Quartile 3 (n = 779) | Quartile 4 (n = 779) | p Value |
---|---|---|---|---|---|
Volumetric analysis | |||||
Average EEM area (mm 3 /mm) | 17.5 (17.0–18.0) | 16.5 (16.0–16.9) | 15.8 (15.4–16.2) | 15.5 (15.1–15.9) | <0.001 ⁎ |
Average luminal area (mm 3 /mm) | 9.4 (9.1–9.7) | 8.7 (8.4–8.9) | 8.0 (7.8–8.3) | 7.5 (7.3–7.7) | <0.001 ⁎ |
Average P&M area (mm 3 /mm) | 8.1 (7.9–8.3) | 7.8 (7.6–8.0) | 7.8 (7.5–8.0) | 7.9 (7.7–8.2) | 0.11 ⁎ |
Plaque burden (%) | 46.2 (45.8–46.6) | 47.4 (47.0–47.8) | 49.0 (48.6–49.4) | 51.1 (50.6–51.5) | <0.001 ⁎ |
Lesion length (mm) | 7.6 (7.2–8.1) | 13.8 (13.0–14.6) | 18.7 (17.6–19.7) | 23.6 (22.4–24.8) | <0.001 ⁎ |
MLA site analysis | |||||
EEM area (mm 2 ) | 16.5 (16.1–17.0) | 15.2 (14.8–15.6) | 14.4 (14.0–14.8) | 13.9 (13.5–14.3) | <0.001 ⁎ |
MLA (mm 2 ) | 7.9 (7.7–8.1) | 6.9 (6.7–7.1) | 6.1 (5.9–6.2) | 5.2 (5.0–5.4) | <0.001 ⁎ |
MLA ≤4 mm 2 | 78 (10.0%) | 93 (11.9%) | 157 (20.2%) | 271 (34.8%) | <0.001 † |
Plaque burden (%) | 52.1 (51.5–52.6) | 54.5 (53.9–55.1) | 57.3 (56.7–58.0) | 61.6 (60.1–62.3) | <0.001 ⁎ |
Plaque burden ≥70% | 13 (1.7%) | 28 (3.6%) | 77 (9.9%) | 160 (20.5%) | <0.001 † |
Remodeling index | 0.95 (0.94–0.96) | 0.92 (0.91–0.93) | 0.91 (0.90–0.92) | 0.89 (0.88–0.90) | <0.001 ⁎ |
Morphologic analysis | |||||
Echolucent plaque | 3 (0.4%) | 16 (2.1%) | 30 (3.9%) | 78 (10.0%) | <0.001 † |
Plaque rupture | 6 (0.8%) | 11 (1.4%) | 26 (3.3%) | 58 (7.4%) | <0.001 † |
Overall, VH-IVUS data were available for 2,780 of the 3,115 IVUS lesions. VH-IVUS volumetric analysis showed that percentage NC volume and percentage dense calcium volume increased significantly from the first to the fourth angiographic quartile, while percentage fibrofatty volume decreased from the first to the fourth quartile (all p values <0.001). MLA site analysis also showed similar results ( Table 4 ). There was a monotonic increase in the frequency of VH-TCFAs from the first to the fourth angiographic quartile (13.4%, 22.0%, 24.4%, and 30.3%, respectively, p <0.001; Figure 1 ). The frequency of ThCFA also increased among the 4 groups (p for trend = 0.03). Thus, combining VH-TCFAs and ThCFAs, the fibroatheroma frequency increased from 48.6% in the first quartile, to 56.2% in the second quartile, to 63.2% in the third quartile, and to 72.3% in the fourth quartile (p <0.001; Figure 1 ). In contrast, the incidence of pathologic intimal thickening decreased from the first to the fourth angiographic quartile (p <0.001). The frequencies of VH-TFCAs and ThCFAs with multiple confluent NCs increased from the first to the fourth angiographic quartile. The maximal arc of NC abutting to the lumen in lesions classified as VH-TCFAs significantly increased from the first to the fourth angiographic quartile. Similarly, total VH-TCFA length increased from 2.0 mm (range 1.6 to 2.4) in the first quartile to 4.2 mm (range 3.6 to 4.7) in the fourth quartile (p <0.001).
Variable | Quartile 1 (n = 702) | Quartile 2 (n = 692) | Quartile 3 (n = 696) | Quartile 4 (n = 690) | p Value |
---|---|---|---|---|---|
Volumetric analysis | |||||
NC volume (%) | 12.3 (11.6–13.0) | 12.5 (11.8–13.2) | 13.0 (12.3–13.7) | 14.0 (13.3–14.7) | <0.001 ⁎ |
Dense calcium volume (%) | 6.0 (5.4–6.6) | 6.1 (5.7–6.6) | 6.5 (6.0–7.0) | 7.2 (6.6–7.7) | <0.001 ⁎ |
Fibrous tissue volume (%) | 59.4 (58.6–60.2) | 59.8 (59.0–60.6) | 59.8 (59.1–60.6) | 59.1 (58.4–59.8) | 0.33 ⁎ |
Fibrofatty volume (%) | 22.3 (21.3–23.4) | 21.6 (20.6–22.6) | 20.6 (19.7–21.5) | 19.7 (18.8–20.6) | <0.001 ⁎ |
MLA site analysis | |||||
NC volume (%) | 12.8 (12.0–13.6) | 13.3 (12.5–14.1) | 13.7 (12.9–14.6) | 15.2 (14.3–16.1) | <0.001 ⁎ |
Dense calcium volume (%) | 5.8 (5.2–6.4) | 6.4 (5.8–7.0) | 6.6 (5.9–7.3) | 6.8 (6.2–7.5) | 0.06 ⁎ |
Fibrous tissue volume (%) | 59.2 (58.3–60.2) | 59.4 (58.4–60.4) | 60.1 (59.2–61.0) | 59.6 (58.6–60.5) | 0.55 ⁎ |
Fibrofatty volume (%) | 22.1 (20.9–23.3) | 20.9 (19.8–22.1) | 19.4 (18.4–20.5) | 18.4 (17.3–19.5) | <0.001 ⁎ |
Morphologic analysis | |||||
VH-TCFA with multiple confluent NC | 53 (7.5%) | 115 (16.6%) | 114 (16.4%) | 164 (23.8%) | <0.001 † |
VH-TCFA with single confluent NC | 41 (5.8%) | 37 (5.3%) | 56 (8.0%) | 45 (6.5%) | 0.19 † |
Total VH-TCFA length per lesion (mm) | 2.0 (1.6–2.4) | 3.2 (2.7–3.7) | 3.4 (2.9–4.0) | 4.2 (3.6–4.7) | <0.001 ⁎ |
Maximal arc of NC abutting to the lumen (°) | 71.2 (65.7–76.7) | 74.8 (70.5–79.0) | 78.6 (72.9–84.3) | 80.2 (76.1–84.2) | 0.04 ⁎ |
ThCFA with multiple confluent NC | 144 (20.5%) | 151 (21.8%) | 185 (26.6%) | 209 (30.3%) | 0.002 † |
ThCFA with single confluent NC | 103 (14.7%) | 86 (12.4%) | 85 (12.2%) | 81 (11.7%) | 0.45 † |
Total ThCFA length per lesion (mm) | 3.1 (2.7–3.4) | 5.0 (4.4–5.7) | 6.2 (5.5–6.8) | 7.7 (7.0–8.5) | <0.001 ⁎ |
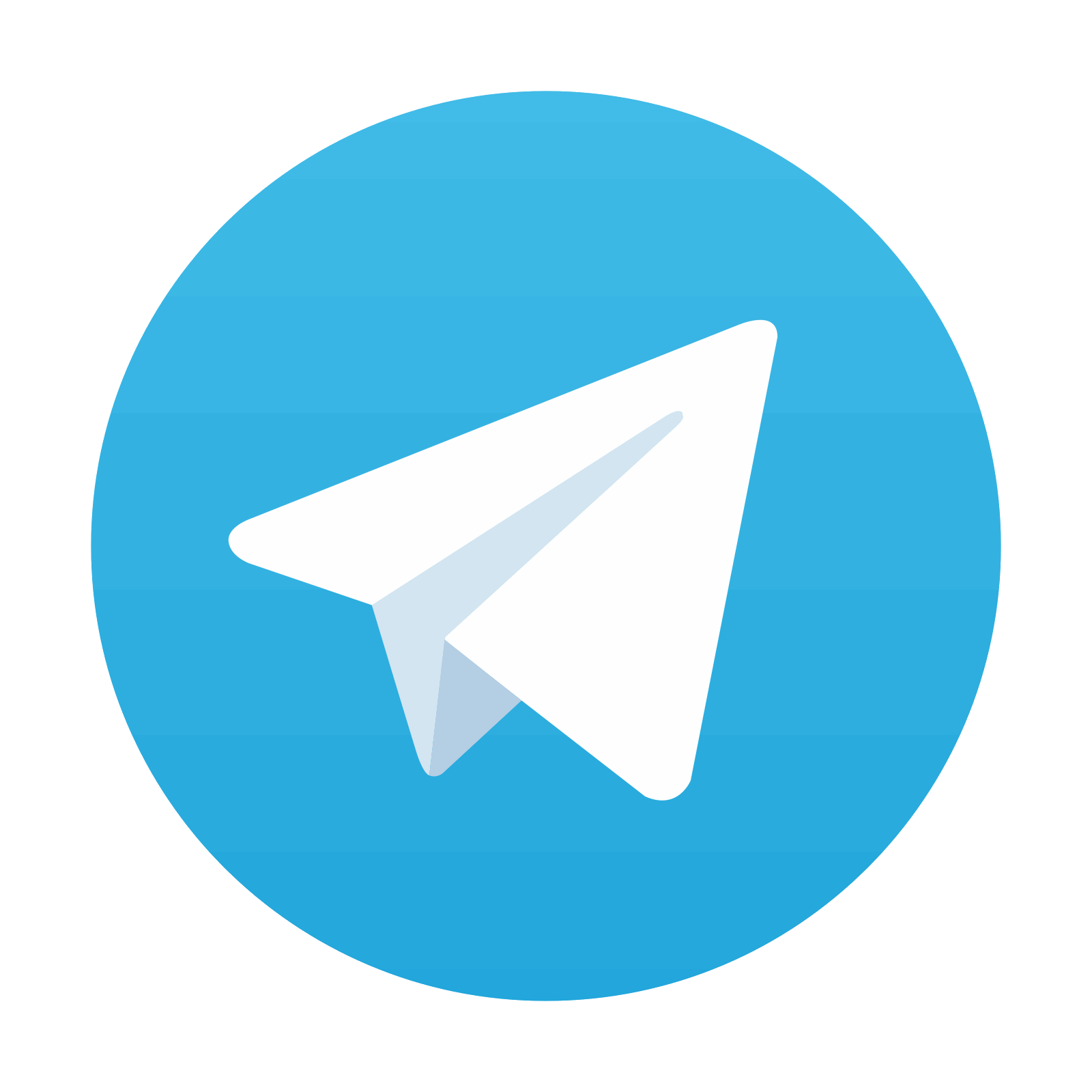
Stay updated, free articles. Join our Telegram channel

Full access? Get Clinical Tree
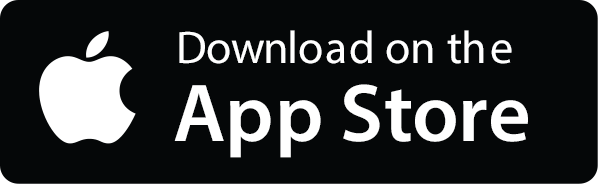
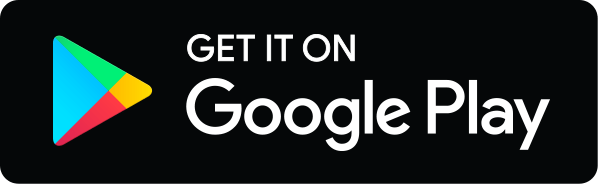
