Background
The quantification of right ventricular (RV) size and function is of diagnostic and prognostic importance. Recently, new software for the analysis of RV geometry using three-dimensional (3D) echocardiographic images has been validated. The aim of this study was to provide normal reference values for RV volumes and function using this technique.
Methods
A total of 245 subjects, including 15 to 20 subjects for each gender and age decile, were studied. Dedicated 3D acquisitions of the right ventricle were obtained in all subjects.
Results
The mean RV end-diastolic and end-systolic volumes were 49 ± 10 and 16 ± 6 mL/m 2 respectively, and the mean RV ejection fraction was 67 ± 8%. Significant correlations were observed between RV parameters and body surface area. Normalized RV volumes were significantly correlated with age and gender. RV ejection fractions were lower in men, but differences across age deciles were not evident.
Conclusion
The current study provides normal reference values for RV volumes and function that may be useful for the identification of clinical abnormalities.
The evaluation of right ventricular (RV) dimension and function is known to be of clinical importance in many cardiac diseases. However, because of the complexity of RV anatomy, precise two-dimensional (2D) echocardiographic estimations of RV volumes have been nearly impossible because of the need to apply simple geometric assumptions, even though RV chamber shape is quite complex. With the introduction of real-time three-dimensional (3D) transthoracic echocardiographic (3DTTE) imaging, new dedicated RV quantification software has been proposed and validated in comparison with cardiac magnetic resonance (CMR) and radionuclide ventriculography as gold standards. The accuracy of the 3DTTE method has been analyzed in vitro and in patients with different pathologies, as well as in limited series of normal subjects. As is the case with left ventricular (LV) volumes, despite the excellent correlation, 3D echocardiography has been demonstrated to slightly underestimate CMR RV volumes. To our knowledge, few data are available on 3D normal reference values of RV dimension and function. Accordingly, the aims of our study were threefold: to obtain normal 3D echocardiographic reference ranges for RV volumes and function in a large series of normal subjects, to correlate volumes with gender and age deciles (from 20 to 80 years), and to analyze the accuracy of the method by determining the reproducibility and test-retest variability of 3D volume measurements.
Methods
Population and Study Protocol
A total of 260 subjects of both genders and across a wide range of ages, recruited from the employees of Centro Cardiologico Monzino (Milan, Italy) and their relatives, were prospectively studied. The study design aimed to include 15 to 20 normal subjects for each gender and age decile from 20 to 80 years. Exclusion criteria were poor echocardiographic image quality, obesity (body mass index ≥ 28 kg/m 2 ), hypertension, diabetes mellitus, anemia, endocrinal dysfunction, and previous cardiovascular diseases. Moreover, 2D echocardiographic examinations were performed in all subjects to exclude any subclinical structural cardiac abnormalities. Subjects with trivial mitral regurgitation were included. The protocol was approved by the institutional review board, and informed consent was obtained from all participants.
Two-Dimensional Echocardiography
All echocardiographic exams were performed using an iE33 system (Philips Medical Systems, Andover, MA) using the S5-1 sector-array probe. Complete standard M-mode and 2D echocardiographic examinations were performed according to clinical laboratory practice. From the 4-chamber apical view, the M-mode cursor was positioned at the junction of the tricuspid valve plane with the RV free wall to evaluate tricuspid annular plane systolic excursion (TAPSE), defined as the difference in the displacement of the RV base from end-diastole to end-systole and used as an index of RV function.
Systolic artery pulmonary pressure was obtained using the noninvasive Doppler echocardiographic method from the systolic RV–right atrial gradient calculated from the peak velocity of systolic transtricuspid regurgitant flow signal using the modified Bernoulli equation and right atrial pressure derived by means of the inferior vena cava collapsibility index measured in the subcostal view.
Real-Time 3DTTE Imaging
Real-time 3DTTE imaging was performed at the end of the 2D examination using the same ultrasound unit and the X3-1 matrix-array probe, imaging with temporal resolution ranging from 26 to 37 frames/s. Two consecutive acquisitions were obtained (gathered over 7 consecutive cardiac cycles) for each of the 2 ventricles in full-volume mode from the 4-chamber apical view adapted to improve the visualization of the RV and LV cavities, respectively. The volumetric data sets were digitally stored and then transferred into a workstation for offline postprocessing analysis and 3D reconstruction.
Quantification of RV Volumes and Function
A commercially available dedicated system (EchoView, TomTec Imaging, Inc, Munich, Germany) equipped with four-dimensional RV analysis software was used to analyze the RV 3DTTE volumetric data sets ( Figure 1 A). Briefly, after manual selection of the central reference points of the tricuspid and mitral valve annuli and of the LV apex, the software visualized the RV cavity by the coronal, sagittal, and frontal cut planes automatically obtained from the 3D data set both at end-systole and at end-diastole. The first frame corresponding to the R wave of the electrocardiogram (cycles are aligned for R-R tracking) was used as end-diastole. The automatic identification of end-systole, however, needed adjustment by visual inspection of the cine loops, resulting in the manual selection of the frame with the smallest RV cavity size. After manual tracing of the endocardial borders, on each of the 3 cut planes at both end-diastole and end-systole, the software automatically detects the RV surfaces throughout the cardiac cycle. Manual correction to adjust the endocardial contours in each frame prior to quantification was performed when needed. Papillary muscles, moderator bands, and trabeculae were included in the cavity volume ( Figure 1 B). RV volumes were computed throughout the cardiac cycle, from which RV end-diastolic volume (RVEDV) and RV end-systolic volume (RVESV) ( Figure 1 C) were obtained as maximums and minimums, respectively. RV stroke volume (RVSV) and RV ejection fraction (RVEF) were then measured as the difference and as the percentage change of the volumes, respectively. The postprocessing quantification required 4 ± 2 minutes.

Quantification of LV Volumes and Function
The LV 3DTTE volumetric data sets were analyzed using commercial software (4D LV-Function 2.0, TomTec Imaging, Inc), as previously described. Briefly, end-diastolic and end-systolic volumes were semiautomatically derived after manual tracing of the endocardial borders in 4-chamber, 3-chamber, and 2-chamber views. From the obtained values, LV stroke volume (LVSV) and LV ejection fraction were calculated.
Statistical Analysis
All considered parameters were found to be normally distributed using the Kolmogorov-Smirnov test and are therefore presented as mean ± SD. To assess the relationship between RV volumes and RVSV with body surface area (BSA), Pearson’s correlation coefficient ( r ) was computed. To verify the dependence of age deciles and gender on BSA-normalized RV volumes, Spearman’s rank correlation coefficient (ρ) was used. Furthermore, the relationship between RVSV and LVSV was evaluated using linear regression (Pearson’s correlation coefficient) and Bland-Altman analyses.
To assess the reproducibility of the semiautomated quantification of RV volumes in a subset of 20 randomly chosen subjects, the main investigator reevaluated the same 3D data sets ≥2 weeks after the first analysis, blinded with respect to the results of the previous evaluation. For each computed parameter, intraobserver variability was then evaluated. The same subset was also evaluated by a different observer, blinded to the results obtained by the main investigator, to assess interobserver variability. Both intraobserver and interobserver variability were expressed as coefficients of variation (percentages), defined as the standard deviation of the difference between the two measurements, multiplied by 100 and divided by their mean value. In addition, to evaluate the bias and limits of intraobserver or interobserver agreement, Bland-Altman analysis was applied. Also, a test-retest analysis was performed using the second 3DTTE data set acquired during the same examination and the relevant coefficient of variation computed for each parameter.
Results
Of 260 enrolled subjects, a total of 15 subjects (6%) were excluded from further analysis because of inadequate 3DTTE image quality, despite the good 2D acoustic windows. Baseline characteristics of the studied population (245 subjects) are summarized in Table 1 .
All | Men | Women | |
---|---|---|---|
(n = 245) | (n = 119) | (n = 126) | |
Age (y) | |||
<30 | 44 | 22 | 22 |
30-39 | 40 | 20 | 22 |
40-49 | 43 | 20 | 23 |
50-59 | 43 | 22 | 21 |
60-69 | 39 | 20 | 19 |
>70 | 34 | 15 | 19 |
Mean age (y) | 48 ± 17 | 48 ± 17 | 49 ± 17 |
BSA (m 2 ) | 1.8 ± 0.2 | 1.9 ± 0.1 | 1.6 ± 0.1 |
Systolic pulmonary pressure (mm Hg) | 26 ± 4 | 26 ± 3 | 26 ± 4 |
TAPSE (mm) | 24 ± 3 | 25 ± 3 | 24 ± 3 |
LV end-diastolic volume (mL/m 2 ) | 54 ± 8 | 56 ± 9 | 51 ± 7 |
LV end-systolic volume (mL/m 2 ) | 20 ± 5 | 22 ± 6 | 18 ± 5 |
LV stroke volume (mL/m 2 ) | 34 ± 6 | 35 ± 6 | 33 ± 5 |
LV ejection fraction (%) | 63 ± 7 | 62 ± 7 | 64 ± 7 |
Normal ranges for RV volumes, RVSV, and RVEF across age deciles, with gender differentiation, are reported in Table 2 . A good correlation ( r = 0.87, P < .001) was observed between RVSV and LVSV, with narrow limits of agreement (±13.4 mL) and minimal bias (−1.5 mL) ( Figure 2 ).
RVEDV (mL) | RVESV (mL) | RVEF (%) | |||||||
---|---|---|---|---|---|---|---|---|---|
Age decile (y) | All | Men | Women | All | Men | Women | All | Men | Women |
<30 | 92 ± 23 | 107 ± 22 | 78 ± 12 | 33 ± 13 | 41 ± 12 | 24 ± 8 | 66 ± 8 | 62 ± 6 | 69 ± 9 |
30-39 | 88 ± 20 | 99 ± 22 | 79 ± 11 | 30 ± 10 | 35 ± 10 | 25 ± 7 | 66 ± 8 | 64 ± 8 | 69 ± 7 |
40-49 | 86 ± 19 | 96 ± 20 | 76 ± 13 | 28 ± 10 | 34 ± 10 | 22 ± 7 | 68 ± 8 | 64 ± 8 | 71 ± 7 |
50-59 | 87 ± 20 | 99 ± 21 | 74 ± 8 | 30 ± 11 | 36 ± 11 | 24 ± 6 | 66 ± 7 | 65 ± 6 | 67 ± 8 |
60-69 | 82 ± 22 | 96 ± 13 | 68 ± 19 | 27 ± 10 | 31 ± 10 | 23 ± 10 | 67 ± 9 | 68 ± 9 | 67 ± 10 |
>70 | 80 ± 22 | 94 ± 23 | 70 ± 15 | 26 ± 11 | 33 ± 12 | 21 ± 7 | 68 ± 7 | 65 ± 6 | 71 ± 7 |
All | 86 ± 21 | 99 ± 14 | 74 ± 14 | 29 ± 11 | 35 ± 7 | 23 ± 7 | 67 ± 8 | 64 ± 8 | 69 ± 8 |

Significant Pearson’s correlation coefficients (RVEDV, r = 0.59; RVESV, r = 0.51; RVSV, r = 0.48; RVEF, r = −0.21; P < .01) between RV parameters and BSA were observed. Consequently, RV volumes and RVSV were normalized for BSA, and the ranges for normalized RV parameters are reported in Table 3 . The upper limit for normal values of RVEDV was 68 mL/m 2 for the entire group, ranging from 61 mL/m 2 (women, fifth age decile) to 79 mL/m 2 (men, first age decile). The upper limit for normal values of RVESV was 27 mL/m 2 for the whole population, ranging from 21 mL/m 2 (women, sixth age decile) to 34 mL/m 2 (men, first age decile). The lower reference value for RVEF was 51%.
RVEDV index (mL/m 2 ) | RVESV index (mL/m 2 ) | |||||
---|---|---|---|---|---|---|
Age decile (y) | All | Men | Women | All | Men | Women |
<30 | 53 ± 10 | 57 ± 11 | 48 ± 6 | 18 ± 7 | 22 ± 6 | 15 ± 5 |
30-39 | 49 ± 8 | 50 ± 10 | 49 ± 6 | 16 ± 4 | 18 ± 5 | 15 ± 4 |
40-49 | 48 ± 9 | 50 ± 9 | 47 ± 8 | 16 ± 5 | 18 ± 5 | 14 ± 5 |
50-59 | 49 ± 9 | 52 ± 10 | 46 ± 8 | 17 ± 5 | 19 ± 5 | 15 ± 4 |
60-69 | 46 ± 10 | 50 ± 6 | 41 ± 10 | 15 ± 5 | 16 ± 5 | 13 ± 5 |
>70 | 46 ± 12 | 50 ± 13 | 43 ± 10 | 15 ± 6 | 18 ± 7 | 13 ± 4 |
All | 49 ± 10 | 52 ± 8 | 46 ± 8 | 16 ± 6 | 18 ± 4 | 14 ± 4 |
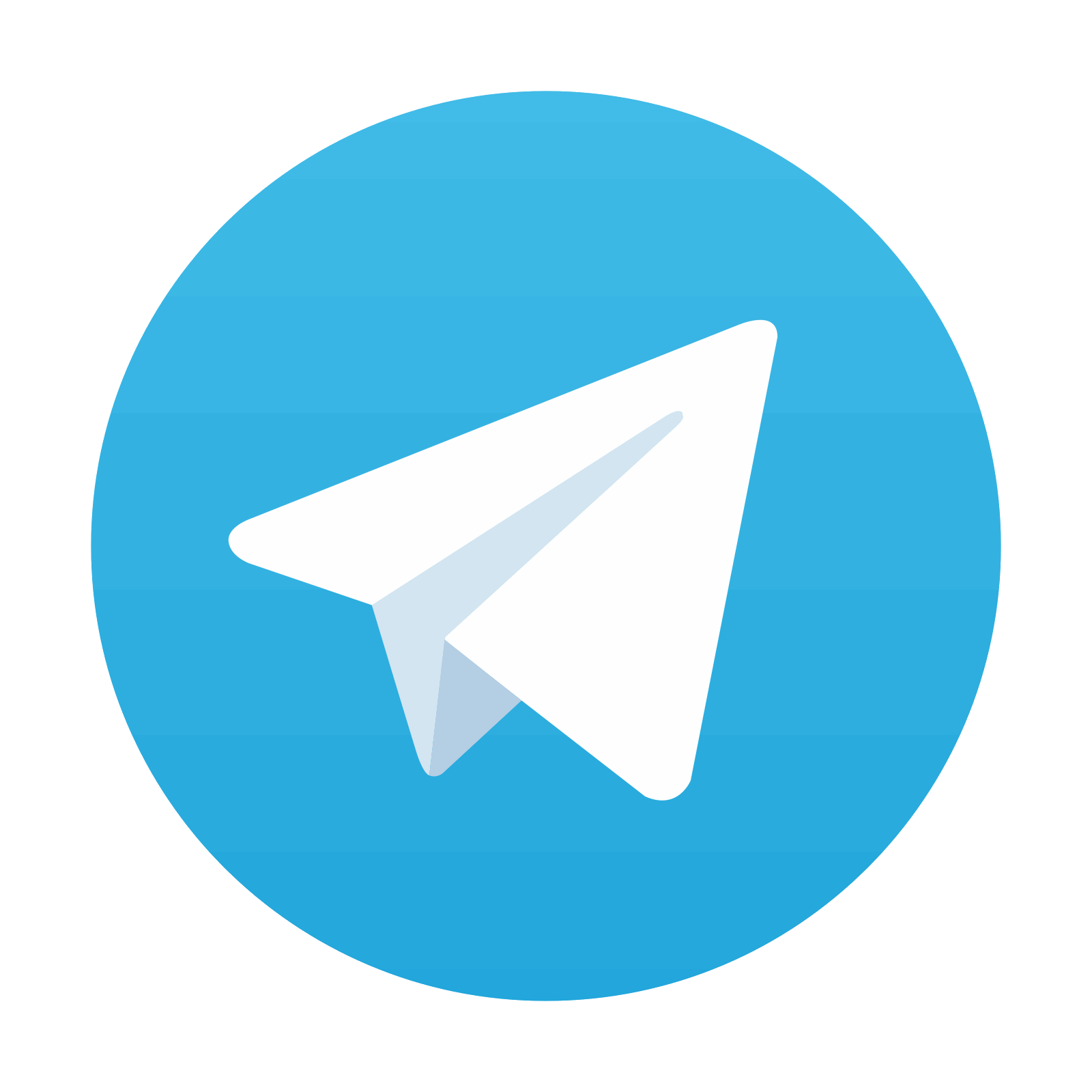
Stay updated, free articles. Join our Telegram channel

Full access? Get Clinical Tree
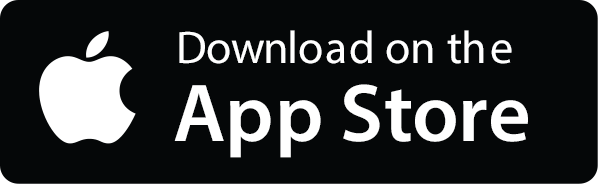
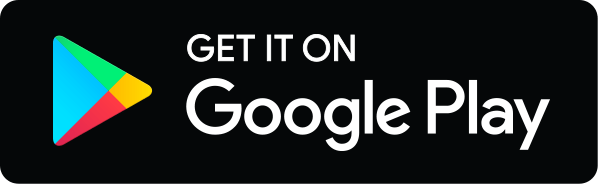
