Background
The accurate evaluation of intrinsic myocardial contractility in children with or without congenital heart disease (CHD) has turned out to be a challenge. Two-dimensional strain echocardiographic (2DSTE) imaging or two-dimensional speckle-tracking echocardiographic imaging appears to hold significant promise as a tool to improve the assessment of ventricular myocardial function. The aim of this study was to estimate left ventricular myocardial systolic function using 2DSTE imaging in a large cohort consisting of healthy children and young adults to establish reference strain values.
Methods
Transthoracic echocardiograms were acquired in 195 healthy subjects (139 children, 56 young adults) and were retrospectively analyzed. Longitudinal, circumferential, and radial peak systolic strain values were determined by means of speckle tracking. Nonlinear regression analysis was performed to assess the effect of aging on these 2DSTE parameters.
Results
There was a strong, statistically significant second-order polynomial relation ( P < .001) between global peak systolic strain parameters and age. Global peak systolic strain values were lowest in the youngest and oldest age groups.
Conclusion
This is the first report to establish age-dependent reference values per cardiac segment for myocardial strain in all three directions assessed using 2DSTE imaging in a large pediatric and young adult cohort. There is a need to use age-specific reference values for the adequate interpretation of 2DSTE measurements.
Despite impressive developments in echocardiographic technology during the past 50 years, there are still important challenges that new techniques try to meet. One important yet partially unresolved challenge that remains is the quantification of ventricular myocardial function in children with congenital heart disease (CHD). Traditional methods, such as the one-dimensional M-mode technique (e.g., fractional shortening) and two-dimensional imaging (e.g., ejection fraction), are used to evaluate left ventricular function. However, these methods are not always applicable to pediatric hearts with complex congenital malformations. The cardinal reason for this is that they are based on geometric assumptions and overlook the complex characteristics of CHD. Moreover, those current indices provide no information about regional alterations in ventricular myocardial contraction. These disadvantages complicate the assessment of systolic and diastolic function in children with CHD and underscore the need for better quantitative techniques to assess ventricular function in this subset of patients.
New modalities, such as strain imaging, have been developed to address the previously mentioned shortcomings. Myocardial strain is a dimensionless assessment of regional ventricular deformation (i.e., a percentage of deformation), whereas myocardial strain rate (SR), which is a time derivate of strain, indicates the rate of deformation of a defined myocardial segment. Multiple studies have proven the accuracy and reliability of strain (and SR) techniques in the assessment of (regional) ventricular myocardial function. Moreover, these techniques appear to be able to detect subclinical myocardial dysfunction at an earlier stage compared with conventional imaging modalities. Several diagnostic imaging modalities to assess myocardial strain and SR have been developed in recent years. In addition to tagged magnetic resonance imaging (MRI) and Doppler tissue imaging (DTI), a third method to determine myocardial strain was introduced some years ago. This relatively novel technique is based on two-dimensional echocardiographic images, hence the name: two-dimensional strain echocardiographic (2DSTE) imaging. It determines myocardial deformation by means of frame-by-frame tracking and motion analysis of speckles within B-mode images using optical flow algorithms. Validation studies with tagged MRI and sonomicrometry in the adult population have provided evidence that 2DSTE imaging is a reliable method to determine ventricular myocardial function. Despite its limitations, such as sensitivity to noise, it has the advantages over DTI-based strain imaging that the strain estimates are not angle dependent and that strain is obtained in two dimensions. Intraobserver and interobserver reliability scores have been established previously and are high for almost all parameters. Because this novel technique could be easily accessible and applicable, and moreover is not based on geometric assumptions, it may serve as an important tool in the future evaluation of regional and global ventricular function in pediatric subjects with or without CHD. The establishment of normal values in a healthy pediatric cohort is a mandatory prerequisite for its use in evaluating (pathologic) changes in ventricular function. Data in this area are scarce and include only peak systolic strain in one or two directions. For these reasons, we aimed to evaluate left ventricular myocardial strain in all three directions (longitudinal, circumferential, and radial) assessed by means of 2DSTE imaging in a large, healthy pediatric and young adult cohort to establish 2DSTE reference values and to determine the influence of age and growth on these values.
Materials and Methods
Study Population
Subjects who were routinely referred for echocardiographic evaluation of an asymptomatic, innocent heart murmur between May 1, 2005, and November 1, 2009, were retrospectively analyzed for their eligibility for inclusion in the study. All consecutive healthy subjects age from birth to 40 years were identified from our echocardiographic database from the outpatient clinic at both the Children’s Heart Centre and the Adult Heart Centre (Nijmegen, The Netherlands). Patients with structural (congenital) heart disease or abnormal cardiac rhythms were excluded. Other exclusion criteria consisted of hypertension and/or chronic or recent acute illness. Demographic characteristics, including age and gender, were collected at the time the echocardiographic studies were performed. Informed consent was obtained from each participant. This study was approved by the local medical ethics committee.
Two-Dimensional Strain Echocardiographic Data Acquisition
A complete physical examination was performed, including weight, height, and blood pressure measurements (see Table 1 ). Subsequently, all subjects underwent a detailed transthoracic echocardiographic examination in the left lateral position according to the recommendations of the American Society of Echocardiography. Every examination was performed at rest, without using sedation. Images were obtained with a 3.0-MHz (S3) or a 5.0-MHz (S5) phased-array transducer using a commercially available system, the Vivid 7 echocardiographic scanner (GE Vingmed Ultrasound AS, Horten, Norway). The choice of an S3 or S5 transducer depended on the age and posture of the child. Two-dimensional multiframe B-mode (grayscale) images were obtained in the apical four-chamber and parasternal midcavity short-axis view (at the level of the papillary muscle) and parasternal basal short-axis view (at the level of the mitral valve). A sector scan angle of 30° to 60° was chosen, and frame rates of 70 to 90 Hz were used, because these rates are considered to be optimal for two-dimensional speckle tracking. Data were stored at the same frame rate as the acquisition frame rate. Preferably images from three cardiac cycles triggered by the R wave of the QRS complex were digitally saved in cine loop format. Offline speckle-tracking analysis was performed using software for echocardiographic quantification (EchoPAC version 6.1.0; GE Vingmed Ultrasound AS). The timing of aortic valve closure and mitral valve opening with respect to peak systolic strain were manually obtained, using single gated pulsed-wave Doppler or continuous-wave Doppler images of the left ventricular outflow tract. For these measurements, special care was taken to keep the heart rate in the same range as during the two-dimensional grayscale imaging used for two-dimensional strain calculations. Endomyocardial borders of the left ventricle were manually traced within the end-systolic frame. The second, epicardial tracing was automatically generated by the computer algorithm and, when necessary, manually adjusted to cover the whole myocardial wall. The tracking algorithm then followed the myocardial speckles during the cardiac cycle. Tracking was accepted only if both visual inspection as well as the EchoPAC software indicated adequate tracking. This means that tracking of any given segment was accepted only when it was indicated with a green box. The software automatically divided the cross-sectional image into six segments, which were named and identified according to the statement of the Cardiac Imaging Committee of the Council on Clinical Cardiology of the American Heart Association. The left ventricular segments to be analyzed were the apical, middle, and basal segments of the septal and the lateral wall of the four-chamber view, as well as the anteroseptal, anterior, lateral, posterior, inferior, and septal segments of the basal and midcavity short-axis views. Strain curves of the three consecutive cardiac cycles and values of the manual timing were imported into a custom-made software package for further analysis. The Q-Q interval was estimated from the electrocardiographic signal to obtain cardiac cycle length. It is known that the systolic phase of the cardiac cycle does not change much with small changes in heart rate, in contrast to the diastolic phase. Therefore, the diastolic phase of the three cardiac cycles was automatically extended and adjusted by the software package to the longest of the three cardiac cycles. This intervention prevents a shift of the peak systolic strain while averaging the three consecutive cardiac cycles. Cardiac cycles with lengths >10% different from the mean length of the three cardiac cycles were excluded from averaging and thus from further analysis. Myocardial longitudinal, radial, and circumferential strain values were obtained. To determine global strain, the strain values of the six segments were averaged for the four-chamber as well as the short-axis views. Strain values are dimensionless and are expressed as percentages. Negative strain values reflect shortening, while positive strain values reflect lengthening or thickening. All offline measurements with EchoPAC were performed by a single observer (K.A.M.). Interobserver and intraobserver variability was determined by measurement of left ventricular myocardial strain in 40 randomly selected subjects (five in each age group). To assess intraobserver variability, the same observer (K.A.M.) measured the left ventricular segments again with an interval >2 months to avoid recall bias. To assess interobserver variability, strain measurements were performed by a second observer (M.B.), who was blinded to the results of the first observer (K.A.M.). Figures 1 and 2 show an illustration of two-dimensional strain imaging.
Age group | ||||||||
---|---|---|---|---|---|---|---|---|
1 | 2 | 3 | 4 | 5 | 6 | 7 | 8 | |
Variable | 0 y | 1–4 y | 5–9 y | 10–14 y | 15–19 y | 20–24 y | 25–29 y | 30–40 y |
Number | 24 | 34 | 36 | 29 | 21 | 25 | 13 | 13 |
Male | 13 (54%) | 19 (56%) | 25 (69%) | 16 (55%) | 9 (43%) | 16 (64%) | 8 (62%) | 6 (46%) |
Age (y) | 0.3 ± 0.3 | 2.9 ± 1.0 | 7.2 ± 1.2 | 12.8 ± 1.6 | 17.0 ± 1.3 | 21.7 ± 1.2 | 27.3 ± 1.3 | 35.6 ± 2.6 |
Height (m) | 0.62 ± 0.11 | 0.95 ± 0.10 | 1.26 ± 0.09 | 1.59 ± 0.13 | 1.76 ± 0.09 | 1.78 ± 0.10 | 1.82 ± 0.08 | 1.77 ± 0.10 |
Weight (kg) | 6.3 ± 2.6 | 14.6 ± 3.4 | 24.7 ± 4.4 | 46.3 ± 12.0 | 66.4 ± 12.0 | 70.3 ± 11.9 | 76.5 ± 11.9 | 77.8 ± 11.0 |
BSA (m 2 ) | 0.32 ± 0.10 | 0.62 ± 0.10 | 0.93 ± 0.12 | 1.43 ± 0.23 | 1.81 ± 0.20 | 1.86 ± 0.20 | 1.96 ± 0.19 | 1.95 ± 0.18 |
BMI (kg/m 2 ) | 15.9 ± 2.2 | 15.9 ± 1.4 | 15.4 ± 1.3 | 17.9 ± 2.1 | 21.2 ± 2.3 | 22.1 ± 2.0 | 23.1 ± 2.2 | 24.9 ± 2.9 |
HR (beats/min) | 118 ± 12 | 101 ± 14 | 84 ± 13 | 77 ± 14 | 65 ± 9 | 63 ± 11 | 60 ± 14 | 65 ± 8 |
SBP (mm Hg) | 82 ± 8 | 98 ± 10 | 104 ± 8 | 110 ± 10 | 116 ± 11 | 118 ± 12 | 121 ± 11 | 123 ± 12 |
DBP (mm Hg) | 56 ± 6 | 62 ± 10 | 70 ± 8 | 72 ± 8 | 75 ± 9 | 75 ± 8 | 77 ± 8 | 78 ± 9 |


Conventional Echocardiographic Parameters
Quantification of cardiac chamber size, ventricular mass, and systolic and diastolic left ventricular function were measured in accordance with the recommendations for chamber quantification of the American Society of Echocardiography. Left ventricular systolic function was indicated using fractional shortening, ejection fraction, left ventricular myocardial performance index (Tei index), end-systolic wall stress, and rate-corrected velocity of circumferential fiber shortening (VCFc). Ejection fraction was calculated using the modified Simpson’s rule. The pulsed-wave Doppler–derived myocardial performance index was calculated by adding the isovolumetric contraction time and the isovolumetric relaxation time and dividing the sum by the ejection time. Left ventricular end-systolic wall stress was calculated using the modified formula of Rowland and Gutgesell. VCFc was calculated using the formula obtained from Colan et al. Left ventricular mass (LVM) was calculated using the formula for estimation of LVM according to Devereux and Reichek and was subsequently indexed to body surface area.
Statistical Analysis
Patients were divided into eight different age groups (see Table 1 ) for further analysis. All demographic, conventional echocardiographic, and two-dimensional strain values are expressed as mean ± SD. The relations between age and global strain parameters were determined using scatterplots, one-way analysis of variance, and second-order polynomial regression analysis. Multiple linear regression analysis was performed to determine the additional effect of anthropometrics (including heart rate, weight, and height) and conventional echocardiographic parameters on the myocardial global strain variables.
Intraobserver and interobserver agreement was calculated using the Bland-Altman approach, including the calculation of mean bias (average difference between measurements), the statistical significance of the mean bias on paired t tests (the null hypothesis was zero bias), and the lower and upper limits of agreement (95% limits of agreement of mean bias). In addition, the coefficient of variation was determined (i.e., the standard deviation of the difference of paired samples divided by the average of the paired samples). P values < .05 was considered to indicate significance. Statistical analyses were performed using SPSS for Windows version 16.0 (SPSS, Inc., Chicago, IL).
Results
A total of 226 subjects were evaluated for inclusion in the study. Of those subjects, 31 (13.7%) were subsequently excluded in light of incomplete echocardiographic data or suboptimal imaging quality. In total, 195 healthy subjects (139 children and 56 young adults) were enrolled in the study. Subject characteristics and anthropometric parameters are described in Table 1 . Conventional echocardiographic parameters of the study subjects are presented in Table 2 . All standard echocardiographic findings were within previously described normal values. Myocardial strain parameters are presented in Tables 3 to 5 and Figures 3 to 5 . Tracking was feasible in 91% of all segments in the four-chamber view, in 96% of all segments in the short-axis view at the level of the papillary muscle, and in 91% of all segments in the short-axis view at the level of the mitral valve.
Age group | ||||||||
---|---|---|---|---|---|---|---|---|
1 | 2 | 3 | 4 | 5 | 6 | 7 | 8 | |
Variable | 0 y | 1–4 y | 5–9 y | 10–14 y | 15–19 y | 20–24 y | 25–29 y | 30–40 y |
LVET (sec) | 0.19 ± 0.01 | 0.23 ± 0.01 | 0.26 ± 0.01 | 0.28 ± 0.01 | 0.29 ± 0.01 | 0.29 ± 0.01 | 0.29 ± 0.01 | 0.30 ± 0.01 |
LVETc (sec) | 0.28 ± 0.02 | 0.30 ± 0.02 | 0.30 ± 0.02 | 0.32 ± 0.03 | 0.30 ± 0.02 | 0.29 ± 0.03 | 0.29 ± 0.03 | 0.31 ± 0.03 |
VCFc (circ/sec) | 1.32 ± 0.22 | 1.28 ± 0.20 | 1.25 ± 0.19 | 1.19 ± 0.19 | 1.31 ± 0.20 | 1.21 ± 0.43 | 1.20 ± 0.43 | 1.20 ± 0.41 |
Tei index | 0.37 ± 0.01 | 0.37 ± 0.01 | 0.38 ± 0.01 | 0.38 ± 0.03 | 0.40 ± 0.03 | 0.39 ± 0.03 | 0.38 ± 0.01 | 0.38 ± 0.02 |
FS | 0.37 ± 0.05 | 0.38 ± 0.05 | 0.37 ± 0.04 | 0.37 ± 0.04 | 0.39 ± 0.06 | 0.39 ± 0.07 | 0.37 ± 0.05 | 0.40 ± 0.04 |
Biplane EF | 0.73 ± 0.07 | 0.71 ± 0.06 | 0.67 ± 0.05 | 0.70 ± 0.06 | 0.67 ± 0.07 | 0.74 ± 0.04 | 0.77 ± 0.04 | 0.75 ± 0.05 |
ESWS (g/cm 2 ) | 30.7 ± 11.0 | 42.8 ± 13.9 | 43.6 ± 10.7 | 45.3 ± 11.6 | 44.4 ± 13.4 | 40.0 ± 10.4 | 45.7 ± 16.2 | 41.8 ± 10.1 |
LVM/BSA (g/m 2 ) | 36.2 ± 12.1 | 48.5 ± 11.6 | 57.2 ± 12.3 | 59.9 ± 14.2 | 71.8 ± 16.0 | 78.6 ± 18.0 | 77.3 ± 19.1 | 75.4 ± 17.2 |
Age group | ||||||||
---|---|---|---|---|---|---|---|---|
1 | 2 | 3 | 4 | 5 | 6 | 7 | 8 | |
Variable | 0 y | 1–4 y | 5–9 y | 10–14 y | 15–19 y | 20–24 y | 25–29 y | 30–40 y |
Septum | ||||||||
Basal | −17.0 ± 2.6 | −19.6 ± 1.5 | −20.1 ± 1.8 | −20.0 ± 1.7 | −20.4 ± 1.6 | −19.6 ± 1.5 | −19.2 ± 1.3 | −17.5 ± 1.5 |
Mid | −18.2 ± 2.7 | −20.9 ± 1.3 | −21.1 ± 1.4 | −21.5 ± 1.6 | −22.5 ± 1.4 | −20.3 ± 1.8 | −20.3 ± 1.4 | −18.8 ± 1.4 |
Apical | −20.4 ± 1.9 | −22.7 ± 1.7 | −22.6 ± 1.5 | −23.8 ± 1.3 | −25.1 ± 1.2 | −22.8 ± 1.9 | −22.0 ± 2.0 | −20.7 ± 1.4 |
Lateral wall | ||||||||
Apical | −19.6 ± 1.5 | −22.5 ± 1.7 | −22.6 ± 1.5 | −23.9 ± 1.7 | −25.0 ± 1.4 | −22.7 ± 1.8 | −22.4 ± 1.8 | −20.5 ± 1.2 |
Mid | −16.2 ± 1.1 | −20.2 ± 1.6 | −20.1 ± 1.8 | −21.7 ± 1.6 | −22.4 ± 1.7 | −20.3 ± 1.6 | −20.3 ± 1.0 | −19.1 ± 1.1 |
Basal | −15.8 ± 1.3 | −19.4 ± 1.5 | −19.3 ± 1.8 | −20.0 ± 1.2 | −20.5 ± 1.7 | −19.4 ± 1.3 | −19.7 ± 1.2 | −17.7 ± 1.2 |
Global S-L | −18.3 ± 1.9 ∗ | −20.7 ± 1.3 ∗ | −21.0 ± 1.3 ∗ | −21.8 ± 1.3 ∗ | −22.5 ± 1.3 ∗ | −20.9 ± 1.3 ∗ | −20.6 ± 1.2 ∗ | −18.9 ± 1.0 ∗ |
5th percentile global S-L | −14.5 | −18.1 | −18.4 | −19.2 | −19.9 | −18.3 | −18.2 | −16.9 |
95th percentile global S-L | −22.1 | −23.3 | −23.6 | −24.4 | −25.1 | −23.5 | −23.0 | −20.9 |
∗ P < .05 vs with age groups denoted by superscript numerals, determined by one-way analysis of variance with Bonferroni’s correction for multiple comparisons.
Age group | ||||||||
---|---|---|---|---|---|---|---|---|
1 | 2 | 3 | 4 | 5 | 6 | 7 | 8 | |
Variable | 0 y | 1–4 y | 5–9 y | 10–14 y | 15–19 y | 20–24 y | 25–29 y | 30–40 y |
PM | ||||||||
Anteroseptal | 43.3 ± 10.7 | 44.9 ± 5.8 | 44.5 ± 7.2 | 46.5 ± 6.1 | 48.7 ± 3.9 | 47.7 ± 6.9 | 47.9 ± 6.5 | 44.3 ± 5.7 |
Anterior | 50.0 ± 14.8 | 51.9 ± 7.8 | 52.8 ± 8.5 | 57.1 ± 5.7 | 55.9 ± 4.4 | 55.5 ± 5.8 | 51.5 ± 6.4 | 52.9 ± 5.8 |
Lateral | 58.5 ± 12.0 | 58.8 ± 8.6 | 59.4 ± 7.8 | 62.8 ± 5.8 | 61.2 ± 4.6 | 62.0 ± 5.9 | 57.0 ± 6.3 | 56.4 ± 5.9 |
Posterior | 63.2 ± 11.6 | 61.1 ± 8.9 | 64.8 ± 7.1 | 66.3 ± 5.5 | 66.8 ± 4.1 | 66.4 ± 5.5 | 63.1 ± 5.8 | 62.2 ± 5.6 |
Inferior | 56.6 ± 12.6 | 58.5 ± 9.0 | 61.2 ± 6.1 | 65.2 ± 6.5 | 64.3 ± 4.3 | 60.9 ± 4.8 | 60.2 ± 5.3 | 60.5 ± 6.4 |
Septal | 40.1 ± 7.8 | 45.9 ± 6.5 | 46.6 ± 5.4 | 50.1 ± 5.9 | 51.7 ± 5.3 | 51.1 ± 5.8 | 48.0 ± 5.7 | 49.1 ± 4.2 |
Global RP | 52.0 ± 9.9 ∗ | 53.5 ± 6.7 | 54.9 ± 5.5 | 58.0 ± 5.4 ∗ | 58.1 ± 4.0 ∗ | 57.3 ± 5.0 | 54.6 ± 5.3 | 54.2 ± 4.7 |
5th percentile global S-RP | 32.2 | 40.1 | 43.9 | 47.2 | 50.1 | 47.3 | 44.0 | 44.8 |
95th percentile global S-RP | 71.8 | 66.9 | 65.9 | 68.8 | 66.1 | 67.3 | 65.2 | 63.6 |
MV | ||||||||
Anteroseptal | 39.6 ± 4.2 | 41.6 ± 5.1 | 43.4 ± 5.1 | 46.8 ± 5.3 | 49.9 ± 3.4 | 48.2 ± 5.1 | 46.0 ± 5.3 | 43.2 ± 5.1 |
Anterior | 46.7 ± 5.6 | 48.3 ± 7.0 | 49.2 ± 5.1 | 52.7 ± 5.8 | 54.7 ± 3.2 | 52.4 ± 5.5 | 51.8 ± 3.8 | 51.4 ± 5.2 |
Lateral | 55.5 ± 6.1 | 54.1 ± 8.2 | 56.6 ± 5.2 | 58.6 ± 7.1 | 57.6 ± 4.0 | 57.3 ± 6.9 | 55.0 ± 5.9 | 56.1 ± 5.4 |
Posterior | 60.5 ± 6.0 | 58.5 ± 7.7 | 61.2 ± 7.4 | 62.2 ± 6.5 | 63.0 ± 5.8 | 62.5 ± 6.0 | 59.5 ± 4.3 | 60.1 ± 4.4 |
Inferior | 56.3 ± 7.0 | 54.7 ± 6.9 | 57.5 ± 5.4 | 59.0 ± 6.4 | 60.3 ± 5.2 | 59.3 ± 6.5 | 57.8 ± 4.8 | 57.2 ± 3.8 |
Septal | 41.1 ± 5.0 | 42.9 ± 5.3 | 45.8 ± 4.9 | 50.3 ± 5.6 | 51.1 ± 3.7 | 49.6 ± 4.8 | 46.7 ± 5.2 | 45.4 ± 5.8 |
Global S-RM | 49.9 ± 4.3 ∗ | 50.0 ± 5.7 ∗ | 52.3 ± 4.5 ∗ | 54.9 ± 5.4 | 56.1 ± 3.8 ∗ | 54.9 ± 5.4 ∗ | 52.8 ± 4.1 | 52.2 ± 4.3 |
5th percentile global S-RM | 41.3 | 38.6 | 43.3 | 44.1 | 48.5 | 44.1 | 44.6 | 43.6 |
95th percentile global S-RM | 58.5 | 61.4 | 61.3 | 65.7 | 63.7 | 65.7 | 61.0 | 60.8 |
∗ P < .05 vs with age groups denoted by superscript numerals, determined by one-way analysis of variance with Bonferroni’s correction for multiple comparisons.
Age group | ||||||||
---|---|---|---|---|---|---|---|---|
1 | 2 | 3 | 4 | 5 | 6 | 7 | 8 | |
Variable | 0 y | 1–4 y | 5–9 y | 10–14 y | 15–19 y | 20–24 y | 25–29 y | 30–40 y |
PM | ||||||||
Anteroseptal | −25.2 ± 5.7 | −26.8 ± 3.6 | −26.3 ± 2.3 | −26.1 ± 3.0 | −27.5 ± 2.8 | −25.2 ± 2.1 | −24.7 ± 2.5 | −24.1 ± 2.0 |
Anterior | −18.2 ± 1.6 | −21.3 ± 4.1 | −24.4 ± 2.2 | −24.1 ± 2.0 | −22.9 ± 2.0 | −22.5 ± 1.7 | −20.9 ± 2.6 | −20.4 ± 2.3 |
Lateral | −13.8 ± 2.3 | −18.1 ± 2.0 | −20.5 ± 1.5 | −21.0 ± 1.7 | −21.1 ± 2.2 | −19.7 ± 1.5 | −18.6 ± 2.1 | −17.8 ± 2.0 |
Posterior | −10.7 ± 4.0 | −16.0 ± 1.9 | −19.4 ± 1.1 | −20.0 ± 1.4 | −19.2 ± 2.5 | −18.0 ± 1.6 | −18.0 ± 2.1 | −17.3 ± 2.4 |
Inferior | −17.0 ± 6.5 | −20.4 ± 2.0 | −22.7 ± 2.0 | −22.8 ± 1.7 | −22.4 ± 2.1 | −20.9 ± 1.9 | −19.6 ± 1.5 | −20.7 ± 2.8 |
Septal | −26.5 ± 6.0 | −25.3 ± 3.6 | −27.2 ± 2.6 | −26.9 ± 2.8 | −28.6 ± 2.5 | −24.8 ± 2.8 | −24.6 ± 3.2 | −23.5 ± 2.7 |
Global S-CP | −18.6 ± 3.3 ∗ | −21.3 ± 2.0 ∗ | −23.4 ± 1.7 ∗ | −23.5 ± 1.8 ∗ | −23.6 ± 2.0 ∗ | −21.8 ± 1.5 ∗ | −21.1 ± 1.9 ∗ | −20.6 ± 2.2 ∗ |
5th percentile global S-CP | −12.0 | −17.3 | −20.0 | −19.9 | −19.6 | −18.8 | −17.3 | −16.2 |
95th percentile global S-CP | −25.2 | −25.3 | −26.8 | −27.1 | −27.6 | −24.8 | −24.9 | −25.0 |
MV | ||||||||
Anteroseptal | −25.1 ± 3.8 | −27.1 ± 3.9 | −25.3 ± 3.8 | −24.3 ± 3.3 | −24.0 ± 2.5 | −24.8 ± 2.6 | −25.1 ± 4.1 | 23.2 ± 2.3 |
Anterior | −16.5 ± 4.2 | −20.3 ± 4.4 | −21.1 ± 2.1 | −21.8 ± 2.0 | −22.4 ± 1.9 | −20.8 ± 2.0 | −20.6 ± 1.5 | −19.7 ± 1.6 |
Lateral | −11.5 ± 3.6 | −15.4 ± 3.6 | −17.4 ± 2.9 | −19.2 ± 1.5 | −19.3 ± 2.4 | −18.2 ± 1.4 | −18.8 ± 1.2 | −18.3 ± 1.1 |
Posterior | −11.4 ± 4.0 | −12.4 ± 2.7 | −15.3 ± 2.9 | −18.2 ± 1.2 | −17.7 ± 2.0 | −18.2 ± 2.1 | −16.9 ± 2.0 | −16.2 ± 1.2 |
Inferior | −16.0 ± 7.6 | −17.8 ± 3.4 | −20.1 ± 2.1 | −20.7 ± 1.8 | −21.9 ± 2.7 | −20.3 ± 1.8 | −20.1 ± 1.6 | −20.2 ± 2.2 |
Septal | −24.5 ± 5.2 | −24.9 ± 4.7 | −26.4 ± 3.4 | −24.7 ± 2.3 | −26.0 ± 2.5 | −24.0 ± 2.2 | −24.3 ± 3.4 | −23.4 ± 3.9 |
Global S-CM | −17.5 ± 2.5 ∗ | −19.7 ± 2.0 ∗ | −20.9 ± 2.0 ∗ | −21.5 ± 1.7 ∗ | −21.9 ± 2.1 ∗ | −21.1 ± 1.3 ∗ | −21.0 ± 1.6 ∗ | −20.2 ± 1.4 ∗ |
5th percentile global S-CM | −12.5 | −15.7 | −16.9 | −18.1 | −17.7 | −18.5 | −17.8 | −17.4 |
95th percentile global S-CM | −22.5 | −23.7 | −24.9 | −24.9 | −26.1 | −23.7 | −24.2 | −23.0 |
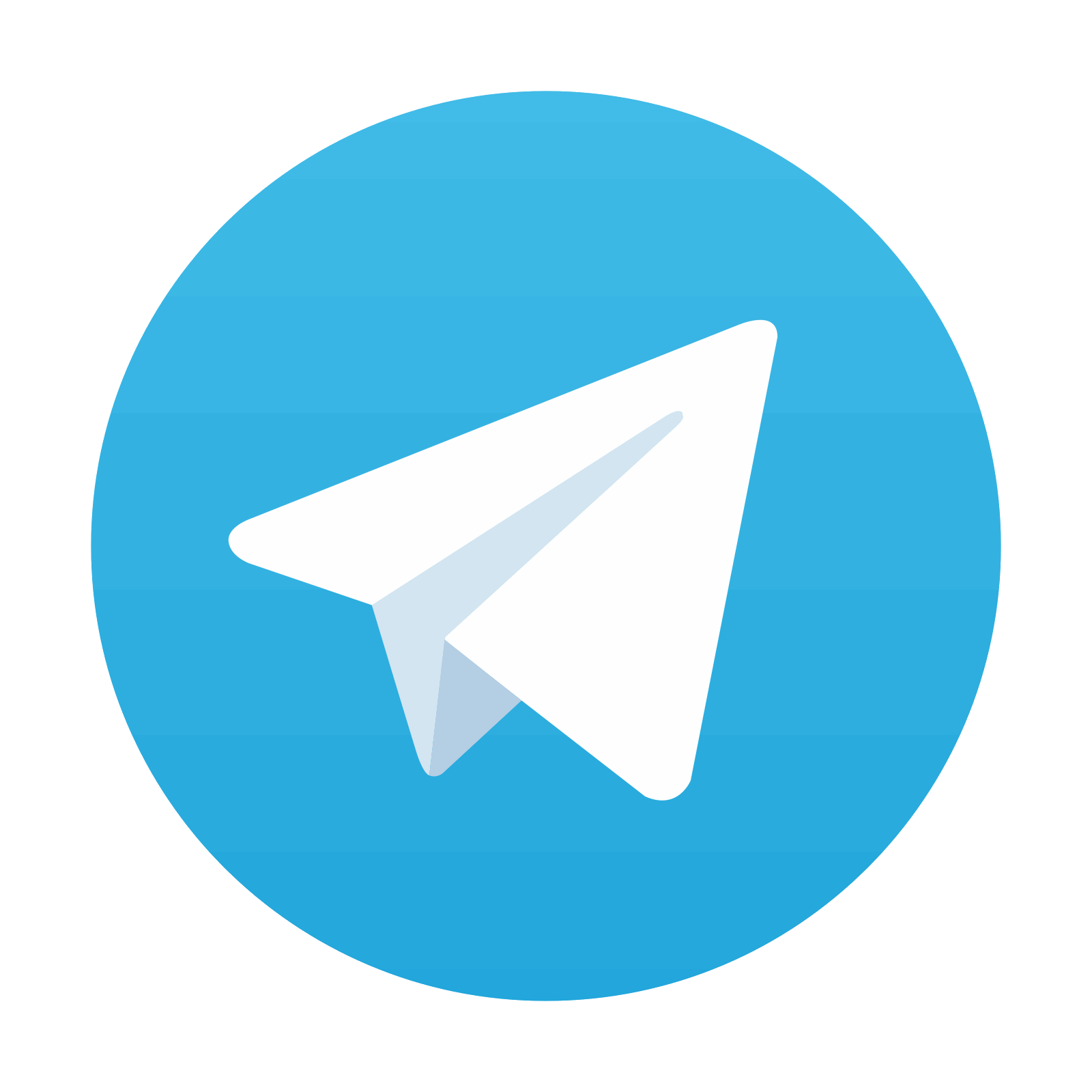
Stay updated, free articles. Join our Telegram channel

Full access? Get Clinical Tree
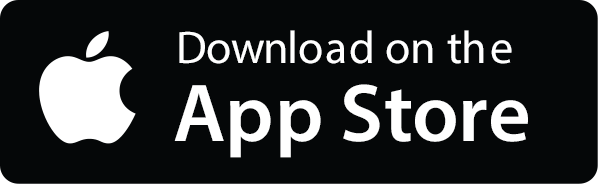
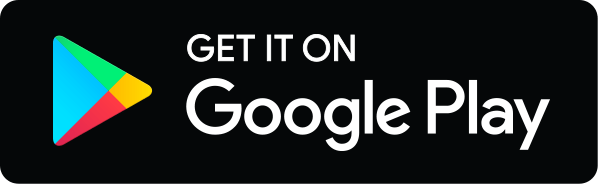
