Hypertension remains a major contributor to the global burden of disease. The measurement of blood pressure continues to have pitfalls related to both physiological aspects and acute variation. As the left ventricle (LV) remains one of the main target organs of hypertension, and echocardiographic measures of structure and function carry prognostic information in this setting, the development of a consensus position on the use of echocardiography in this setting is important. Recent developments in the assessment of LV hypertrophy and LV systolic and diastolic function have prompted the preparation of this document. The focus of this work is on the cardiovascular responses to hypertension rather than the diagnosis of secondary hypertension. Sections address the pathophysiology of the cardiac and vascular responses to hypertension, measurement of LV mass, geometry, and function, as well as effects of treatment.
Pathophysiology of Cardiac Responses to Hypertension
Left Ventricular Hypertrophy
Size and Geometry of the Normal Heart
The main contribution of echocardiography to the management of hypertension is the assessment of left ventricular (LV) mass (LVM). Body habitus represents one of several factors that confound the association between hypertension and LVM. However, cardiac size is influenced by body size, and for any given size, men have larger hearts than women, athletes have larger hearts than non-athletes, and obese subjects have larger hearts than non-obese subjects. LVM and volumes bear an approximately quadratic (rather than approximately cubic) relationship with height in men and women.
In the enlarged heart, wall (fibre) stress increases with LV size (radius and volume). This increase is compensated by a proportional increase of wall thickness, so that wall stress remains matched with the systolic pressure. The ‘relative’ geometry of the ventricle appears to be similar across species and body size, with normal relative wall thickness [RWT, the ratio of twice the posterior wall thickness (PW) and the LV diastolic diameter] from 0.32 to 0.42. Mass/volume ratios corresponding to the above-mentioned normal RWTs range between 1.1 and 1.3. RWT and M/V do not require correction for body size.
Effect of Gender
Data from several studies indicate that after adjustment for blood pressure and anthropometric parameters, LV volume and LVM are higher in men than in women. These differences persist when values of LVM are corrected for fat-free mass. This sex difference may explain the surprising lack of consensus in appropriate indexation of LVM, as it impacts the optimal method for indexing LVM for body height. Figure 1 displays LVM, calculated by the Devereux formula (unidimensional 2D measurements) in the healthy reference subgroup of the Asklepios population. Using the allometric index 1.7, the body height– LVM relationship in men (red) and women (blue) is parallel and indexation for body height is optimally achieved by height (ht) 1.7 in both sexes. However, when an allometric exponent is computed for males and females considered together (thick black line) without adjustment for gender, there is an exaggeration of nonlinearity in the height–LVM relationship (allometric index 2.7). This has important clinical and epidemiological implications, resulting in marked overestimation of the prevalence of LV hypertrophy in short subjects and a marked underestimation in tall subjects. The appropriate indexation remains an issue of contention.

Effect of Age
LV volumes are inversely associated with age. LVM decreases with age as well, albeit to a more limited extent than volume. As a consequence, RWT and M/V ratios increase. There is an age-related development of a concentric remodelling (see the Identification of LV Geometric Patterns section) with systolic and diastolic dysfunction.
Effect of Exercise and Sport
Isotonic exercise involves movement of large muscle groups. The profound vasodilatation of the skeletal muscle vasculature that is involved produces hypertrophy by increasing venous return to the heart and volume overload. This hypertrophy is characterized by chamber enlargement and a proportional change in wall thickness, with no changes in RWT. In contrast, isometric or static exercise involves developing muscular tension against resistance with little movement. Reflex and mechanical changes cause a pressure load on the heart rather than a volume load resulting in a slightly enlarged ventricle with increased RWT hypertrophy.
Effect of Obesity and Diabetes
Obesity is associated with increased LV volumes, increased LVM, and most typically increased RWT. In the Framingham study, an increase of body mass index over time was closely related to increased LVM and volumes. Insulin resistance, metabolic syndrome, and diabetes mellitus type II are similarly associated with increased LVM, RWT, and diastolic dysfunction. Diabetes patients have decreased systolic function as well. Correction of LVM for height preserves both the effects of obesity and elevated blood pressures on LVM. In contrast, correction of LVM for body surface area (BSA) effectively corrects for not only height but also obesity-related LV hypertrophy, which will remain undetected.
Inherited and Ethnic Contributions
Some of the variance in LV dimensions and mass may be explained by heredity, independent of the effects of sex, age, body size, blood pressure, heart rate, medications, and diabetes. Familial patterns of LV geometry were observed in subsequent generations of the Framingham study, but not in spouses. The greatest inheritable risk was found for concentric remodelling.
Normal ranges of LVM differ across races, being larger in African-Americans than in white Americans and/or Hispanics and smaller in Asian-Americans. Within one ethnicity, differences also exist between populations, e.g. Scandinavians being different from Mediterraneans. Only a part of these differences is accountable to ethnic variation in body size, and can be corrected by scaling. It is still unclear to what extent ethnic differences prevail when scaling for fat-free mass. It remains to be clarified to what extent these ethnic and population differences include a different prognosis and how to integrate ethnicities and populations in the definition of hypertrophy. At present, normal values and cutoffs should be adapted for each population.
LV Hypertrophy Due to Increased Load
Two basic patterns of cardiac hypertrophy occur in response to haemodynamic overload. In pressure overload (e.g. hypertension), pressure elevation most commonly leads to an increase in wall thickness and RWT, a phenomenon known as concentric remodelling (see the Identification of LV Geometric Patterns section). Eventually, an increase in systolic wall stress leads to concentric hypertrophy, caused by the addition of sarcomeres in parallel (hence, widening the cardiac myocytes), an increase in myocyte cross-sectional area, and an increase in LV wall thickening. In the Framingham Heart study, hypertensive patients had a greater increase in LVM and volume, and a smaller age-related reduction in LV size than individuals with normal blood pressure. In contrast, eccentric hypertrophy due to volume overload (e.g. with mitral regurgitation) is caused by increased diastolic wall stress. This leads to an increase in myocyte length with the addition of sarcomeres in series (hence, lengthening of cardiac myocytes), thereby engendering LV enlargement.
Adaptation of LV Function to Increased Load
The complex changes that occur in the heart during LV remodelling cause alterations in LV size and geometry, but the process of LV remodelling also leads to alterations in contraction and relaxation, the volume of myocyte and non-myocyte components of the myocardium, the properties of the myocyte (sarcomeres, e.g. titin), and the extracellular matrix (balance of collagen types I and III, and collagen fraction). Diastolic function is influenced by alterations in LV systolic function and geometry, delayed myocardial relaxation, increased passive stiffness of the sarcomere and extracellular matrix, and altered myocardial tone.
Cardiac myocyte hypertrophy leads to foetal gene reactivation and decreased expression of a number of genes normally expressed in the adult heart. Depending on age, sex, duration of hypertension, severity, and treatment, differing cellular and molecular events may underlie the evolution from a ventricle with concentric hypertrophy to a more dilated failing ventricle (often presenting as HFrEF, heart failure reduced ejection fraction) or to a heavily fibrotic and non-dilated ventricle (presenting as HFpEF, heart failure preserved ejection fraction), according to the three stages in the hypertrophic process (overload, hypertrophy, and failure). Physiological hypertrophy (growth, pregnancy, and exercise) is characterized by normal organization of cardiac structure and normal or enhanced cardiac function, whereas pathological hypertrophy is commonly associated with upregulation of foetal genes, fibrosis, cardiac dysfunction, and increased mortality. The continuous vs. intermittent nature of overload in the settings of pathological and physiological hypertrophy is unlikely to account for the differences in response. In contrast to early-systolic load, late-systolic load delays myocardial relaxation and induces more maladaptive hypertrophy.
Morphology of the Hypertensive Heart
LV Morphology
LV hypertrophy is defined on a normative basis; a definition based on 2 SD above the mean LVM in the general population will differ from a definition based on the healthy population without obesity or hypertension. Separate cutoffs are required for men and women. If LVM is corrected for BSA, it should be recognized that this corrects for obesity-related LVM, or for height. In the end-stage hypertensive heart, there is an increase in LV volumes and sphericity, a decrease in stroke volume, and finally a reduction in EF.
LA Morphology
Left atrial (LA) volume may be calculated by either area-length or modified Simpson’s methods, and is usually scaled for BSA and expressed in mL/m²; the normal range is up to and including 34 mL/m 2 . As with the LV, scaling by BSA corrects for an obesity-related increase in LA size that as a consequence will remain undetected. The LA is not symmetrical, and enlargement may occur non-uniformly, predominantly in one direction. Consequently, LA size is much better evaluated with 2D- or 3D-based LA volume rather than with M-mode. In hypertension and other situations where diastolic dysfunction occurs, reduction in early diastolic emptying is compensated by forceful atrial contraction. In addition, intermittent or permanent elevation of LV filling pressures leads to overfilling of the LA. The resulting LA enlargement is the ‘morpho-physiologic expression’ of chronic diastolic dysfunction, hypothesized to reflect the duration and severity of increased LA pressure. Although the presence of atrial fibrillation itself contributes to atrial size, LA enlargement is a well-known independent determinant of stroke, cardiovascular events, and death. Moreover, atrial fibrosis may be another endpoint of this process, predisposing to atrial remodelling and dysfunction with atrial fibrillation. This is a common endpoint that may be initiated by a number of aetiologies, including hypertension and diabetes mellitus.
The main determinants of an increasing atrial size with age are the cardiovascular risk factors of elevated blood pressure and obesity. In hypertensive patients, LA enlargement is related to LVM (rather than the type of LV hypertrophy), overweight, higher fasting glucose, and metabolic syndrome.
Measurement of LVM
Linear Echocardiographic Dimensions
Acquisition and Measurements
The measurement of LVM requires accurate measurements of wall thickness and chamber dimensions, as described in the Chamber Quantification update. The linear measurements of LV internal dimension (LVDd), septal (IVS), and PW are made from the parasternal long-axis acoustic window at the level of the LV minor axis, approximately at the mitral valve leaflet tips. M-mode recordings have excellent temporal resolution, and may be chosen from 2D images. However, even when directed by 2D guidance, it may not be possible to align the M-mode cursor perpendicular to the long axis of the ventricle ( Figure 2 ). Software has been developed to reconstruct anatomical M-mode images from 2D images ( Figure 3 ), but this is not yet universally available. Reference normal values for LV linear measurements are published in the Chamber Quantification update. Alternatively, chamber dimension and wall thicknesses can be acquired from the parasternal short-axis view using direct 2D measurements. The use of 2D-derived linear dimensions overcomes the common problem of oblique parasternal images resulting in overestimation of cavity and wall dimensions from M-mode ( Figure 4 ).
When 2D measurements are used, the wall thicknesses and linear dimensions should be measured at the level of the LV minor dimension, at the mitral leaflet tips level. The upper limit of normal for LVDd is smaller than the M-mode measurement. Left ventricle internal dimension diastole (LVIDd), inter-ventricular septum diastole (IVSd), and posterior wall diastole (PWd) are measured at end-diastole from 2D or M-mode recordings, preferably on several beats.
Understanding the LVM literature is facilitated by recognizing various methods:
- i.
The original American Society of Echocardiography (ASE) approach recommended that dimensions be measured from the leading edge to the leading edge of echocardiographic borders. This results in the inclusion of endocardial echoes from the IVS and PW, and the exclusion of endocardial echoes from the LVDd. This was because the trailing edge of endocardial signals is dependent on gain settings. This may impact on LVM measurements, especially at the upper and lower extremes of these measurements. The simplified calculation of LVM with this approach is LVM = 1.04[(IVS + LVDd + PW) 3 − (LVDd) 3 ] + 0.6 g.
- ii.
The subsequent Penn convention excluded endocardial echoes from IVS and PW dimensions, but included endocardial echoes in measurement of the LVDd. As the Penn convention gives larger cavity dimensions and smaller wall thicknesses than the ASE convention, the use of this approach necessitates subtraction of 13.6 from the previous mass calculation.
- iii.
The current ASE/European Association of Cardiovascular Imaging (EACVI) Chamber Quantitation Guidelines point out that refinements in image processing have allowed measurement of the actual visualized thickness of the ventricular septum and other chamber dimensions as defined by the actual tissue–blood interface, rather than the distance between the leading edge echoes, which had previously been recommended ( Figure 5 ).
Figure 5
Range of measurement options for measuring LVM.
All LVM algorithms (M-mode, 2D, or 3D echocardiographic measurements) are based on subtraction of the LV cavity volume from the volume enclosed by the LV epicardium to obtain the volume of the shell between the LV cavity and the epicardial surface. This shell volume is then converted to mass by multiplying LV wall volume by the specific gravity of myocardium (1.05 g/mL). The formula used for estimation of LVM from LV linear dimensions is based on modelling the LV as a prolate ellipse, and assumes that the major/minor axis ratio is 2 : 1: LVM = 0.8 × {1.04[(LVIDd + PW + IVSd) 3 − (LVIDd) 3 ]} + 0.6 g. Extensive validation of this formula has been performed from necropsy specimens.
Normal Values
Table 1 summarizes the reported range of normal values for LVM by M-mode echocardiography. These values differ between men and women, with the latter systematically lower than the former, even when indexed for BSA ( Table 1 ; see the section below—methods of indexation). The upper limits of normal ranges in the ASE chamber quantification update are >95 g/m 2 (>44 g/ht 2.7 ) in women and >115 g/m 2 (>48 g/ht 2.7 ) in men.
Source | Year | Men | Women | Age (years) | Body size indexation | Measurement convention | LVM | Upper limit of LVMI | Basis for upper limits | ||
---|---|---|---|---|---|---|---|---|---|---|---|
Men | Women | Men | Women | ||||||||
Henry et al. | 1980 | 78 | 58 | 20–97 | None | ASE | 160 ± 25 g (107 ± 17 g/m 2 ) | 210 g (140 g/m 2 ) | 95% CL | ||
Devereux et al. | 1981 | 106 | 120 | 39 ± 13 | BSA | Penn | 89 ± 21 | 69 ± 19 | 136 g/m 2 | 112 g/m 2 | 97th percentile |
Hammond et al. | 1984 | 83 | 77 | 44 ± 13 | BSA | Penn | 155 ± 50 g (Penn) 193 ± 55 g (ASE) 84 ± 23 g/m 2 (Penn) | – | 134 g/m | 110 g/m 2 | Comparison with hypertensive population: LV determination |
Byrd et al. | 1985 | 44 | 40 | 35 ± 10 | BSA | – | 148 ± 26 g 76 ± 13 g/m 2 | 108 ± 21 g 66 ± 11 g/m 2 | 200 g 102 g/m 2 | 150 g 88 g/m 2 | 95th percentile |
Levy et al. | 1987 | 347 | 50 | 43 ± 12 | Ht/BSA | ASE | 208 ± 43 g (ASE) 177 ± 41 g (Penn) | 145 ± 27 g (ASE) 118 ± 24 g (Penn) | 294 g 163 g/m 150 g/m 2 | 198 g 121 g/m 120 g/m 2 | M + 2 SD |
Koren et al. | 1991 | 167 | 86 | 47 ± 13 | BSA | Penn | – | – | 125 g/m 2 | 125 g/m 2 | CV risk at 10 years |
De Simone et al. | 1992 | 137 | 91 | 39 ± 14 | None Height Height 2.7 BSA | Penn Penn Penn Penn | 155 ± 34 g 89 ± 19 g/m 35 ± 8 g/m 2.7 89 ± 16 g/m 2 | 117 ± 28 g 72 ± 17 g/m 2.7 32 ± 8 g/m 2 73 ± 16 g/m 2 | 223 g 127 g/m 2.7 51 g/m 2 117 g/m 2 | 173 g 106 g/m 2 48 g/m 2 105 g/m 2 | M + 2 SD M + 2 SD M + 2 SD M + 2 SD |
Kuch et al. | 2000 | 213 | 291 | 42 ± 12 | Height Height 2.7 BSA FFM | ASE ASE ASE ASE | 97 ± 21 g/m 37 ± 8 g/m 2.7 89 ± 18 g/m 2 2.91 ± 0.59 g/kg | 71 ± 18 g/m 31 ± 8 g/m 2.7 70 ± 17 g/m 2 2.71 ± 0.70 g/kg | 139 g/m 53 g/m 2.7 135 g/m 2 4.09 g/kg | 107 g/m 47 g/m 2.7 104 g/m 2 4.11 g/kg | M + 2 SD M + 2 SD M + 2 SD M + 2 SD |
CV Health Study | 2001 | 651 | 1066 | 72 ± 5 (65–98) | None Height Height 2.7 BSA | ASE ASE ASE ASE | 166 ± 45 g 96 ± 27 g/m 37 ± 11 g/m 2.7 87 ± 24 g/m 2 | 127 ± 35 g 80 ± 22 g/m 36 ± 10 g/m 2.7 77 ± 19 g/m 2 | 256 g 150 g/m 59 g/m 2.7 135 g/m 2 | 197 g 124 g/m 56 g/m 2.7 115 g/m 2 | M + 2 SD M + 2 SD M + 2 SD M + 2 SD |
CV Health Study (Healthy Substudy) | 2013 | 93 | 213 | 75 ± 4 | None Height Height 2.7 BSA | ASE ASE ASE ASE | 146 ± 36 g 84 ± 20 g/m 33 ± 9 g/m 2.7 77 ± 19 g/m 2 | 121 ± 32 g 76 ± 20 g/m 34 ± 9 g/m 2.7 74 ± 19 g/m 2 | 218 g 124 g/m 51 g/m 2.7 115 g/m 2 | 185 g 116 g/m 52 g/m 2.7 114 g/m 2 | M + 2 SD M + 2 SD M + 2 SD M + 2 SD |
Asklepios—total population | 2007 | 1301 | 1223 | 46 (41–51) (35–55) | None Height 1.7 BSA | 2D 2D 2D | 175 ± 39 g 67 ± 15 g/m 1.7 87 ± 17 g/m 2 | 121 ± 30 g 53 ± 13 g/m 1.7 69 ± 14 g/m 2 | 243 g 92 g/m 1.7 116 g/m 2 | 177 g 77 g/m 1.7 94 g/m 2 | 95th percentile 95th percentile 95th percentile |
Asklepios Healthy, Risk factor deprived | 2007 | 198 | 414 | 43 (39–48) (35–55) | None Height 1.7 BSA | 2D 2D 2D | 155 ± 36 g 58 ± 13 g/m 1.7 82 ± 17 g/m 2 | 108 ± 21 g 46 ± 9 g/m 1.7 65 ± 11 g/m 2 | 214 g 81 g/m 1.7 112 g/m 2 | 143 g 60 g/m 1.7 86 g/m 2 | 95th percentile 95th percentile 95th percentile |
Limitations
There are four principal limitations in the calculation of LVM using linear methods:
- i.
The ‘Cube’ formula is not accurate in patients with major distortions of LV geometry (e.g. apical aneurysm, or any condition where the 2 : 1 axis ratio requirement is not met).
- ii.
Because this formula involves cubing primary measurements, even small errors in these measurements may be magnified.
- iii.
These measurements are insensitive to small changes in mass.
- iv.
The measurements are highly dependent on imaging quality and observer expertise.
Two-Dimensional Echocardiography
The most commonly used 2D methods for measuring LVM are based on the area-length formula and the truncated ellipsoid model, as described in detail in the previous ASE/EACVI chamber quantification document ( Figure 6 ). In the presence of shape distortions, such as that caused by post-myocardial infarction (MI) remodelling, the geometric assumptions inherent in this approach remain problematic. Both methods were validated in the early 1980s in animal models and by comparing premorbid echocardiograms with measured LV weight at autopsy in human beings. Normal values are summarized in Table 2 , and the degrees of abnormality are classified in Table 3 . The main limitations relate to image quality and the temporal resolution of 2D imaging, compared with M-mode echocardiography. The limitations of M-mode regarding geometrical assumptions and the impact of small error on measurements are also applicable to 2D measurements. In addition, 2D imaging leads to frequent foreshortening due to inappropriate cut-planes.
European | Japanese | |||
---|---|---|---|---|
Men | Women | Men | Women | |
2D LVM (g) | 96–200 | 66–150 | ||
2D LVM index (g/m 2 ) | 50–102 | 44–88 | ||
3D LVM (g) | ||||
3D LVM index (g/m 2 ) | 77 (57–97) | 74 (58–90) | 64 (40–88) | 56 (34–78) |
Women | Men | |||||||
---|---|---|---|---|---|---|---|---|
Reference range | Mildly abnormal | Moderately abnormal | Severely abnormal | Reference range | Mildly abnormal | Moderately abnormal | Severely abnormal | |
Linear method | ||||||||
LVM, g | 67–162 | 163–186 | 187–210 | ≥211 | 88–224 | 225–258 | 259–292 | ≥293 |
LVM/BSA, g/m 2 | 43 – 95 | 96 – 108 | 109 – 121 | ≥122 | 49 – 115 | 116 – 131 | 132 – 148 | ≥149 |
LVM/height, g/m | 41–99 | 100–115 | 116–128 | ≥129 | 52–126 | 127–144 | 145–162 | ≥163 |
LVM /height 2.7 , g/m 2.7 | 18–44 | 45–51 | 52–58 | ≥59 | 20–48 | 49–55 | 56–63 | ≥64 |
Relative wall thickness, cm | 0.22–0.42 | 0.43–0.47 | 0.48–0.52 | ≥0.53 | 0.24–0.42 | 0.43–0.46 | 0.47–0.51 | ≥0.52 |
Septal thickness, cm | 0.6 – 0.9 | 1.0 – 1.2 | 1.3 – 1.5 | ≥1.6 | 0.6 – 1.0 | 1.1 – 1.3 | 1.4 – 1.6 | ≥1.7 |
Posterior wall thickness, cm | 0.6 – 0.9 | 1.0 – 1.2 | 1.3 – 1.5 | ≥1.6 | 0.6 – 1.0 | 1.1 – 1.3 | 1.4 – 1.6 | ≥1.7 |
2D method | ||||||||
LVM, g | 66–150 | 151–171 | 172–182 | >193 | 96–200 | 201–227 | 228–254 | >255 |
LVM/BSA, g/m 2 | 44 – 88 | 89 – 100 | 101 – 112 | ≥113 | 50 – 102 | 103 – 116 | 117 – 130 | ≥131 |
Three-Dimensional Echocardiography
The benefit of three-dimensional echocardiography (3DE) is especially to obviate inaccurate geometric assumptions, inherent to 2DE, that become exaggerated in remodelled ventricles. 3DE is a potentially attractive modality for the measurement of LVM, and normal ranges have been developed. The accuracy of 3DE is reportedly similar to cardiac magnetic resonance (CMR) imaging methods for measuring LVM. However, there are wide limits of agreement which primarily relate to difficulties in accurately tracing the LV epicardial border, particularly in dilated ventricles, and generally show that while 3DE is imperfect for LVM estimation—with a tendency to underestimate LVM compared with CMR imaging in patients with cardiac disease—the accuracy is more favourable than with alternative ultrasound methods. Normal values of M-mode, 2D mass, and 3D mass are given in Tables 1 and 2 . Degrees of abnormality of LVM are summarized in Table 3 and the validation of all methods against reference techniques is summarized in Table 4 . A later section describes the use of 2D and 3D for the assessment of LV function.
LVM is prognostically important and should be reported in hypertensive patients.
In the normally shaped LV, either M-mode or 2DE formulas can be used to calculate LVM. The majority of community-acquired prognostic evidence has been gathered with M-mode imaging.
In laboratories that use 3DE routinely, 3D LVM measurement should be considered—especially in abnormally shaped ventricles or in individuals with asymmetric or localized hypertrophy. 3DE is the only echocardiographic technique that measures myocardial volume directly, without geometric assumptions about LV shape and distribution of wall thickening.
End-diastole | End-systole | |||||||
---|---|---|---|---|---|---|---|---|
r | SEE (g) | P -value | Regression equation | r | SEE (g) | P -value | Regression equation | |
1D Echo-Penn vs. CMR | 0.725 | 25.6 | .018 | 1D Echo-Penn = 0.99 (CMR) + 4.0 | 0.788 | 28.7 | .007 | 1D Echo-Penn = 1.35 (CMR) − 19.2 |
2D Echo-AL vs. CMR | 0.694 | 24.2 | .030 | 2D Echo-AL = 0.86 CMR) + 32.4 | 0.717 | 28.2 | .030 | 2D Echo-AL = 1.10 (CMR) + 14.1 |
2D Echo-TE vs. CMR | 0.687 | 21.8 | .030 | 2D Echo-TE = 0.76 (CMR) + 27.7 | 0.710 | 24.5 | .020 | 2D Echo-TE = 0.90 (CMR) + 13.0 |
3D Echo-PSR vs. CMR | 0.882 | 10.4 | .001 | 3D Echo-PSR = 0.72 (CMR) + 32.2 | 0.908 | 10.8 | .001 | 3D Echo-PSR = 0.86 (CMR) + 13.2 |
Measurement of LVM
Linear Echocardiographic Dimensions
Acquisition and Measurements
The measurement of LVM requires accurate measurements of wall thickness and chamber dimensions, as described in the Chamber Quantification update. The linear measurements of LV internal dimension (LVDd), septal (IVS), and PW are made from the parasternal long-axis acoustic window at the level of the LV minor axis, approximately at the mitral valve leaflet tips. M-mode recordings have excellent temporal resolution, and may be chosen from 2D images. However, even when directed by 2D guidance, it may not be possible to align the M-mode cursor perpendicular to the long axis of the ventricle ( Figure 2 ). Software has been developed to reconstruct anatomical M-mode images from 2D images ( Figure 3 ), but this is not yet universally available. Reference normal values for LV linear measurements are published in the Chamber Quantification update. Alternatively, chamber dimension and wall thicknesses can be acquired from the parasternal short-axis view using direct 2D measurements. The use of 2D-derived linear dimensions overcomes the common problem of oblique parasternal images resulting in overestimation of cavity and wall dimensions from M-mode ( Figure 4 ).
When 2D measurements are used, the wall thicknesses and linear dimensions should be measured at the level of the LV minor dimension, at the mitral leaflet tips level. The upper limit of normal for LVDd is smaller than the M-mode measurement. Left ventricle internal dimension diastole (LVIDd), inter-ventricular septum diastole (IVSd), and posterior wall diastole (PWd) are measured at end-diastole from 2D or M-mode recordings, preferably on several beats.
Understanding the LVM literature is facilitated by recognizing various methods:
- i.
The original American Society of Echocardiography (ASE) approach recommended that dimensions be measured from the leading edge to the leading edge of echocardiographic borders. This results in the inclusion of endocardial echoes from the IVS and PW, and the exclusion of endocardial echoes from the LVDd. This was because the trailing edge of endocardial signals is dependent on gain settings. This may impact on LVM measurements, especially at the upper and lower extremes of these measurements. The simplified calculation of LVM with this approach is LVM = 1.04[(IVS + LVDd + PW) 3 − (LVDd) 3 ] + 0.6 g.
- ii.
The subsequent Penn convention excluded endocardial echoes from IVS and PW dimensions, but included endocardial echoes in measurement of the LVDd. As the Penn convention gives larger cavity dimensions and smaller wall thicknesses than the ASE convention, the use of this approach necessitates subtraction of 13.6 from the previous mass calculation.
- iii.
The current ASE/European Association of Cardiovascular Imaging (EACVI) Chamber Quantitation Guidelines point out that refinements in image processing have allowed measurement of the actual visualized thickness of the ventricular septum and other chamber dimensions as defined by the actual tissue–blood interface, rather than the distance between the leading edge echoes, which had previously been recommended ( Figure 5 ).
Figure 5
Range of measurement options for measuring LVM.
All LVM algorithms (M-mode, 2D, or 3D echocardiographic measurements) are based on subtraction of the LV cavity volume from the volume enclosed by the LV epicardium to obtain the volume of the shell between the LV cavity and the epicardial surface. This shell volume is then converted to mass by multiplying LV wall volume by the specific gravity of myocardium (1.05 g/mL). The formula used for estimation of LVM from LV linear dimensions is based on modelling the LV as a prolate ellipse, and assumes that the major/minor axis ratio is 2 : 1: LVM = 0.8 × {1.04[(LVIDd + PW + IVSd) 3 − (LVIDd) 3 ]} + 0.6 g. Extensive validation of this formula has been performed from necropsy specimens.
Normal Values
Table 1 summarizes the reported range of normal values for LVM by M-mode echocardiography. These values differ between men and women, with the latter systematically lower than the former, even when indexed for BSA ( Table 1 ; see the section below—methods of indexation). The upper limits of normal ranges in the ASE chamber quantification update are >95 g/m 2 (>44 g/ht 2.7 ) in women and >115 g/m 2 (>48 g/ht 2.7 ) in men.
Source | Year | Men | Women | Age (years) | Body size indexation | Measurement convention | LVM | Upper limit of LVMI | Basis for upper limits | ||
---|---|---|---|---|---|---|---|---|---|---|---|
Men | Women | Men | Women | ||||||||
Henry et al. | 1980 | 78 | 58 | 20–97 | None | ASE | 160 ± 25 g (107 ± 17 g/m 2 ) | 210 g (140 g/m 2 ) | 95% CL | ||
Devereux et al. | 1981 | 106 | 120 | 39 ± 13 | BSA | Penn | 89 ± 21 | 69 ± 19 | 136 g/m 2 | 112 g/m 2 | 97th percentile |
Hammond et al. | 1984 | 83 | 77 | 44 ± 13 | BSA | Penn | 155 ± 50 g (Penn) 193 ± 55 g (ASE) 84 ± 23 g/m 2 (Penn) | – | 134 g/m | 110 g/m 2 | Comparison with hypertensive population: LV determination |
Byrd et al. | 1985 | 44 | 40 | 35 ± 10 | BSA | – | 148 ± 26 g 76 ± 13 g/m 2 | 108 ± 21 g 66 ± 11 g/m 2 | 200 g 102 g/m 2 | 150 g 88 g/m 2 | 95th percentile |
Levy et al. | 1987 | 347 | 50 | 43 ± 12 | Ht/BSA | ASE | 208 ± 43 g (ASE) 177 ± 41 g (Penn) | 145 ± 27 g (ASE) 118 ± 24 g (Penn) | 294 g 163 g/m 150 g/m 2 | 198 g 121 g/m 120 g/m 2 | M + 2 SD |
Koren et al. | 1991 | 167 | 86 | 47 ± 13 | BSA | Penn | – | – | 125 g/m 2 | 125 g/m 2 | CV risk at 10 years |
De Simone et al. | 1992 | 137 | 91 | 39 ± 14 | None Height Height 2.7 BSA | Penn Penn Penn Penn | 155 ± 34 g 89 ± 19 g/m 35 ± 8 g/m 2.7 89 ± 16 g/m 2 | 117 ± 28 g 72 ± 17 g/m 2.7 32 ± 8 g/m 2 73 ± 16 g/m 2 | 223 g 127 g/m 2.7 51 g/m 2 117 g/m 2 | 173 g 106 g/m 2 48 g/m 2 105 g/m 2 | M + 2 SD M + 2 SD M + 2 SD M + 2 SD |
Kuch et al. | 2000 | 213 | 291 | 42 ± 12 | Height Height 2.7 BSA FFM | ASE ASE ASE ASE | 97 ± 21 g/m 37 ± 8 g/m 2.7 89 ± 18 g/m 2 2.91 ± 0.59 g/kg | 71 ± 18 g/m 31 ± 8 g/m 2.7 70 ± 17 g/m 2 2.71 ± 0.70 g/kg | 139 g/m 53 g/m 2.7 135 g/m 2 4.09 g/kg | 107 g/m 47 g/m 2.7 104 g/m 2 4.11 g/kg | M + 2 SD M + 2 SD M + 2 SD M + 2 SD |
CV Health Study | 2001 | 651 | 1066 | 72 ± 5 (65–98) | None Height Height 2.7 BSA | ASE ASE ASE ASE | 166 ± 45 g 96 ± 27 g/m 37 ± 11 g/m 2.7 87 ± 24 g/m 2 | 127 ± 35 g 80 ± 22 g/m 36 ± 10 g/m 2.7 77 ± 19 g/m 2 | 256 g 150 g/m 59 g/m 2.7 135 g/m 2 | 197 g 124 g/m 56 g/m 2.7 115 g/m 2 | M + 2 SD M + 2 SD M + 2 SD M + 2 SD |
CV Health Study (Healthy Substudy) | 2013 | 93 | 213 | 75 ± 4 | None Height Height 2.7 BSA | ASE ASE ASE ASE | 146 ± 36 g 84 ± 20 g/m 33 ± 9 g/m 2.7 77 ± 19 g/m 2 | 121 ± 32 g 76 ± 20 g/m 34 ± 9 g/m 2.7 74 ± 19 g/m 2 | 218 g 124 g/m 51 g/m 2.7 115 g/m 2 | 185 g 116 g/m 52 g/m 2.7 114 g/m 2 | M + 2 SD M + 2 SD M + 2 SD M + 2 SD |
Asklepios—total population | 2007 | 1301 | 1223 | 46 (41–51) (35–55) | None Height 1.7 BSA | 2D 2D 2D | 175 ± 39 g 67 ± 15 g/m 1.7 87 ± 17 g/m 2 | 121 ± 30 g 53 ± 13 g/m 1.7 69 ± 14 g/m 2 | 243 g 92 g/m 1.7 116 g/m 2 | 177 g 77 g/m 1.7 94 g/m 2 | 95th percentile 95th percentile 95th percentile |
Asklepios Healthy, Risk factor deprived | 2007 | 198 | 414 | 43 (39–48) (35–55) | None Height 1.7 BSA | 2D 2D 2D | 155 ± 36 g 58 ± 13 g/m 1.7 82 ± 17 g/m 2 | 108 ± 21 g 46 ± 9 g/m 1.7 65 ± 11 g/m 2 | 214 g 81 g/m 1.7 112 g/m 2 | 143 g 60 g/m 1.7 86 g/m 2 | 95th percentile 95th percentile 95th percentile |
Limitations
There are four principal limitations in the calculation of LVM using linear methods:
- i.
The ‘Cube’ formula is not accurate in patients with major distortions of LV geometry (e.g. apical aneurysm, or any condition where the 2 : 1 axis ratio requirement is not met).
- ii.
Because this formula involves cubing primary measurements, even small errors in these measurements may be magnified.
- iii.
These measurements are insensitive to small changes in mass.
- iv.
The measurements are highly dependent on imaging quality and observer expertise.
Two-Dimensional Echocardiography
The most commonly used 2D methods for measuring LVM are based on the area-length formula and the truncated ellipsoid model, as described in detail in the previous ASE/EACVI chamber quantification document ( Figure 6 ). In the presence of shape distortions, such as that caused by post-myocardial infarction (MI) remodelling, the geometric assumptions inherent in this approach remain problematic. Both methods were validated in the early 1980s in animal models and by comparing premorbid echocardiograms with measured LV weight at autopsy in human beings. Normal values are summarized in Table 2 , and the degrees of abnormality are classified in Table 3 . The main limitations relate to image quality and the temporal resolution of 2D imaging, compared with M-mode echocardiography. The limitations of M-mode regarding geometrical assumptions and the impact of small error on measurements are also applicable to 2D measurements. In addition, 2D imaging leads to frequent foreshortening due to inappropriate cut-planes.
European | Japanese | |||
---|---|---|---|---|
Men | Women | Men | Women | |
2D LVM (g) | 96–200 | 66–150 | ||
2D LVM index (g/m 2 ) | 50–102 | 44–88 | ||
3D LVM (g) | ||||
3D LVM index (g/m 2 ) | 77 (57–97) | 74 (58–90) | 64 (40–88) | 56 (34–78) |
Women | Men | |||||||
---|---|---|---|---|---|---|---|---|
Reference range | Mildly abnormal | Moderately abnormal | Severely abnormal | Reference range | Mildly abnormal | Moderately abnormal | Severely abnormal | |
Linear method | ||||||||
LVM, g | 67–162 | 163–186 | 187–210 | ≥211 | 88–224 | 225–258 | 259–292 | ≥293 |
LVM/BSA, g/m 2 | 43 – 95 | 96 – 108 | 109 – 121 | ≥122 | 49 – 115 | 116 – 131 | 132 – 148 | ≥149 |
LVM/height, g/m | 41–99 | 100–115 | 116–128 | ≥129 | 52–126 | 127–144 | 145–162 | ≥163 |
LVM /height 2.7 , g/m 2.7 | 18–44 | 45–51 | 52–58 | ≥59 | 20–48 | 49–55 | 56–63 | ≥64 |
Relative wall thickness, cm | 0.22–0.42 | 0.43–0.47 | 0.48–0.52 | ≥0.53 | 0.24–0.42 | 0.43–0.46 | 0.47–0.51 | ≥0.52 |
Septal thickness, cm | 0.6 – 0.9 | 1.0 – 1.2 | 1.3 – 1.5 | ≥1.6 | 0.6 – 1.0 | 1.1 – 1.3 | 1.4 – 1.6 | ≥1.7 |
Posterior wall thickness, cm | 0.6 – 0.9 | 1.0 – 1.2 | 1.3 – 1.5 | ≥1.6 | 0.6 – 1.0 | 1.1 – 1.3 | 1.4 – 1.6 | ≥1.7 |
2D method | ||||||||
LVM, g | 66–150 | 151–171 | 172–182 | >193 | 96–200 | 201–227 | 228–254 | >255 |
LVM/BSA, g/m 2 | 44 – 88 | 89 – 100 | 101 – 112 | ≥113 | 50 – 102 | 103 – 116 | 117 – 130 | ≥131 |
Three-Dimensional Echocardiography
The benefit of three-dimensional echocardiography (3DE) is especially to obviate inaccurate geometric assumptions, inherent to 2DE, that become exaggerated in remodelled ventricles. 3DE is a potentially attractive modality for the measurement of LVM, and normal ranges have been developed. The accuracy of 3DE is reportedly similar to cardiac magnetic resonance (CMR) imaging methods for measuring LVM. However, there are wide limits of agreement which primarily relate to difficulties in accurately tracing the LV epicardial border, particularly in dilated ventricles, and generally show that while 3DE is imperfect for LVM estimation—with a tendency to underestimate LVM compared with CMR imaging in patients with cardiac disease—the accuracy is more favourable than with alternative ultrasound methods. Normal values of M-mode, 2D mass, and 3D mass are given in Tables 1 and 2 . Degrees of abnormality of LVM are summarized in Table 3 and the validation of all methods against reference techniques is summarized in Table 4 . A later section describes the use of 2D and 3D for the assessment of LV function.
LVM is prognostically important and should be reported in hypertensive patients.
In the normally shaped LV, either M-mode or 2DE formulas can be used to calculate LVM. The majority of community-acquired prognostic evidence has been gathered with M-mode imaging.
In laboratories that use 3DE routinely, 3D LVM measurement should be considered—especially in abnormally shaped ventricles or in individuals with asymmetric or localized hypertrophy. 3DE is the only echocardiographic technique that measures myocardial volume directly, without geometric assumptions about LV shape and distribution of wall thickening.
End-diastole | End-systole | |||||||
---|---|---|---|---|---|---|---|---|
r | SEE (g) | P -value | Regression equation | r | SEE (g) | P -value | Regression equation | |
1D Echo-Penn vs. CMR | 0.725 | 25.6 | .018 | 1D Echo-Penn = 0.99 (CMR) + 4.0 | 0.788 | 28.7 | .007 | 1D Echo-Penn = 1.35 (CMR) − 19.2 |
2D Echo-AL vs. CMR | 0.694 | 24.2 | .030 | 2D Echo-AL = 0.86 CMR) + 32.4 | 0.717 | 28.2 | .030 | 2D Echo-AL = 1.10 (CMR) + 14.1 |
2D Echo-TE vs. CMR | 0.687 | 21.8 | .030 | 2D Echo-TE = 0.76 (CMR) + 27.7 | 0.710 | 24.5 | .020 | 2D Echo-TE = 0.90 (CMR) + 13.0 |
3D Echo-PSR vs. CMR | 0.882 | 10.4 | .001 | 3D Echo-PSR = 0.72 (CMR) + 32.2 | 0.908 | 10.8 | .001 | 3D Echo-PSR = 0.86 (CMR) + 13.2 |
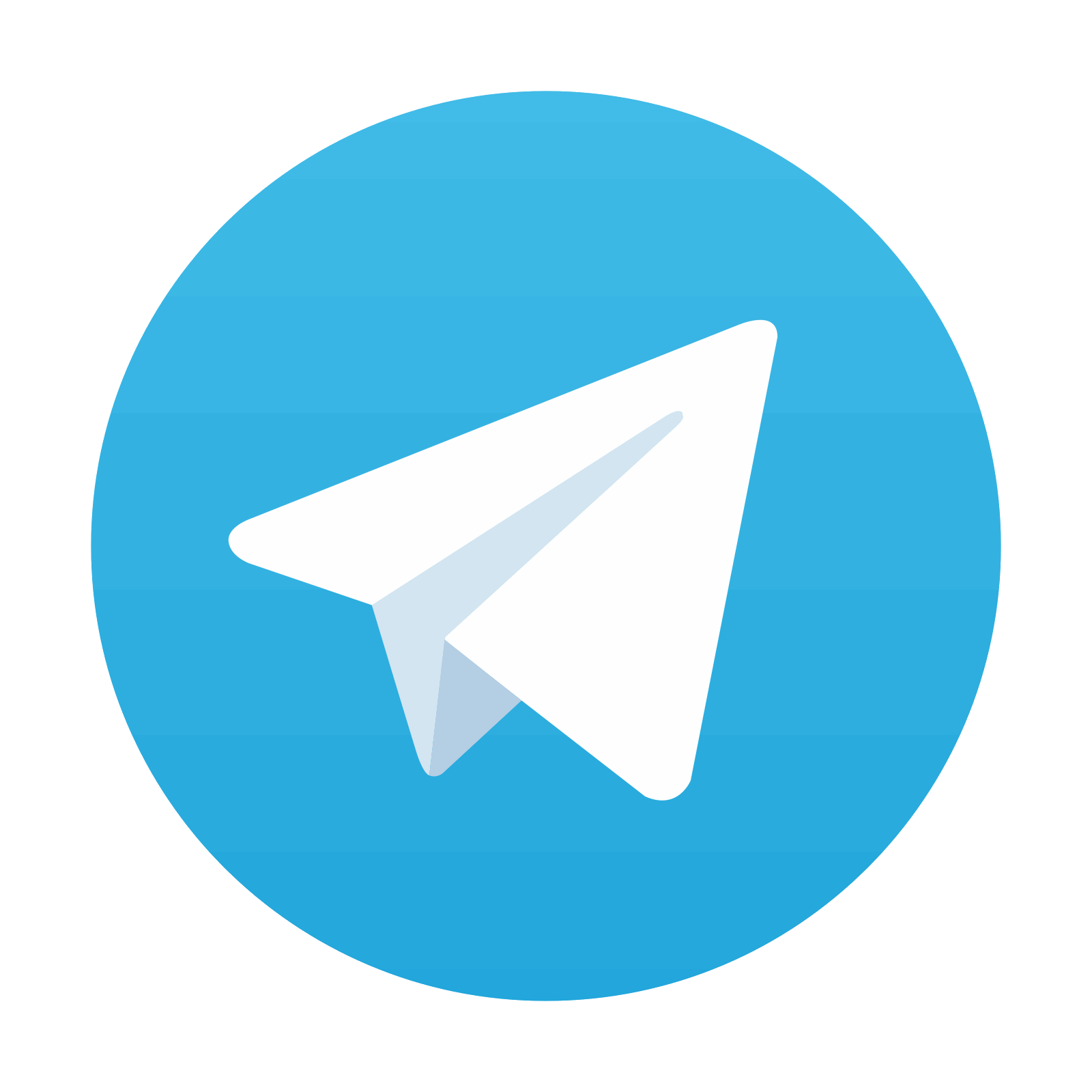
Stay updated, free articles. Join our Telegram channel

Full access? Get Clinical Tree
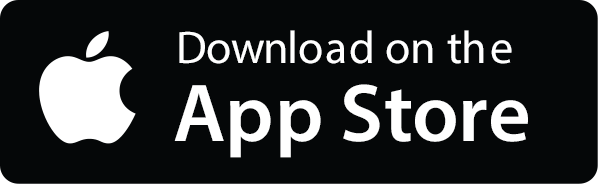
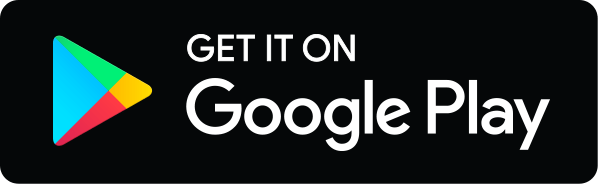
