Echocardiography is the key tool for the diagnosis and evaluation of aortic stenosis. Because clinical decision-making is based on the echocardiographic assessment of its severity, it is essential that standards are adopted to maintain accuracy and consistency across echocardiographic laboratories. Detailed recommendations for the echocardiographic assessment of valve stenosis were published by the European Association of Echocardiography and the American Society of Echocardiography in 2009. In the meantime, numerous new studies on aortic stenosis have been published with particular new insights into the difficult subgroup of low gradient aortic stenosis making an update of recommendations necessary. The document focuses in particular on the optimization of left ventricular outflow tract assessment, low flow, low gradient aortic stenosis with preserved ejection fraction, a new classification of aortic stenosis by gradient, flow and ejection fraction, and a grading algorithm for an integrated and stepwise approach of aortic stenosis assessment in clinical practice.
Introduction
Attention ASE Members:
ASE has gone green! Visit www.aseuniversity.org to earn free continuing medical education credit through an online activity related to this article. Certificates are available for immediate access upon successful completion of the activity. Nonmembers will need to join the ASE to access this great member benefit!
Aortic stenosis (AS) has become the most common primary heart valve disease and an important cause of cardiovascular morbidity and mortality. Echocardiography is the key tool for the diagnosis and evaluation of AS, and is the primary non-invasive imaging method for AS assessment. Diagnostic cardiac catheterization is no longer recommended except in rare cases when echocardiography is non-diagnostic or discrepant with clinical data.
Because clinical decision-making is based on the echocardiographic assessment of the severity of AS, it is essential that standards be adopted to maintain accuracy and consistency across echocardiographic laboratories when assessing and reporting AS. Recommendations for the echocardiographic assessment of valve stenosis in clinical practice were published by the European Association of Echocardiography and the American Society of Echocardiography in 2009. The aim of the 2009 paper was to detail the recommended approach to the echocardiographic evaluation of valve stenosis, including recommendations for specific measures of stenosis severity, details of data acquisition and measurement, and grading of severity. These 2009 recommendations were based on the scientific literature and on the consensus of a panel of experts. Since publication of this 2009 document, numerous new studies on AS have been published, in particular with new insights into the difficult subgroup of low gradient AS. Accordingly, a focused update on the echocardiographic assessment of AS appeared to be a needed document and is now provided with this document.
As with the 2009 document, this document discusses a number of proposed methods for evaluation of stenosis severity. On the basis of an updated comprehensive literature review and expert consensus, these methods were categorized for clinical practice as:
- •
Level 1 Recommendation: an appropriate and recommended method for all patients with aortic stenosis.
- •
Level 2 Recommendation: a reasonable method for clinical use when additional information is needed in selected patients.
- •
Level 3 Recommendation: a method not recommended for routine clinical practice although it may be appropriate for research applications and in rare clinical cases.
It is essential in clinical practice to use an integrative approach when grading the severity of AS, combining all Doppler and 2D data as well as clinical presentation, and not relying on one specific measurement. Loading conditions influence velocity and pressure gradients; therefore, these parameters vary depending on intercurrent illness of patients with low vs. high cardiac output. In addition, irregular rhythms or tachycardia can make assessment of AS severity challenging. Ideally, heart rate, rhythm, and blood pressure should be stated in the echocardiographic report and hemodynamic assessment should be performed at heart rates and blood pressures within the normal range. These guidelines provide recommendations for recording and measurement of AS severity using echocardiography. However, although accurate quantification of disease severity is an essential step in patient management, clinical decision-making depends on several other factors, most importantly, whether or not symptoms are present. This document is meant to provide echocardiographic standards and does not make recommendations for clinical management. The latter are detailed in the current guidelines for management of adults with heart valve disease.
Highlights in this focused update on aortic stenosis document include:
- •
Optimization of LVOT assessment.
- •
Low flow, low gradient aortic stenosis with reduced LVEF.
- •
Low flow, low gradient aortic stenosis with preserved LVEF.
- •
New classification of AS by gradient, flow and ejection fraction.
- •
AS grading algorithm- an integrated and stepwise approach.
Etiologies and Morphologic Assessment
The most common causes of valvular AS are calcific stenosis of a tricuspid valve, a bicuspid aortic valve with superimposed calcific changes, and rheumatic valve disease ( Figure 1 ). Congenital aortic stenosis owing to a unicuspid aortic valve is rare in adults with usually marked dysmorphic features including severe thickening and calcification and associated with significant concomitant aortic regurgitation (AR). In Europe and North America, calcific AS represents by far the most frequent aetiology with the prevalence of bicuspid vs. tricuspid aortic valves as underlying anatomy being highly age dependent. While tricuspid valves predominate in the elderly (>75 years) bicuspid valves are more common in younger patients (age < 65 years). While rheumatic AS has become rare in Europe and North America, it is still prevalent worldwide.

Anatomic evaluation of the aortic valve is based on a combination of short- and long-axis images to identify the number of cusps, and to describe cusp mobility, thickness, and calcification. In addition, the combination of imaging and Doppler allows the determination of the level of obstruction: subvalvular, valvular, or supravalvular. Transthoracic imaging is usually adequate, although transesophageal echocardiography (TEE) may be helpful when image quality is suboptimal.
A bicuspid valve most often results from fusion of the right and left coronary cusps, resulting in a larger anterior and smaller posterior cusp with both coronary arteries arising from the anterior cusp (∼80% of cases). Fusion of the right and non-coronary cusps resulting in larger right than left cusp, with one coronary artery arising from each cusp is less common (∼20% of cases). Fusion of the left and non-coronary cusps and valves with two equally sized cusps (“true” bicuspid valve) are rare. Diagnosis is most reliable when the two cusps are seen in systole with only two commissures framing an elliptical systolic orifice. Diastolic images may mimic three cusps when a raphe is present. Long-axis views may show an asymmetric closure line, systolic doming, or diastolic prolapse of one or both of the cusps, but these findings are less specific than a short-axis systolic image. In children, adolescents and young adults, a bicuspid valve may be stenotic without extensive calcification. However, in most adults, stenosis of a bicuspid aortic valve typically results from superimposed calcific changes, which often obscures the number of cusps, making determination of bicuspid vs. tricuspid valve difficult. Geometry and dilatation of the aortic root and ascending aorta may provide indirect hints that a bicuspid valve may be present.
Calcification of a tricuspid aortic valve is most prominent in the central and basal parts of each cusp while commissural fusion is absent, resulting in a stellate-shaped systolic orifice. Calcification of a bicuspid valve is often more asymmetric. The severity of valve calcification can be graded semi-quantitatively, as mild (few areas of dense echogenicity with little acoustic shadowing), moderate (multiple larger areas of dense echogenicity), or severe (extensive thickening and increased echogenicity with a prominent acoustic shadow). The degree of valve calcification is a predictor of clinical outcome including heart failure, need for aortic valve replacement and death. Radiation induced aortic stenosis represents a special challenge as the aortic valve is often heavily calcified in a younger population making the assessment of aortic valve morphology and LVOT diameter difficult.
Rheumatic AS is characterized by commissural fusion, resulting in a triangular systolic orifice, with thickening and calcification most prominent along the edges of the cusps. Rheumatic disease nearly always affects the mitral valve too, so that rheumatic aortic valve disease is accompanied by rheumatic mitral valve changes.
Subvalvular and supravalvular stenosis are distinguished from valvular stenosis based on the site of the increase in velocity seen with colour or pulsed Doppler and on the anatomy of the outflow tract and aorta, respectively. Subvalvular obstruction may be fixed, owing to a discrete membrane or muscular band, with haemodynamics similar to obstruction at the valvular level. Dynamic subaortic obstruction, for example, with hypertrophic cardiomyopathy, refers to obstruction that changes in severity during ventricular ejection, with obstruction developing predominantly in mid-to-late systole, resulting in a late peaking velocity curve. Dynamic obstruction also varies with loading conditions, with increased obstruction when ventricular volumes are smaller and when ventricular contractility is increased.
Supravalvular stenosis is uncommon and typically results from a congenital condition, such as Williams syndrome with persistent or recurrent obstruction in adulthood. In supravalvular stenosis flow acceleration is noted above the valve which confirms the morphologic suspicion of a narrowing typically at the sinotubular junction with or without extension into the ascending aorta.
With the advent of percutaneous aortic valve implantation, anatomic assessment has become increasingly important for patient selection and planning of the intervention. Besides underlying morphology (bicuspid vs. tricuspid) as well as extent and distribution of calcification, the assessment of annulus dimension is critical for the choice of prosthesis size. For the latter, 2D/3D TEE is superior to transthoracic echocardiography (TTE). Because multi-slice computed tomography (MSCT) has not only been shown to provide measurements of the annulus size with high accuracy, but also provides a comprehensive pre-procedural evaluation including aortic root shape, distance between coronary arteries and annulus, and anatomic details of the entire catheter route, it is frequently used now for this purpose. Thus, in cases when computed tomography is performed it may not be necessary to undergo TEE. Nevertheless, accurate measurements of the aortic valve annulus can also be made by 3D-TEE. Moreover, CT may not be feasible in patients who have renal insufficiency and TEE is a reliable alternative in such patients. Pre-interventional evaluation and echocardiographic monitoring of aortic valve intervention are not part of this focused update and are covered in separate documents.
Etiologies and Morphologic Assessment
The most common causes of valvular AS are calcific stenosis of a tricuspid valve, a bicuspid aortic valve with superimposed calcific changes, and rheumatic valve disease ( Figure 1 ). Congenital aortic stenosis owing to a unicuspid aortic valve is rare in adults with usually marked dysmorphic features including severe thickening and calcification and associated with significant concomitant aortic regurgitation (AR). In Europe and North America, calcific AS represents by far the most frequent aetiology with the prevalence of bicuspid vs. tricuspid aortic valves as underlying anatomy being highly age dependent. While tricuspid valves predominate in the elderly (>75 years) bicuspid valves are more common in younger patients (age < 65 years). While rheumatic AS has become rare in Europe and North America, it is still prevalent worldwide.
Anatomic evaluation of the aortic valve is based on a combination of short- and long-axis images to identify the number of cusps, and to describe cusp mobility, thickness, and calcification. In addition, the combination of imaging and Doppler allows the determination of the level of obstruction: subvalvular, valvular, or supravalvular. Transthoracic imaging is usually adequate, although transesophageal echocardiography (TEE) may be helpful when image quality is suboptimal.
A bicuspid valve most often results from fusion of the right and left coronary cusps, resulting in a larger anterior and smaller posterior cusp with both coronary arteries arising from the anterior cusp (∼80% of cases). Fusion of the right and non-coronary cusps resulting in larger right than left cusp, with one coronary artery arising from each cusp is less common (∼20% of cases). Fusion of the left and non-coronary cusps and valves with two equally sized cusps (“true” bicuspid valve) are rare. Diagnosis is most reliable when the two cusps are seen in systole with only two commissures framing an elliptical systolic orifice. Diastolic images may mimic three cusps when a raphe is present. Long-axis views may show an asymmetric closure line, systolic doming, or diastolic prolapse of one or both of the cusps, but these findings are less specific than a short-axis systolic image. In children, adolescents and young adults, a bicuspid valve may be stenotic without extensive calcification. However, in most adults, stenosis of a bicuspid aortic valve typically results from superimposed calcific changes, which often obscures the number of cusps, making determination of bicuspid vs. tricuspid valve difficult. Geometry and dilatation of the aortic root and ascending aorta may provide indirect hints that a bicuspid valve may be present.
Calcification of a tricuspid aortic valve is most prominent in the central and basal parts of each cusp while commissural fusion is absent, resulting in a stellate-shaped systolic orifice. Calcification of a bicuspid valve is often more asymmetric. The severity of valve calcification can be graded semi-quantitatively, as mild (few areas of dense echogenicity with little acoustic shadowing), moderate (multiple larger areas of dense echogenicity), or severe (extensive thickening and increased echogenicity with a prominent acoustic shadow). The degree of valve calcification is a predictor of clinical outcome including heart failure, need for aortic valve replacement and death. Radiation induced aortic stenosis represents a special challenge as the aortic valve is often heavily calcified in a younger population making the assessment of aortic valve morphology and LVOT diameter difficult.
Rheumatic AS is characterized by commissural fusion, resulting in a triangular systolic orifice, with thickening and calcification most prominent along the edges of the cusps. Rheumatic disease nearly always affects the mitral valve too, so that rheumatic aortic valve disease is accompanied by rheumatic mitral valve changes.
Subvalvular and supravalvular stenosis are distinguished from valvular stenosis based on the site of the increase in velocity seen with colour or pulsed Doppler and on the anatomy of the outflow tract and aorta, respectively. Subvalvular obstruction may be fixed, owing to a discrete membrane or muscular band, with haemodynamics similar to obstruction at the valvular level. Dynamic subaortic obstruction, for example, with hypertrophic cardiomyopathy, refers to obstruction that changes in severity during ventricular ejection, with obstruction developing predominantly in mid-to-late systole, resulting in a late peaking velocity curve. Dynamic obstruction also varies with loading conditions, with increased obstruction when ventricular volumes are smaller and when ventricular contractility is increased.
Supravalvular stenosis is uncommon and typically results from a congenital condition, such as Williams syndrome with persistent or recurrent obstruction in adulthood. In supravalvular stenosis flow acceleration is noted above the valve which confirms the morphologic suspicion of a narrowing typically at the sinotubular junction with or without extension into the ascending aorta.
With the advent of percutaneous aortic valve implantation, anatomic assessment has become increasingly important for patient selection and planning of the intervention. Besides underlying morphology (bicuspid vs. tricuspid) as well as extent and distribution of calcification, the assessment of annulus dimension is critical for the choice of prosthesis size. For the latter, 2D/3D TEE is superior to transthoracic echocardiography (TTE). Because multi-slice computed tomography (MSCT) has not only been shown to provide measurements of the annulus size with high accuracy, but also provides a comprehensive pre-procedural evaluation including aortic root shape, distance between coronary arteries and annulus, and anatomic details of the entire catheter route, it is frequently used now for this purpose. Thus, in cases when computed tomography is performed it may not be necessary to undergo TEE. Nevertheless, accurate measurements of the aortic valve annulus can also be made by 3D-TEE. Moreover, CT may not be feasible in patients who have renal insufficiency and TEE is a reliable alternative in such patients. Pre-interventional evaluation and echocardiographic monitoring of aortic valve intervention are not part of this focused update and are covered in separate documents.
Basic Assessment of Severity
Recommendations for data recording and measurements are summarized in Table 1 . Measures of AS severity obtained by Doppler echocardiography are summarized in Table 2 .
Data element | Recording | Measurement |
---|---|---|
LVOT diameter |
|
|
LVOT velocity |
|
|
AS jet velocity |
|
|
Valve anatomy |
|
|
∗ See text for the limitations of the assumption of a circular LVOT shape.
Units | Formula/method | Cut-off for severe | Concept | Advantages | Limitations | |
---|---|---|---|---|---|---|
AS jet velocity | m/s | Direct measurement | 4.0 | Velocity increases as stenosis seventy increases | Direct measurement of velocity. Strongest predictor of clinical outcome |
|
Mean gradient | mmHg | Δ P = ∑ 4 v 2 / N | 40 | Pressure gradient calculated from velocity using the Bernouli equation |
|
|
Continuity equation valve area | cm 2 | AVA = ( CSA LVOT × VTI LVOT ) / VTI AV | 1.0 | Volume flow proximal to and in the stenotic orifice is equal |
| Requires LVOT diameter and flow velocity data, along with aortic velocity. Measurement error more likely |
Simplified continuity equation | cm 2 | AVA = ( CSA LVOT × V LVOT ) / V AV | 1.0 | The ratio of LVOT to aortic velocity is similar to the ratio of VTIs with native aortic valve stenosis | Uses more easily measured velocities instead of VTIs | Less accurate if shape of velocity curves is atypical |
Velocity ratio | None | VR = V LVOT V AV | 0.25 | Effective AVA expressed as a proportion of the LVOT area | Doppler-only method. No need to measure LVOT size, less variability than continuity equation | Limited longitudinal data. Ignores LVOT size variability beyond patient size dependence |
Planimetry of anatomic valve area | cm 2 | TTE, TEE, 3D-echo | 1.0 | Anatomic (geometric) CSA of the aortic valve orifice as measured by 2D or 3D echo | Useful if Doppler measurements are unavailable | Contraction coefficient (anatomic/effective valve area) may be variable. Difficult with severe valve calcification |
LV % stroke work loss | % | % SWL = Δ P ¯ Δ P ¯ + SBP · 100 | 25 | Work of the LV wasted each systole for flow to cross the aortic valve, expressed as a % of total systolic work | Very easy to measure. Related to outcome in one longitudinal study | Flow-dependent, Limited longitudinal data |
Recovered pressure gradient | mmHg | P distal − P vc = 4 · v 2 · 2 · AVA AoA · ( 1 − AVA AoA ) | – | Pressure difference between the LV and the aorta, slightly distal to the vena contracta, where distal pressure has increased | Closer to the global haemodynamic burden caused by AS in terms of adaptation of the cardiovascular system. Relevant at high flow states and in patients with small ascending aorta | Introduces complexity and variability related to the measurement of the AoA. No prospective studies showing real advantages over established methods |
Energy loss index | cm 2 /m 2 | ELI = ( AVA · AoA ) / AoA − AVA BSA | 0.5 | Equivalent to the concept of AVA but correcting for distal recovered pressure in the ascending aorta | (As above) Most exact measurement of AS in terms of flow-dynamics. Increased prognostic value in one longitudinal study | Introduces complexity and variability related to the measurement of the AoA |
Valvulo-arterial impedance | mmHg/mL/m 2 | Z VA = Δ P ¯ act + SBP SVI | 5 | Global systole load imposed lo the LV, where the numerator represents an accurate estimation of total LV pressure | Integrates information on arterial bed to the haemodynamic burden of AS, and systemic hypertension is a frequent finding in calcific-degenerative disease | Although named ‘impedance’, only the steady-flow component (i.e. mean resistance) is considered No longitudinal prospective study available |
Aortic valve resistance | Dynes/s/cm 2 | AVR = Δ P ¯ Q ¯ = 4 · v 2 ¯ − r LVOR 2 · v L V ¯ O R · 1333 | 280 | Resistance to flow caused by AS, assuming the hydrodynamics of a tubular (non flat) stenosis | Initially suggested to be less flow-dependent in low-flow AS, but subsequently shown to not be true | Flow dependence. Limned prognostic value. Unrealistic mathematic modelling of flow-dynamics of AS |
Projected valve area at normal flow rate | cm 2 | AVA proj = AVA rest + VC · ( 250 − Q rest ) | 1.0 | Estimation of AVA at normal flow rate by plotting AVA vs. flow and calculating the slope of regression (DSE) | Accounts for the variable changes in flow during DSE in low flow, low gradient AS, provides improved interpretation of AVA changes | Clinical impact still to be shown. Outcome of low-flow AS appears closer related to the presence/absence of LV contractility reserve |
Recommendations for Standard Clinical Practice
(Level 1 Recommendation = appropriate in all patients with AS).
The primary haemodynamic parameters recommended for clinical evaluation of AS severity are:
- •
AS peak jet velocity.
- •
Mean transvalvular pressure gradient.
- •
Aortic valve area by continuity equation.
Peak Jet Velocity
The antegrade systolic velocity across the narrowed aortic valve, or aortic jet velocity, is measured using continuous-wave (CW) Doppler (CWD) ultrasound. Accurate data recording mandates the use of multiple acoustic windows in order to determine the highest velocity (apical and right parasternal or suprasternal view most frequently yield the highest velocity; rarely subcostal or supraclavicular windows yield the highest velocities). Careful patient positioning and adjustment of transducer position and angle are crucial as velocity measurement assumes a parallel intercept angle between the ultrasound beam and direction of blood flow, whereas the direction of the aortic jet in three dimensions is unpredictable and usually cannot be visualized. AS jet velocity is defined as the highest velocity signal obtained from any window after a careful examination; lower values from other views are not reported. The acoustic window that provides the highest aortic jet velocity is noted in the report and usually remains constant on sequential studies in an individual patient, prior to intervention. Occasionally, colour Doppler is helpful to avoid recording the CWD signal of an eccentric mitral regurgitation (MR) jet, but is usually not helpful for AS jet direction. ‘Angle correction’ should not be used because it is likely to introduce more error, given the unpredictable jet direction.
A dedicated small dual-crystal CWD transducer (pencil or PEDOF-pulse echo Doppler flow velocity meter probe) is strongly recommended both because of its higher signal-to-noise ratio and because it allows optimal transducer positioning and angulation, particularly when suprasternal and right parasternal windows are used. However, when flow velocity is low (<3 m/s) and cusp opening is well seen, a combined imaging-Doppler transducer may be adequate.
The spectral Doppler signal is recorded with the spectrogram baseline and the velocity scale adjusted so the signal fills, but fits, on the vertical axis, and with a time scale on the x -axis of 50–100 mm/s. Wall (high-pass) filters are set at a high level and gain is decreased to optimize identification of the velocity curve from the spectrogram envelope. A grayscale signal intensity look-up table is used because this scale maps signal strength using a decibel scale that allows visual separation of noise and transit time effect from the velocity signal. In addition, all of the validation and inter-observer variability studies have been performed using this mode. Colour scales for CW Doppler have different approaches to matching signal strength to colour hue or intensity and are not recommended unless a decibel scale can be verified. In case of poor acoustic quality, the use of echo contrast media has been suggested but is not used in many echocardiography laboratories. In case of its use, proper machine settings (e.g. adequate adjustment gain lowering) are crucial to avoid artefacts and overestimation of velocities.
A smooth velocity curve with a dense outer edge and clear maximum velocity should be recorded. The maximum velocity is measured at the outer edge of the dark signal; fine linear signals at the peak of the curve are owing to transit-time effects and should not be included in measurements. The outer edge of the spectral Doppler envelope is traced ( Figure 2 ) to provide both the velocity–time integral (VTI) for the continuity equation and the mean gradient (see below).
Three or more beats should be averaged for patients in sinus rhythm.
Averaging of more beats is mandatory with irregular rhythms (at least 5 consecutive beats). Special care must be taken to select representative sequences of beats and to avoid post-extrasystolic beats.
The shape of the CWD velocity curve is helpful in distinguishing the level and severity of obstruction. Although the time course of the velocity curve is similar for fixed obstruction at any level (valvular, subvalvular, or supravalvular), the maximum velocity occurs later in systole and the curve is more rounded in shape with more severe obstruction. With mild obstruction, the peak is in early systole with a triangular shape of the velocity curve, compared with the rounded curve with the peak moving towards mid systole in severe stenosis, reflecting a high gradient throughout systole. The shape of the CWD velocity curve also can be helpful in determining whether the obstruction is fixed or dynamic. Dynamic subaortic obstruction shows a characteristic late-peaking velocity curve, which is usually concave upward in early systole ( Figure 3 ).
Mean Pressure Gradient
The pressure difference between the left ventricle (LV) and aorta in systole, or transvalvular aortic gradient, is another standard measure of stenosis severity. Gradients are calculated from velocity information, and therefore the peak gradient obtained from the peak velocity does not add additional information when compared with peak velocity. However, the calculation of the mean gradient, the average gradient across the valve occurring during the entire systole, has potential advantages and should be reported. Although there is overall good correlation between peak gradient and mean gradient, this relationship depends on the shape of the velocity curve, which varies with stenosis severity and flow rate. Transaortic pressure gradient (Δ P ) is calculated from velocity ( v ) using the simplified Bernoulli equation as:
The maximum gradient is calculated from maximum velocity:
The mean gradient is calculated by averaging the instantaneous gradients over the ejection period, a function included in currently available clinical instrument measurement packages using the traced velocity curve. Importantly, the mean gradient requires averaging of instantaneous mean gradients and cannot be calculated from the mean velocity.
This clinical (simplified) equation has been derived from the more complex Bernoulli equation by assuming that viscous losses and acceleration effects are negligible and by using an approximation for the constant that relates to the mass density of blood, a conversion factor for measurement units. In addition, the simplified Bernoulli equation assumes that the proximal velocity can be ignored, a reasonable assumption when this velocity is < 1 m/s because squaring a number <1 makes it even smaller. When the proximal velocity is over 1.5 m/s or the transvalvular velocity is < 3.0 m/s, the proximal velocity should be included in the Bernoulli equation when calculating maximum gradients so that
It is more problematic to include proximal velocity in mean gradient calculations as each point on the ejection curve for the proximal and jet velocities would need to be matched and this approach is not used clinically. In this situation, maximum velocity and gradient should be used to grade stenosis severity.
Sources of error for pressure gradient calculations
Misalignment of the ultrasound beam with the AS jet results in significant underestimation of jet velocity and an even greater underestimation of pressure gradient, owing to the squared relationship between velocity and pressure difference. This highlights the importance of using multiple acoustic windows (as detailed above) for the CWD assessment of AS. Inadvertent recording of MR jet and neglect of an elevated proximal velocity are other limitations of transaortic pressure gradient calculations. It is important to distinguish the AS jet from MR (see Mitral regurgitation section).
There are two additional concerns, related to comparing pressure gradients calculated from Doppler velocities to pressures measured at cardiac catheterization. First, the peak gradient calculated from the maximum Doppler velocity represents the maximum instantaneous pressure difference across the valve, not the difference between the peak left ventricular (LV) and peak aortic pressure that is commonly measured from the pressure tracings. The peak LV and peak aortic pressure do not occur at the same point in time; so, this difference does not represent a physiological measurement and is less than the maximum instantaneous pressure difference.
The second concern is the phenomenon of pressure recovery (PR). The conversion of potential energy to kinetic energy across a narrowed valve results in a high velocity and a drop in pressure. However, distal to the orifice, flow decelerates again. Although some of the kinetic energy dissipates into heat owing to turbulence and viscous losses, some of the kinetic energy will be reconverted into potential energy, which causes an increase in measured static pressure referred to as PR. PR is greatest in stenoses with gradual distal widening, because occurrence of turbulence is then reduced. In general, aortic stenosis, with its abrupt widening from the small orifice to the larger aorta has an unfavourable geometry for PR. However, PR (in mmHg) can be important for some patients with AS. It can be calculated from the Doppler gradient that corresponds to the initial pressure drop across the valve (i.e. 4 v 2 ), the effective orifice area (EOA) as given by the continuity equation and the cross-sectional area of the ascending aorta (AoA), by the following equation: PR = 4 v 2 × 2EOA/AoA × (1 − EOA/AoA). Thus, PR is basically related to the ratio of EOA/AoA. As a relatively small EOA is required to create a relevant gradient, AoA must also be relatively small to end up with a ratio favouring PR. For clinical purposes, aortic size, therefore, is an important determinant and PR should be taken into account primarily in patients with a diameter of the ascending aorta <30 mm. In most adults with native AS, the magnitude of PR is small and can be ignored as long as the diameter of the ascending aorta (at its maximally imaged point) is > 30 mm. However, when the ascending aorta is < 30 mm, one should be aware that the initial pressure drop from LV to the vena contracta, as reflected by Doppler measurement, may be significantly higher than the actual net pressure drop between the LV and the ascending aorta, which represents the pathophysiologically relevant measurement. Therefore, if the magnitude of PR is significant, the aortic valve gradient may be overestimated by echo as it does not incorporate PR. PR may be clinically relevant particularly in congenital AS. Another clinical scenario where PR can be important is in the presence of bileaflet mechanical valves where it can account for falsely elevated prosthetic valve gradients.
Aortic Valve Area
Doppler velocity and pressure gradients are flow dependent. For a given orifice area, velocity, and gradient increase with an increase in transaortic flow rate, and decrease with a decrease in flow rate. Calculation of the stenotic orifice area or effective aortic valve area (AVA) is particularly important when flow rates are very low or very high, although even the degree of valve opening varies to some degree with flow rate (see below).
AVA is calculated by using the continuity-equation ( Figure 4 ) which is based on the concept that the stroke volume (SV) ejected through the LV outflow tract (LVOT) all passes through the stenotic orifice (AVA) and thus SV at valve orifice level is equal to the LVOT SV:
Because volume flow through any crossectional area (CSA) is equal to the CSA times flow velocity over the ejection period (the VTI of the systolic velocity curve), this equation can be rewritten as:
Solving for AVA yields the continuity equation
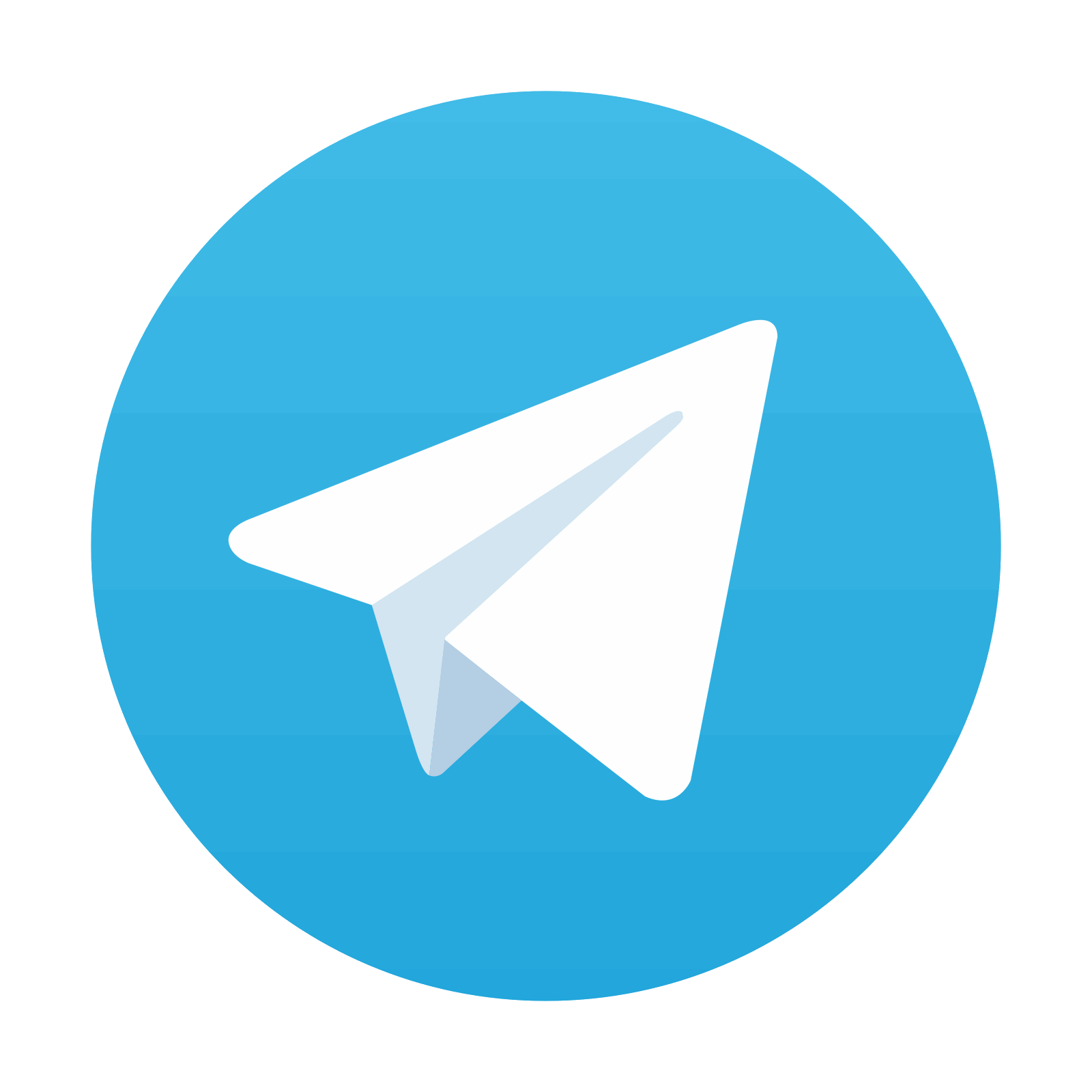
Stay updated, free articles. Join our Telegram channel

Full access? Get Clinical Tree
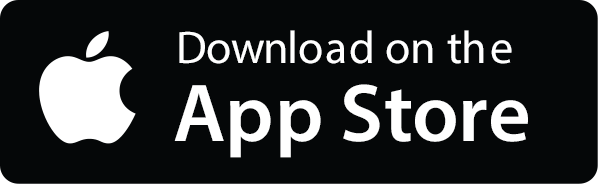
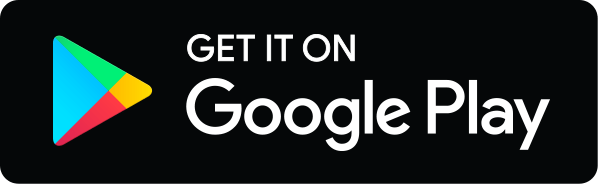