I
Introduction
Attention ASE Members:
ASE has gone green! Visit www.aseuniversity.org to earn free continuing medical education credit through an online activity related to this article. Certificates are available for immediate access upon successful completion of the activity. Nonmembers will need to join the ASE to access this great member benefit!
II
Evaluation of Valvular Regurgitation: General Considerations
A
Identifying the Mechanism of Regurgitation
Valvular regurgitation or insufficiency results from a variety of etiologies that prevent complete apposition of the valve leaflets or cusps. These are grossly divided into organic valve regurgitation (primary regurgitation) with structural alteration of the valvular apparatus and functional regurgitation (secondary regurgitation), whereby cardiac chamber remodeling affects a structurally normal valve, leading to insufficient coaptation. Etiologies of primary valve regurgitation are numerous and include degeneration, inflammation, infection, trauma, tissue disruption, iatrogenic, or congenital. Doppler techniques are very sensitive, and thus trivial or physiologic valve regurgitation, even in a structurally normal valve, can be detected and occurs frequently in right-sided valves.
It is not sufficient to only note the presence of regurgitation. One is obligated to describe the mechanism and possible etiologies, particularly in clinically significant regurgitation, as these affect the severity of regurgitation, cardiac remodeling, and management. The mechanism of regurgitation is not necessarily synonymous with the cause. For example, endocarditis can cause either perforation or valvular prolapse. The resolution (spatial and temporal) of imaging modalities have markedly improved, resulting in identification of the underlying mechanism of regurgitation in the majority of cases. Transthoracic echocardiography (TTE) is usually the first-line imaging modality to investigate valvular regurgitation (etiology, severity, and impact). However, if the TTE is suboptimal, reliance on transesophageal echocardiography (TEE) or CMR would be the next step in evaluating the etiology or severity of regurgitation. Three-dimensional echocardiography has significantly enhanced our understanding of the mechanism of regurgitation and provides a real-time display of the valve in the 3D space. This is particularly evident when imaging the mitral, aortic, and tricuspid valves (TVs) with TEE.
B
Evaluating Valvular Regurgitation with Echocardiography
1
General Principles
TTE with Doppler provides the core of the evaluation of valvular regurgitation severity. Additional methods, echocardiographic (TEE) and nonechocardiographic (computed tomography, CMR, angiography), can be useful at the discretion of examining physicians based on the combination of the potential for these methods to be informative versus their potential risk. This could be particularly important for patients with suboptimal image quality and/or whenever there is a discrepancy between the clinical presentation/symptoms and the evaluation by echocardiography. When TTE provides a complete array of good quality data on the regurgitation, little or no additional information may be needed for the clinical care of patients. However, when the quality of the data is in question, or more precise/accurate measurements are required for clinical decision making, advanced imaging has an important role.
There are a number of principles to apply in the evaluation of valvular regurgitation with echocardiography:
a
Comprehensive imaging
All modalities included in the standard TTE evaluation inclusive of M-mode, 2D, and 3D where applicable, pulsed, color, continuous wave Doppler (CWD), and combined qualitative and quantitative assessment contribute to valve regurgitation assessment.
b
Integrative interpretation
While the predictive power for outcome of all the measurements is not equal and is dominated by a few powerful quantitative measures, interpretation should not rely on a single parameter. Single measures are subject to variability (anatomic, physiologic, and operator); a combination of measures and signs should be comprehensively used to describe and report the final assessment of valve regurgitation.
c
Individualization
Recent data show that valve regurgitation measures and signs that appear similar may have different implications in different etiologies, so that measures and signs require individualized interpretation, taking into account body size, cause of regurgitation, cardiac compliance and function, acuteness or chronicity of the regurgitation, regurgitation dynamics, and hemodynamic conditions at measurement, among others.
d
Precise language
Avoiding imprecision and including detailed and comprehensive observations of the cause, mechanism, severity, location, associated lesions, and cardiac response are required. This language should be standardized and concise. Table 1 summarizes the essential parameters needed in the evaluation of valvular regurgitation with echocardiography.
Parameters | |
---|---|
Clinical information | Symptoms and related clinical findings |
Height/weight/body surface area | |
Blood pressure and heart rate | |
Imaging of the valve | Motion of leaflets: prolapse, flail, restriction, tenting of atrioventricular valves, valve coaptation |
Structure: thickening, calcifications, vegetations | |
Annular size/dilatation | |
Doppler echocardiography of the valve | Site of origin of regurgitation and its direction in the receiving chamber by color Doppler |
The three color Doppler components of the jet: flow convergence, VC, and jet area | |
Density of the jet velocity signal, CW | |
Contour of the jet in MR and TR, CW | |
Deceleration rate or pressure half-time in AR and PR, CW | |
Flow reversal in pulmonary/hepatic veins (MR, TR); in aorta/PA branches (AR, PR) | |
LV and RV filling dynamics (MR, TR) | |
Quantitative parameters for regurgitation | PISA optimization for calculation of RVol and EROA |
Valve annular diameters and corresponding pulsed Doppler for respective SV calculations and derivation of RVol and RF | |
Optimization of LV chamber quantitation (contrast when needed) | |
3D echocardiography ∗ | Localization of valve pathology, particularly with TEE |
LV/RV volumes calculation | |
Measured EROA | |
Automated quantitation of flow and RVol by 3D color flow Doppler † | |
Other echocardiographic data | LV and RV size, function, and hypertrophy |
Left and right atrial size | |
Concomitant valvular disease | |
Estimation of PA pressure |
∗ If available in a laboratory.
2
Echocardiographic Imaging
The main goal of echocardiographic imaging is to define the etiology, mechanism, severity, and impact of the regurgitant lesion on remodeling of the cardiac chambers.
a
Valve structure and severity of regurgitation
Competent leaflets are characterized by a sufficient coaptation surface, which approximates 8-10 mm for the mitral valve (MV), 4-9 mm for the TV, and a few millimeters for semilunar valves. Measurement of leaflet coaptation surface is not accurate with TTE. Three-dimensional TEE or other imaging modalities may allow a prediction of regurgitation severity based on leaflet coaptation. Severe regurgitant lesions when noted represent direct signs of large regurgitant orifices. Such lesions occur in various etiologies: large perforations, large flail segments, profound retraction of leaflets leaving a coaptation gap, or marked tenting of leaflets with tethering and loss of coaptation. All of these findings predict severe valve regurgitation with a high positive predictive value but low sensitivity. Hence, these specific signs are useful when present, but their absence does not exclude severe regurgitation. TTE is the main modality to assess valvular structure usually with the 2D approach, with TEE reserved for inconclusive studies, and to assess eligibility and suitability for transcatheter or surgical procedures. Three-dimensional applications in evaluating valve morphology have had a significant impact on the accuracy of localization of valvular lesions mostly from the transesophageal approach, particularly for the atrioventricular valves. The current lower spatial and temporal resolution of 3D TTE limits its evaluation of valvular structure, however, this is improving.
b
Impact of regurgitation on cardiac remodeling
As blood is incompressible, the regurgitant volume (RVol) must be contained in the cardiac cavities affected, implying that some degree of cavity dilation is proportional to the severity and chronicity of regurgitation. Despite this obligatory remodeling, the dilatation of cardiac cavities is considered in general a supportive sign of valvular regurgitation severity and not a specific sign (unless some conditions are met) because of multiple factors affecting cardiac remodeling. Acute severe regurgitation is characterized by a large regurgitant orifice, but cavity dilatation is minimized. The kinetic energy transmitted through the regurgitant orifice is affected by low cavity compliance, whereby the regurgitant energy is transformed into potential energy (elevated pressure in the receiving chamber) so that rapid equalization of pressure occurs with a low driving force for regurgitation. Consequently, acute severe regurgitation may be brief, with low RVol (low kinetic energy) and little cavity dilatation. In chronic regurgitation, however, cavity dilatation should reflect the regurgitation severity and duration. Cavity dilatation may be specific for significant regurgitation when ventricular function is preserved but loses specificity in conditions such as cardiomyopathy or ischemic ventricular dysfunction. A component of intrinsic dilatation (e.g., cardiomyopathy, atrial dilatation due to atrial fibrillation) may exaggerate the apparent “consequences” of regurgitation. Conversely, in patients with small cavities prior to the onset of regurgitation, an increase in cavity size may be underestimated if preregurgitation cavity size is unknown. Anatomic variability and technical issues may limit the ability to detect cavity dilatation. Measuring cavity diameters rather than volumes has inherent limitations as the diameter-volume relationship is nonlinear. Furthermore, the proposed range of normal values currently available is based on a limited number of subjects, so that for patients with small or very large body size, normalcy is difficult to define. The small body size limitation is of particular concern in evaluating valve regurgitation in females, where normalizing ventricular and regurgitant measurements to body size may provide a more accurate assessment of outcomes. Nevertheless, in a patient with regurgitation, an enlarged ventricle is consistent with significant regurgitation in the chronic setting and in the absence of other modulating factors, particularly when ventricular function is normal. Once a diagnosis of significant regurgitation is established, serial echocardiography with TTE is currently the method of choice to assess the progression of the impact of regurgitation on cardiac chamber structure and function. Careful attention to consistency of measurements and individualized interpretation of results are critical to the assessment of cardiac remodeling as a sign of regurgitation severity. Contrast echocardiography should be used in technically difficult studies for better endocardial visualization, as it enhances overall accuracy of ventricular volume measurements. Three-dimensional TTE can also be used for an overall more accurate assessment of volumes and ejection fraction, as it avoids foreshortening of the left ventricle (LV).
Echocardiography in general tends to underestimate measurements of LV volumes compared to other techniques when the traced endocardium includes ventricular trabeculations; the use of contrast to better visualize the endocardial borders excludes trabeculations and provides larger measurements of cavity size, closer to those by computed tomography and CMR.
3
Color Doppler Imaging
Color flow Doppler is widely used for the detection of regurgitant valve lesions and is the primary method for assessment of regurgitation severity. This technique provides visualization of the origin of the regurgitant jet and its size (VC), the spatial orientation of the regurgitant jet area in the receiving chamber, and, in cases of significant regurgitation, flow convergence into the regurgitant orifice ( Figure 1 ). Experience has shown that attention to these three components of the regurgitation lesion by color Doppler—as opposed to the traditional regurgitant jet area alone with its inherent limitations—significantly improves the overall accuracy of assessment of regurgitation severity. The following are important considerations for color Doppler imaging of regurgitant jets:

a
Jet characteristics and jet area
Since color Doppler visualization of regurgitant jets plays such a significant role in the assessment of valvular regurgitation, it is useful to discuss the underlying basis of color jet formation and display and factors that affect it. A more detailed exposition on color jet formation has been described elsewhere. First, it is important to understand that simply knowing the orifice flow rate is not enough to predict jet size, since the jet will entrain additional flow as it propagates into the receiving chamber and this entrainment strongly depends on the orifice velocity (which in turn is affected by the orifice driving pressure). Rather, jet flow is governed mainly by conservation of momentum. Cardiologists are likely less familiar with momentum as opposed to the other two conserved quantities in fluid flow: mass (manifest in the continuity equation) and energy (found in the Bernoulli equation); but momentum is a critical concept for understanding regurgitant jets. For a jet originating through a regurgitant orifice with effective orifice area A and velocity v , the flow Q is equal to Av , and the momentum M is given by Qv or Av. 2 (By extension, energy is given by Qv 2 or Av 3 ). The amount of momentum that is within a jet at its orifice remains constant throughout the jet. Thus, a 5 m/sec mitral regurgitation (MR) jet with a flow rate of 100 mL/sec should appear the same by color Doppler as a 2.5 m/sec tricuspid regurgitation (TR) jet with a flow rate of 200 mL/sec. For a free turbulent jet, the centerline velocity in the jet drops off inversely with distance from the regurgitant orifice.
To understand how large a jet will appear in color Doppler, one needs to know the minimum velocity that can be detected by the instrument. This is not specifically defined on the echocardiogram but typically is a fraction (around 10%) of the full Nyquist velocity. The jet will appear anywhere the jet velocity is greater than this minimally detectible velocity. The situation is somewhat more complicated in that no jets inside the heart are completely free but are constrained by the chamber walls, causing the velocity to fall off earlier than it would otherwise. The effect of the interplay among momentum, chamber constraint, and minimal displayed velocity on jet area is complex, but for clinical purposes, it suffices to know the following determinants of jet size ( Table 2 ):
- •
Jet momentum ( Av 2 ): a major overall determinant of jet size.
- •
Jet constraint/wall impingement: eccentric wall-hugging jets lose momentum rapidly, thus appearing smaller than nonconstrained jets of the same RVol.
- •
Nyquist limit (velocity scale): reducing the velocity scale emphasizes lower velocities and makes the jet appear larger. In addition, blood cells within the receiving chamber that move in response to or are entrained by the regurgitant jet may reach the minimal velocity and thus appear part of the regurgitant jet.
- •
Orifice geometry: slit-like orifices (particularly imaged along the long axis of the orifice) and multiple separate orifices lead to larger jets than single, relatively round orifices.
- •
Pulse repetition frequency (PRF): affects jet area inversely
- •
Doppler gain: jet size is quite sensitive and proportional to gain.
- •
Ultrasound attenuation: attenuation in the far field, from body habitus, or from an interposing highly reflectant structure such as calcium or metal (interferes with both imaging and Doppler) will decrease jet size.
- •
Transducer frequency: this has a dual effect. The higher frequency experiences a significant Doppler shift at lower velocities, making jets larger, such as in TEE. On the other hand, these higher frequency beams suffer excessive attenuation and jets may appear smaller in the far field, during TTE.
- •
Angle of interrogation: since color Doppler is sensitive only to the component of flow in the direction of the transducer, jets interrogated orthogonally may appear smaller than the same jet imaged axially. This effect actually is lessened as the turbulence within jets leads to high-velocity flow in all directions, thus making the jet visible even when imaged from the side.
- •
Color versus tissue threshold: if the tissue priority is set too high, structures may encroach on the color Doppler signal.
Increases jet area | Reduces jet area |
---|---|
Higher momentum Larger regurgitant orifice area Higher velocity (greater pressure gradient) Higher entrainment of flow | Lower momentum Smaller regurgitant orifice area Lower velocity (lower pressure gradient) Chamber constraint/wall-impinging jet |
Lower Nyquist limit | Higher Nyquist limit |
Higher Doppler gain | Lower Doppler gain |
Far-field beam widening | Far-field attenuation/attenuation by an interposed ultrasound-reflecting structure |
Slit-like regurgitant orifice, imaged along the thin, long shape of the orifice | |
Multiple orifices |
Thus, a larger area of a jet that is central in the cavity may imply more regurgitation, but as discussed, sole reliance on this parameter can be misleading. Figure 2 illustrates examples of modifiers of jet size. Standard technique is to use a Nyquist limit (aliasing velocity [Va]) of 50-70 cm/sec and a high color gain that just eliminates random color speckle from nonmoving regions ( Figure 2 ). Eccentric wall-impinging jets appear significantly smaller than centrally directed jets of similar hemodynamic severity. Their presence however, should also alert to the possibility of structural valve abnormalities (e.g., prolapse, flail, or perforation), frequently situated in the leaflet or cusp opposite to the direction of the jet. A jet may appear larger by increasing the driving pressure across the valve (higher momentum); hence the importance of measuring blood pressure for left heart lesions at the time of the study, particularly in the intraoperative setting or in a sedated patient. Lastly, it is important to note that in cases of very large regurgitant orifice areas, such as in cases of massive TR with a wide, noncoapting valve, a distinct jet may not be seen with color Doppler because of laminar flow and very low blood velocity.

b
Vena contracta
The vena contracta (VC) is the narrowest portion of the regurgitant flow that occurs at or immediately downstream of the regurgitant orifice ( Figure 1 ). It is characterized by high-velocity laminar flow and is slightly smaller than the anatomic regurgitant orifice (ARO). Thus, the cross-sectional area (CSA) of the VC represents a measure of the effective regurgitant orifice area (EROA), a true parameter of lesion severity. The size of the hydraulic VC is independent of flow rate and driving pressure for a fixed orifice. However, if the regurgitant orifice is dynamic, the VC may change during the cardiac cycle. In general, the VC by color Doppler significantly overestimates the hydraulic VC and is dependent on flow rate, likely because of entrainment. Despite these limitations, it remains a helpful semiquantitative measure of valve regurgitation severity. The VC by color Doppler is considerably less dependent on technical factors (e.g., PRF) compared with the jet extent. Imaging of the VC can be achieved using 2D or 3D color-flow Doppler, each presenting different challenges. For 2D VC measurement, it is indispensable to have a linear view of the three components of regurgitant flow (flow convergence, VC, jet area) and to orient the ultrasonic beam as perpendicular to the flow as possible to take advantage of axial measurement accuracy. Hence, it is often necessary to angulate the transducer out of the conventional echocardiographic imaging planes. Proper beam-flow orientation is best achieved for aortic or pulmonary regurgitation (PR), less for MR, and even less for TR. A zoomed view is also indispensable to minimize the measurement inaccuracies for a width of a few millimeters. VCA tracing requires 3D imaging and is achieved offline by reorienting images and using cropping planes to locate the VC. The color flow sector should also be as narrow as possible, to maximize lateral and temporal resolution. Achieving reasonable certainty that the smallest flow area is traced is a tedious process and may be difficult and lengthy; automated processes are being developed to this end. Because of the small values of the width of the VC (usually <1 cm), small errors in its measurement may lead to a large percent error and misclassification of the severity of regurgitation, hence the importance of accurate acquisition of the primary data and measurements.
c
Flow convergence
Locating the flow convergence proximal to the regurgitant orifice provides qualitative information on both the location of the lesion causing the regurgitation and the magnitude of the regurgitant flow. A well-defined small flow convergence combined with a small jet is specific for mild regurgitation, while a large flow convergence recorded at the minimum 50-70 cm/sec range, persisting throughout the duration of flow is specific for severe regurgitation. Flow convergence is amenable to quantitation of regurgitant flow (see below).
4
Pulsed Doppler
Pulsed Doppler is used combined with multiple measures of flow velocity as part of the quantitative assessment of valve regurgitation (see below). However, alterations in forward flow and reversed flow detected with pulsed Doppler associated with regurgitation can also be used as qualitative measures of valve regurgitation severity.
a
Forward flow
Valve regurgitation implies that the forward stroke volume (SV) across the affected valve during the cardiac cycle is increased. For atrioventricular valves, increased forward flow is characterized by increased early E velocity and E/A ratio, generally associated with a short E deceleration time in the absence of stenosis. This supportive sign is mired by the multiple factors affecting ventricular filling including diastolic function, ventricular inflow obstruction (e.g., annular calcification), or alterations in cardiac output. For semilunar valves, ejection velocity is slightly increased with severe regurgitation, but the velocity time integral (VTI) of forward flow at the annulus is generally increased along with a prolongation in ejection time. This supportive sign is nonspecific but is a useful part of the constellation of findings in severe regurgitation.
b
Flow reversal
The RVol, when significant, may cause flow reversal in the receiving or proximal chamber, depending on the valve. In atrioventricular valves, valve regurgitation may result in pulmonary systolic venous flow reversal with MR or hepatic venous systolic flow reversal in cases of TR. Such systolic reversals are considered specific (>85% probability of severe regurgitation when present) but insensitive. Care should be taken to exclude other causes of flow reversal such as atrioventricular dissociation or pacemakers with ventriculoatrial conduction. For aortic regurgitation (AR), reversal of flow is diastolic, noted in the aortic arch and abdominal aorta, and is influenced by multiple factors, particularly peripheral vascular resistance and aortic compliance. Hence, prominent holodiastolic aortic flow reversal is a specific sign of severe AR but insensitive. Other causes of diastolic flow reversal should be sought in the absence of AR such as arteriovenous fistulas, ruptured sinus of Valsalva, or patent ductus arteriosus.
5
Continuous Wave Doppler
Recording of jet velocity with continuous wave Doppler (CWD) provides valuable information as to the velocity and gradient between the two cardiac chambers involved in the regurgitation, its time course, and timing of the regurgitation. The density of the signal is also helpful, provided the Doppler waveform is not overgained.
a
Spectral density
The intensity (amplitude) of the returned Doppler signal is proportional to the number of red blood cells reflecting the signal. Hence, the signal density of the CWD of the regurgitant jet should reflect the regurgitant flow. Thus a faint, incomplete, or soft signal is indicative of trace or mild regurgitation. A dense signal may not be able to differentiate moderate from severe regurgitation. Signal density also depends on spectral recording of the jet throughout the relevant portion of the cardiac cycle. Therefore, a central jet well aligned with the ultrasound beam may appear denser than an eccentric jet of much higher severity, if not well aligned.
b
Timing of regurgitation
The duration and timing of regurgitation can be valuable in the overall assessment of the physiology and hemodynamics of regurgitation. While the majority of regurgitant lesions are holosystolic or holodiastolic, some may occur during a brief period ( Figure 3 ). In patients with MV prolapse (MVP), the regurgitation may be limited to late systole and is rarely severe when not holosystolic, with infrequent cardiac remodeling. MR and TR may be limited to isovolumic contraction and relaxation phases or both, particularly in functional regurgitation, which correspond to mild or trivial regurgitation.

c
Time course of the regurgitant velocity
The spectral velocity profile of a regurgitant jet is determined by the pressure difference between the upstream and downstream chambers, with a general parabolic shape during systole for atrioventricular valves and a trapezoid shape during diastole for semilunar valves. For atrioventricular valves, an early peaking or cutoff sign denotes a large regurgitant wave in the respective atrium and significant regurgitation. A rapid decay of the diastolic slope in semilunar valve regurgitation also can denote significant regurgitation but is exaggerated in cases of poor ventricular compliance and thus may not be specific. Pulmonic regurgitation (PR) may end prior to end diastole and may be related to poor ventricular compliance and/or severe regurgitation. Premature termination of diastolic flow is rarely seen in AR and usually denotes acute severe regurgitation.
6
Quantitative Approaches to Valvular Regurgitation
There are few methods using echo Doppler techniques to quantitate valvular regurgitation. All these methods derive three measures of regurgitation severity:
- •
The EROA, the fundamental measure of lesion severity.
- •
The RVol per beat, which provides a measure of the severity of the volume overload.
- •
The regurgitant fraction (RF) provides a ratio of the RVol to the forward SV specific to the patient.
Prior studies suggest that the absolute measurements of EROA and RVol provide the strongest predictors of outcome. It is uncertain whether normalization of these measures to body size (body surface area or body mass index) is superior to absolute values, particularly in women. Careful attention should be paid to assess whether the regurgitation covers its entire period (systole for atrioventricular, diastole for semilunar valves); for regurgitations limited to part of their flow period, the EROA should be normalized to the entire period of potential regurgitation or ignored, as it would overestimate the severity of regurgitation. Overall, in such partial regurgitations, the RVol is a better measure of regurgitation severity.
There are three methods for quantitative assessment of valvular regurgitation:
a
Quantitative pulsed Doppler method
Doppler recording of VTI can be combined with 2D or 3D measurement of flow area to derive SVs at different sites. The difference between inflow and outflow SVs of the same ventricle is caused by the RVol in single valvular regurgitation. This method is simple in principle, however, accurate results require individual training (e.g., practice in normal patients where SVs at different sites are equal). Briefly, forward SV at any valve annulus—the least variable anatomic area of a valve apparatus—is derived as the product of CSA and the VTI, measured by pulsed Doppler at the annulus. Overall, assumption of a circular geometry has worked well clinically. In this case,
SV = CSA * VTI = ( π d 2 / 4 ) * VTI = 0.785 * d 2 * VTI,
Calculations of SV can be made at two or more different sites: left ventricular outflow tract (LVOT), mitral annulus and right ventricular outflow tract (RVOT). In the absence of regurgitation, SV determinations at these sites are equal. In the presence of regurgitation of one valve, without the presence of any intracardiac shunt, the SV through the affected valve is larger than through the other competent valves. The difference between the two represents the RVol ( Figure 4 ). RF is then derived as the RVol divided by the SV through the regurgitant valve. Thus,
RVol = SV RegValv − SV CompValv ,
RF = RVol/SV RegValv ,

EROA can be calculated using the VTI of the regurgitant jet (VTI RegJet ) recorded by CWD as
EROA = RVol/VTI RegJet ,
All measurements are expressed in centimeters or milliliters, leading to the calculation of EROA in square centimeters.
The most common errors encountered in determining these parameters are (1) failure to measure the valve annulus accurately (error is squared in the formula), (2) failure to trace the modal velocity (brightest signal representing the velocity of the majority of blood cells) of the pulsed Doppler tracing, and (3) failure to position the sample volume correctly, and with minimal angulation, at the level of the annulus. Furthermore, in the case of significant calcifications of the mitral annulus and valve, quantitation of flow at the mitral site is less accurate and more prone to errors.
A major challenge with all volumetric methods is that each of the component SVs has intrinsic error, in part due to the multiple parameters that must be combined into each one. These errors increase (as the root sum square) when the SVs are subtracted, with the relative error increasing even more as one subtracts one large number from another to get a small RVol. For example, a recent study of 3D color flow quantitation demonstrated 95% confidence limits of mitral flow (relative to CMR) of ±18.9 mL and ±17.8 mL for the aortic valve. When these SVs are subtracted, the confidence intervals rise to ±26 mL, emphasizing the critical need for meticulous attention to technique with these methods.
b
Quantitative volumetric method
Because blood is incompressible, the total SV ejected by the ventricle in single-valve regurgitation is equal to the SV at the regurgitant valve (SV RegValv ). If the forward SV (SV Forward ) is measurable simultaneously by Doppler or by any other method, the RVol can be calculated. Most use of this method has been for single left-sided regurgitant valves and the LV SV has been calculated using 2D echocardiography measurement of LV volumes. In such cases SV Forward is measured on the nonregurgitant valve (aortic valve for MR or MV for AR). Hence calculations are the following:
SV LV = ( end-diastolic LV volume ) − ( end-systolic LV volume ) ,
RVol = SV LV − SV Forward ,
EROA = RVol/VTI RegJet .
Methods for calculation of LV volumes by echocardiography have been previously detailed. The limitation of the method is the potential pitfall of underestimating true LV volume as noted above and therefore underestimating regurgitation severity. This can be improved with avoidance of foreshortening and use of contrast echocardiography. Assessment of ventricular volumes based on M-mode measurements has important limitations and is not recommended. The use of 3D echocardiography may improve the accuracy of LV volume determinations.
c
Flow convergence method (proximal isovelocity surface area [PISA] method)
In valvular regurgitation, blood flow converges towards the regurgitant orifice forming concentric, roughly hemispheric shells of increasing velocity and decreasing surface area. Color flow mapping offers the ability to image one of these hemispheres that corresponds to the first aliasing threshold (where the displayed color changes from red to yellow) as one moves out from the regurgitant orifice. This is generally done by shifting the baseline of the color scale in the direction of the regurgitant jet (i.e., down for MR into the LA on TTE and up for MR on TEE) to highlight an aliasing contour where flow convergence has a roughly hemispheric shape; it may appear more teardrop-shaped because lateral flows, perpendicular to the ultrasound beam, cannot be detected by Doppler. Alternatively, one could reduce the PRF to decrease the Nyquist (and aliasing) velocity, although most echocardiographers prefer shifting the baseline. The radius of the PISA is measured from the point of color Doppler aliasing (abrupt change in color from blue to yellow if jet direction is away from transducer) to the VC. Regardless, the aliasing contour is better detected if variance color mapping is turned off. For a hemispheric proximal convergence zone with radius r , the regurgitant flow rate (RFlow, in mL/sec) is calculated as the product of the surface area of the hemisphere (2π r 2 ) and the Va as
RFlow = 2 π r 2 * Va,
Assuming that the selected PISA radius occurs at the time of peak regurgitant velocity, the EROA at that specific time is derived as
EROA = ( 6 . 28 * r 2 * Va ) /Peak V RegJet ,
RVol = EROA * VTI RegJet ,
The PISA method is simple conceptually and in its practical calculation ( Figure 5 ). It allows a qualitative and a quantitative assessment of the severity of the regurgitation and has become the main method of quantification of regurgitation, particularly on the mitral and TVs. However, there are several core principles to pay attention to in order to maintain quality control:
- •
Timing of measurements: since the PISA calculation provides an instantaneous peak flow rate, the EROA calculated by this approach may not be equivalent to the average regurgitant orifice throughout the regurgitant phase. Previous studies using timed measurement in MR have shown that the regurgitation is often dynamic; two important precautions were highlighted : first, measurement of flow and velocity should be performed at simultaneous moments of the regurgitant phase ( Figure 5 ; e.g., not combining a late-systolic flow convergence with a midsystolic velocity). Second, the PISA measurement most representative of the mean EROA is that performed simultaneously with the peak velocity of the regurgitation ( Figure 5 ). Hence it is essential to follow these rules without aiming for the largest flow convergence, while also remembering that the dynamic nature of the jets may lead to underestimation in the case of typically bimodal secondary MR or overestimation in the case of late systolic primary MR.
- •
Duration of regurgitation: the issue of instantaneous versus average measurement comes particularly into play for “partial” regurgitation (e.g., myxomatous MR confined to mid-late systole or functional MR in early systole and isovolumic relaxation). For such partial regurgitation, the RVol can be approximated by multiplying the maximal EROA by the VTI from the densest part of the CWD tracing. When reporting the EROA, however, it is best to use the “mean” EROA, where the maximal EROA is normalized by the proportion of systole during which significant regurgitation occurs; preferably, the RVol should be retained as the most appropriate index. Where feasible, volumetric methods can yield these regurgitant parameters without needing to make assumptions about variation in the EROA.
- •
Shape of PISA: the standard method assumes that the valvular plane from which the regurgitant orifice arises is planar and that the flow convergence is homogeneous, but this is not always the case. First, the plane of the regurgitant orifice may be conical rather than flat or planar (180°), as seen in TR and MV leaflet tenting. This conical angle should be accounted for, as the flow convergence covers more than a hemisphere. Second, the shape of the flow convergence may be inconsistent with a hemisphere, which may require adjustment of the Va such that a well-defined hemisphere is shown. If the flow convergence does not fulfill the criteria of a hemisphere due to constraint, the initial approach is to attempt avoiding the constraint by increasing the Va, making the flow convergence smaller and less prone to constraint. However, if the constraint persists, the echocardiographer should decide whether a simple angle correction for the truncation of the flow convergence is possible, and if not, the method should not be reported.
- •
Shape of the regurgitant orifice: a factor that complicates PISA calculations is the shape of the regurgitant orifice itself. While organic disease (e.g., leaflet flail) usually causes a roughly circular orifice, the regurgitant orifice area in functional disease is often elongated throughout the coaptation line. Application of the standard PISA formula to such an elliptical orifice will lead predictably to flow underestimation. Recent computational fluid dynamics simulations have shown that this underestimation may not be as severe as might be feared, depending on the shape of the ellipse, since the flow contours rapidly become hemispheric as one moves away from the orifice. For a 3:1 ellipse, use of the standard PISA formula (with 40 cm/sec Va and 5 m/sec orifice velocity) only results in 8% underestimation relative to a circular orifice. However, a 5:1 ratio leads to a 17% underestimation, and a 10:1 ratio to a 35% underestimation.

A few additional limitations of PISA should be noted. Central jets allow an easy alignment of the ultrasound beam and the centerline of the flow convergence. In contrast, eccentric jets may present a challenge, for both flow convergence and CWD recording (angulation or inability to record jet despite multiple windows). It is generally easy to identify the aliasing line of the hemispheric contour, but deciding on the position of the regurgitant orifice is more challenging. The presence of dark zones indicating horizontal flow perpendicular to the beam of ultrasound represents the best marker of the position of the regurgitant orifice ( Figure 5 ). In cases where this is not obvious, a display of simultaneous color and noncolor 2D images or turning off the color-flow imaging may be helpful. In cases where the regurgitant orifice is noncircular, as frequently is seen in functional MR (crescent shape), the PISA shape is also modified and no longer hemispheric. Three-dimensional color flow would provide a better assessment of the PISA surface (likely underestimated with 2D PISA), although with additional limitations of lower spatial and temporal resolution. Lastly, in patients with multiple jets, the PISA method can be applied to each orifice, with flows and EROA added together; if one lesion is very mild, it can be neglected. Overall, the PISA method by the combination of both qualitative visual assessment and full calculation is the most used method for quantitation of valvular regurgitation in routine clinical practice.
C
Evaluating Valvular Regurgitation with Cardiac Magnetic Resonance
While echocardiography remains the first line modality for assessment of valvular regurgitation, in some situations, it may be suboptimal. In these instances, CMR can play a useful role because of a number of unique advantages: it provides a view of the entire heart without limitations of imaging windows or body habitus, allows free choice of imaging planes as prescribed by the scan operator, is free of ionizing radiation, and does not require contrast administration. In addition to assessing the severity of the regurgitant lesion, a comprehensive CMR study is able to quantitate cardiac remodeling and provide insight into the mechanism of regurgitation.
1
Cardiac Morphology, Function, and Valvular Anatomy
The typical CMR study for evaluating valvular regurgitation involves the performance of a complete set of short-axis and long-axis (two-, three-, and four-chamber views) cine images using a steady-state free precession (SSFP) pulse sequence, which provides excellent signal-to-noise ratio and high blood-to-myocardium contrast. The typical spatial resolution is 1.5-2.0 mm per pixel with 6- to 8-mm slice thickness and 2- to 4-mm interslice gap to produce a short-axis image every 10 mm from base to apex. Some CMR laboratories have adopted a strategy of utilizing no interslice gap to theoretically improve the accuracy of SV quantification, especially for the RV with its unique geometry. Using this fast pulse sequence, temporal resolution of ≤45 msec (frame rates of 20-25/sec) can be achieved within a 5- to 8-second breath hold that is generally tolerable for most patients. In individuals who have significant difficulty with breath holding, a nonbreath-held “real-time” pulse sequence has been shown to provide comparable assessment of LV and RV volumes and ejection fraction with only a modest compromise in spatial and temporal resolution.
An example of a typical series of cine images is shown in Figure 6 . In addition to providing a comprehensive assessment of regional LV and RV function, this data set can be used to perform planimetry of LV and RV volumes at end diastole and end systole, thus determining ventricular SV and ejection fraction with the method of discs. While full details on performing planimetry of ventricular volumes can be found at the Society for Cardiovascular Magnetic Resonance position statement on standardized image interpretation and post processing, there are a few key points worth mentioning:
a
Ventricular volumes
When performing planimetry, it is important to draw the LV and RV end-diastolic and end-systolic contours on the phases with the largest and smallest blood volume, respectively.
b
Correct placement of the basal ventricular short-axis slice is critical
It is recommended that the most basal slice be located immediately on the myocardial side of the atrioventricular junction at end diastole. As a result of systolic motion of the MV toward the apex, a slice containing LV blood volume at end diastole may include only left atrium (LA) without LV blood volume at end systole. The LA can be identified when less than 50% of the blood volume is surrounded by myocardium and the blood volume cavity is seen to be expanding during systole. It can be helpful to use analysis software that allows adjustment for systolic atrioventricular ring descent using cross-referencing from long-axis locations. The RV contouring is performed in an analogous manner, with the RVOT volume included in the RV end-diastolic volume. Because of the tomographic nature of the technique, CMR can provide these volumetric measures in a 3D fashion without the need for geometric assumptions—in fact, it is considered the gold standard, with demonstrated accuracy and high reproducibility.
c
Planimetry of LV epicardial contour
This can be performed to derive LV mass.
d
Left atrial volume
LA volume can be derived by performance of multiple short-axis slices through the LA or by utilizing the biplane area-length method as in echocardiography.
It is important to note that normal reference ranges for left and right cardiac chamber volumes by CMR are higher than for echocardiography and that correlation between the two modalities is improved with use of 3D echocardiography and/or administration of echocardiographic contrast. The ability to image in any plane makes CMR robust for assessment of valvular anatomy and aids in assessment of mechanism of regurgitation. This can be especially useful for assessment of right-sided valves, which may be difficult to visualize by echocardiography. Assessment of valve anatomy is accomplished by performing a series of contiguous (no gap) parallel thin slice (4-5 mm) SSFP cine acquisitions in the plane of interest. The exact planes to be chosen for each valve will be described in detail in subsequent sections.
2
Assessing Severity of Regurgitation with CMR
Assessing the severity of valvular regurgitation can be performed via a variety of methods: (1) visual assessment of the extent of signal loss due to spin dephasing on cine CMR acquisitions, (2) planimetry of the ARO area from the cine CMR acquisitions of the valve, or (3) quantitation of the RVol. While the first two methods can be used to obtain a qualitative assessment of regurgitant severity, the last method is the most robust and the crux of CMR assessment of valvular regurgitation. The following are approaches and considerations for quantitation of valvular regurgitation ( Table 3 ):
Approach | MR | AR | TR | PR |
---|---|---|---|---|
Preferred method for quantitation ∗ | (LV SV)−(AO total forward SV) | Direct diastolic reverse volume at AO root | (RV SV)−(PA total forward SV) | Direct diastolic reverse volume at PA |
Secondary methods for quantitation † |
|
|
|
|
Corroborating signs of significant regurgitation | LV dilation, LA dilation | LV dilation | RV dilation, right atrium dilation | RV dilation |
∗ Preferred method for quantitation is generally not affected by the presence of concomitant valvular regurgitant lesions.
† Secondary methods are most reliable in the absence of concomitant valvular regurgitation, or as long as the severity of other valvular regurgitant lesions can be accounted for.
a
Phase-contrast CMR
This is the imaging sequence of choice in quantifying flow and calculating velocities. Protons moving along a magnetic field gradient acquire a phase shift relative to stationary spins. The phase shift is directly proportional to the velocity of the moving protons in a linear gradient. Phase-contrast CMR produces two sets of images: magnitude images and phase velocity maps ( Figure 7 ). The magnitude image is used for anatomic orientation of the imaging slice and to identify the boundaries of the vessel imaged. The phase map encodes the velocities within each pixel. Using both images, a region of interest can be traced at each time frame of the data set. The region of interest must be drawn carefully for each frame of the cardiac cycle because of movement and deformation of the vessel. Flow is derived by integrating the velocity of each pixel and its area over the cardiac cycle, allowing for calculation of anterograde and retrograde flows through a region of interest ( Figure 7 ). CMR flow measurements have extensive validation in both in vitro and in vivo studies.
b
Quantitative methods
Methods for quantification of valvular regurgitation can be broadly divided into direct and indirect methods. The direct method employs use of through-plane phase-contrast CMR to quantify blood flow at any given location. This method has been shown to be very accurate for assessing anterograde and retrograde flow across semilunar valves and therefore is the preferred technique used for assessing aortic or pulmonic insufficiency. Phase contrast for direct assessment of flow in the mitral or TVs is more difficult because of significant motion of the annulus during systole. For this reason, quantification of mitral or tricuspid RVol is performed using an alternative, indirect approach by comparing ventricular SV (derived by planimetry of short-axis cines) to forward flow (derived by phase-contrast CMR) across the aortic or pulmonary valves (PVs; Figure 8 ). In addition to the preferred methods for each valve lesion described above, multiple additional indirect methods such as comparison of LV and RV SVs or use of mitral or tricuspid diastolic inflow can be employed to serve as an internal check.
c
Technical considerations
When performing flow measurements a few technical aspects need to be kept in mind. The velocity encoding should be set to the lowest velocity feasible without aliasing. It is important that the imaging plane be (1) centered in the vessel of interest, (2) aligned orthogonally to the expected main blood flow direction in two spatial directions, and (3) centered in the isocenter of the magnetic field. Despite these steps, phase offset errors due to eddy currents in the magnetic field can still occur, and it is important to consider the use of phantom or background correction in every case if possible.
d
Thresholds for regurgitation severity
There is a paucity of data on specific CMR thresholds of RVol or fraction that define severe regurgitation based on outcomes. An earlier study suggested using a RF threshold of 48% by CMR to define severe regurgitation of the aortic or MVs, based on achieving the best correlation with limited echocardiographic assessment. One study in MR and another in AR have focused on RVol/fraction thresholds for prognosis. Progression to symptoms and need for valve surgery were seen with a RF of >40% for MR and >33% for AR (or >55 mL). Due to the paucity of data and absence of other recommendations, the general cutoffs for RVols and RFs recommended by echocardiography and the recent American College of Cardiology/American Heart Association (ACC/AHA) guidelines are used.
3
Strengths and Limitations of CMR
CMR has a number of unique strengths, which make it ideal for assessment of valvular disease ( Table 4 ). Specifically, the free choice of imaging planes allows for a comprehensive assessment of all four cardiac valves without limitations of acoustic windows. In addition, volumetric assessment by CMR has been shown to have high interstudy reproducibility and therefore may be ideal for serial assessment.
Strengths | Limitations |
---|---|
No limitations from acoustic windows or body habitus (except inability to fit in scanner). | Not widely available. Claustrophobia in some patients. Cannot be performed at bedside. |
Free choice of imaging planes. | Unable to perform in patients with certain implanted devices (e.g., pacemakers, defibrillators) except in specialized centers. |
High signal-to-noise ratio and high blood pool to myocardium contrast | Requires good breath holding to achieve optimal image quality. |
Accurate and reproducible measures of cardiac remodeling (i.e., ventricular volumes, function, and mass) without geometric assumptions. | Need to acquire images over several cardiac cycles can lead to compromised quality in setting of arrhythmias (i.e., atrial fibrillation or premature ventricular contractions), and current widely available phase-contrast sequences can fail in frequent arrhythmias. |
Ability to provide information about myocardial viability and scarring if gadolinium contrast is administered. | Difficulty to image small or chaotically mobile objects (e.g., vegetations) due to averaging over multiple cardiac cycles and inadequate spatial and temporal resolution. |
Phase-contrast imaging derives flow using velocities from entire orifice (without needing to assume flat transorifice flow profile or certain geometric shape). | For through-plane phase-contrast imaging, the imaging plane needs to be perpendicular to the blood flow, and difficulty can exist especially in dilated PAs. Adjacent sternal wires and stented valve prosthesis can create susceptibility artifact compromising image quality. |
Allows quantitation of flow through each ventricle (LV/RV) and great vessel (aorta/PA). | Phase-contrast acquisitions have lower temporal resolution than echo Doppler–based methods, which may lead to underestimation of peak velocity. Inadequate spatial resolution may lead to underestimation due to partial volume averaging. |
Severity assessment based on quantitation of RVol or fraction (no hemodynamic or shape assumptions; not affected by jet direction or presence of multiple jets). | In case of turbulence when there is mixed stenosis and regurgitation, phase contrast can underestimate volume due to intravoxel dephasing and loss of signal |
For mitral and TVs there is no established direct method to quantify regurgitation severity | |
Limited data on RVol and fraction cutoffs for severity grading, and limited outcome data available based on the grading. |
The limitations of CMR are listed in Table 4 and include its inability to be performed in patients with certain implanted devices. A comprehensive review of all contraindicated devices is beyond the scope of this document, but it is essential that all CMR laboratories perform careful screening on all patients referred for imaging. Since most CMR acquisitions are performed in a segmented fashion (obtained over multiple cardiac cycles), arrhythmias such as atrial fibrillation or premature ventricular contractions may pose a challenge for standard breath-held phase-contrast CMR sequences. CMR is also not as readily available as echocardiography, cannot be performed at the bedside or in some patients with claustrophobia, and is generally a more expensive modality. There are no uniform thresholds for grading severity of regurgitation, and there is a paucity of outcome data available regarding specific thresholds. Lastly, CMR is unable to assess pressures inside a vessel or cardiac chamber.
4
When Is CMR Indicated?
While echocardiography remains the first-line modality for assessment of valvular regurgitation, CMR is generally indicated when (1) echocardiographic images are suboptimal, (2) when there is discordance between 2D echocardiographic features and Doppler findings (e.g., ventricular enlargement greater than expected on the basis of Doppler measures of valvular regurgitation), or (3) when there is discordance between clinical assessment and severity of valvular regurgitation by echocardiography. Specific scenarios when CMR may be indicated will be described in further detail in subsequent sections for individual valvular lesions.
The direct method described above for quantification of aortic or PR and the indirect method described for mitral and TR are independent of other coexisting valvular lesions, and therefore CMR may be especially useful in the setting of multiple valvular lesions when echocardiographic assessment is challenged. This will be presented in more detail in the section on multivalvular disease.
D
Grading the Severity of Valvular Regurgitation
Characterization of the severity of regurgitant lesions is among the most difficult problems in valvular heart disease. Such a determination is important since mild regurgitation does not lead to remodeling of cardiac chambers and has a benign clinical course, whereas severe regurgitation is associated with significant remodeling, morbidity and mortality. Contributing to the difficulty of assessment of regurgitation is the lack of a true gold standard and the dependence of regurgitation severity on the hemodynamic conditions at the time of evaluation. Although angiography has been used historically to define the degree of regurgitation based on opacification of the receiving chamber, it is also dependent on several technical factors and hemodynamics. For example, an increase in blood pressure will increase all parameters of aortic or MR, be it assessed as RF or angiographic grade. Furthermore, the angiographic severity grades, which have ranged between three and five grades, have only modest correlations with quantitative indices of regurgitation.
Doppler and CMR methods for valvular regurgitation have been validated in animal models against independent flow parameters, and clinically, against the angiographic standard and each other. The majority of these studies have involved left-sided cardiac valves. For Doppler echocardiography, and as discussed above, there are several qualitative and quantitative parameters that can provide assessment of valvular regurgitation. Although this adds to the complexity of evaluation, the availability of these different parameters provides an internal check and corroboration of the severity of the lesion, particularly when technical or physiologic conditions preclude the use of one or the other of these indexes. For CMR, the evaluation involves fewer parameters, is mostly quantitative, but is still influenced by technical and physiologic factors. In order to mitigate the effect of these factors within each individual exam, quantitative internal checks within the CMR exam should be employed. For example, in patients with isolated MR, the difference between the LV SV and aortic total forward flow should be the same as the difference between the LV SV and the pulmonary artery (PA) total forward flow or RV SV. Thus, an integrative, comprehensive approach is essential. In echocardiography, if there are signs suggesting that the regurgitation is significant and the quality of the data lends itself to quantitation, it is desirable for echocardiographers with experience in quantitative methods to determine quantitatively the degree of regurgitation, particularly for left-sided lesions. Ultimately, the interpreter of either echocardiography or CMR must integrate the information and disregard “outlying” data (because of poor quality or a physiologic condition that alters accuracy of a certain parameter), making a best estimate of regurgitation severity. The consensus of the writing group is to classify grading of severity of regurgitation into mild, moderate, and severe. “Trace” regurgitation is used in the event that regurgitation is barely detected. Usually this is physiologic, particularly in right heart valves and MV, and may not produce an audible murmur. Since the severity of regurgitation may be influenced by hemodynamic conditions, it is essential to record the patient’s blood pressure, heart rate, and rhythm at the time of the study and note the patient’s medications whenever possible. When following a patient with serial examinations, these factors need to be considered in comparing the severity of regurgitation and its hemodynamic consequences and actual studies reviewed and compared because of inherent variability of techniques and measurements.
- •
A comprehensive evaluation of valvular regurgitation should include identifying the mechanism and the severity of valvular regurgitation, along with adaptation of the heart to the volume overload.
- •
Height, weight, body surface area, heart rate, rhythm, and blood pressure are required clinical parameters in the assessment of regurgitation.
- •
Echocardiography with Doppler is the primary modality for evaluation of native valvular regurgitation.
- •
Color Doppler is the primary method for detection of regurgitation. In evaluating severity of regurgitation with color Doppler, the three components of the regurgitant jet need to be assessed: flow convergence, VC, and the regurgitant jet direction and area into the receiving chamber.
- •
While color Doppler is important, pulsed and CWD are also essential in providing flow characteristics and dynamics. An integrative interpretation of valvular structure, cardiac size and function, and all Doppler parameters is crucial for assessing regurgitation severity, since each of these parameters has advantages and limitations.
- •
CMR is an excellent modality for evaluating native valvular regurgitation. While echocardiography remains the first-line modality, CMR is indicated when:
- ○
Echo images are suboptimal
- ○
Discordance exists between 2D echocardiographic features and Doppler findings
- ○
Discordance exists between clinical assessment and severity of regurgitation by echocardiography
- ○
- •
In addition to quantifying the severity of regurgitation, a comprehensive CMR study will also quantitate cardiac remodeling (both atrial and ventricular) and provides insights into the mechanism of regurgitation.
- •
Regurgitation severity may be difficult to assess, as it lacks a true gold standard and is influenced by hemodynamic conditions. Quantitative parameters include RVol, RF, and regurgitant orifice area. Recommendations for grading severity of regurgitation are those of mild, moderate, and severe.
II
Evaluation of Valvular Regurgitation: General Considerations
A
Identifying the Mechanism of Regurgitation
Valvular regurgitation or insufficiency results from a variety of etiologies that prevent complete apposition of the valve leaflets or cusps. These are grossly divided into organic valve regurgitation (primary regurgitation) with structural alteration of the valvular apparatus and functional regurgitation (secondary regurgitation), whereby cardiac chamber remodeling affects a structurally normal valve, leading to insufficient coaptation. Etiologies of primary valve regurgitation are numerous and include degeneration, inflammation, infection, trauma, tissue disruption, iatrogenic, or congenital. Doppler techniques are very sensitive, and thus trivial or physiologic valve regurgitation, even in a structurally normal valve, can be detected and occurs frequently in right-sided valves.
It is not sufficient to only note the presence of regurgitation. One is obligated to describe the mechanism and possible etiologies, particularly in clinically significant regurgitation, as these affect the severity of regurgitation, cardiac remodeling, and management. The mechanism of regurgitation is not necessarily synonymous with the cause. For example, endocarditis can cause either perforation or valvular prolapse. The resolution (spatial and temporal) of imaging modalities have markedly improved, resulting in identification of the underlying mechanism of regurgitation in the majority of cases. Transthoracic echocardiography (TTE) is usually the first-line imaging modality to investigate valvular regurgitation (etiology, severity, and impact). However, if the TTE is suboptimal, reliance on transesophageal echocardiography (TEE) or CMR would be the next step in evaluating the etiology or severity of regurgitation. Three-dimensional echocardiography has significantly enhanced our understanding of the mechanism of regurgitation and provides a real-time display of the valve in the 3D space. This is particularly evident when imaging the mitral, aortic, and tricuspid valves (TVs) with TEE.
B
Evaluating Valvular Regurgitation with Echocardiography
1
General Principles
TTE with Doppler provides the core of the evaluation of valvular regurgitation severity. Additional methods, echocardiographic (TEE) and nonechocardiographic (computed tomography, CMR, angiography), can be useful at the discretion of examining physicians based on the combination of the potential for these methods to be informative versus their potential risk. This could be particularly important for patients with suboptimal image quality and/or whenever there is a discrepancy between the clinical presentation/symptoms and the evaluation by echocardiography. When TTE provides a complete array of good quality data on the regurgitation, little or no additional information may be needed for the clinical care of patients. However, when the quality of the data is in question, or more precise/accurate measurements are required for clinical decision making, advanced imaging has an important role.
There are a number of principles to apply in the evaluation of valvular regurgitation with echocardiography:
a
Comprehensive imaging
All modalities included in the standard TTE evaluation inclusive of M-mode, 2D, and 3D where applicable, pulsed, color, continuous wave Doppler (CWD), and combined qualitative and quantitative assessment contribute to valve regurgitation assessment.
b
Integrative interpretation
While the predictive power for outcome of all the measurements is not equal and is dominated by a few powerful quantitative measures, interpretation should not rely on a single parameter. Single measures are subject to variability (anatomic, physiologic, and operator); a combination of measures and signs should be comprehensively used to describe and report the final assessment of valve regurgitation.
c
Individualization
Recent data show that valve regurgitation measures and signs that appear similar may have different implications in different etiologies, so that measures and signs require individualized interpretation, taking into account body size, cause of regurgitation, cardiac compliance and function, acuteness or chronicity of the regurgitation, regurgitation dynamics, and hemodynamic conditions at measurement, among others.
d
Precise language
Avoiding imprecision and including detailed and comprehensive observations of the cause, mechanism, severity, location, associated lesions, and cardiac response are required. This language should be standardized and concise. Table 1 summarizes the essential parameters needed in the evaluation of valvular regurgitation with echocardiography.
Parameters | |
---|---|
Clinical information | Symptoms and related clinical findings |
Height/weight/body surface area | |
Blood pressure and heart rate | |
Imaging of the valve | Motion of leaflets: prolapse, flail, restriction, tenting of atrioventricular valves, valve coaptation |
Structure: thickening, calcifications, vegetations | |
Annular size/dilatation | |
Doppler echocardiography of the valve | Site of origin of regurgitation and its direction in the receiving chamber by color Doppler |
The three color Doppler components of the jet: flow convergence, VC, and jet area | |
Density of the jet velocity signal, CW | |
Contour of the jet in MR and TR, CW | |
Deceleration rate or pressure half-time in AR and PR, CW | |
Flow reversal in pulmonary/hepatic veins (MR, TR); in aorta/PA branches (AR, PR) | |
LV and RV filling dynamics (MR, TR) | |
Quantitative parameters for regurgitation | PISA optimization for calculation of RVol and EROA |
Valve annular diameters and corresponding pulsed Doppler for respective SV calculations and derivation of RVol and RF | |
Optimization of LV chamber quantitation (contrast when needed) | |
3D echocardiography ∗ | Localization of valve pathology, particularly with TEE |
LV/RV volumes calculation | |
Measured EROA | |
Automated quantitation of flow and RVol by 3D color flow Doppler † | |
Other echocardiographic data | LV and RV size, function, and hypertrophy |
Left and right atrial size | |
Concomitant valvular disease | |
Estimation of PA pressure |
∗ If available in a laboratory.
2
Echocardiographic Imaging
The main goal of echocardiographic imaging is to define the etiology, mechanism, severity, and impact of the regurgitant lesion on remodeling of the cardiac chambers.
a
Valve structure and severity of regurgitation
Competent leaflets are characterized by a sufficient coaptation surface, which approximates 8-10 mm for the mitral valve (MV), 4-9 mm for the TV, and a few millimeters for semilunar valves. Measurement of leaflet coaptation surface is not accurate with TTE. Three-dimensional TEE or other imaging modalities may allow a prediction of regurgitation severity based on leaflet coaptation. Severe regurgitant lesions when noted represent direct signs of large regurgitant orifices. Such lesions occur in various etiologies: large perforations, large flail segments, profound retraction of leaflets leaving a coaptation gap, or marked tenting of leaflets with tethering and loss of coaptation. All of these findings predict severe valve regurgitation with a high positive predictive value but low sensitivity. Hence, these specific signs are useful when present, but their absence does not exclude severe regurgitation. TTE is the main modality to assess valvular structure usually with the 2D approach, with TEE reserved for inconclusive studies, and to assess eligibility and suitability for transcatheter or surgical procedures. Three-dimensional applications in evaluating valve morphology have had a significant impact on the accuracy of localization of valvular lesions mostly from the transesophageal approach, particularly for the atrioventricular valves. The current lower spatial and temporal resolution of 3D TTE limits its evaluation of valvular structure, however, this is improving.
b
Impact of regurgitation on cardiac remodeling
As blood is incompressible, the regurgitant volume (RVol) must be contained in the cardiac cavities affected, implying that some degree of cavity dilation is proportional to the severity and chronicity of regurgitation. Despite this obligatory remodeling, the dilatation of cardiac cavities is considered in general a supportive sign of valvular regurgitation severity and not a specific sign (unless some conditions are met) because of multiple factors affecting cardiac remodeling. Acute severe regurgitation is characterized by a large regurgitant orifice, but cavity dilatation is minimized. The kinetic energy transmitted through the regurgitant orifice is affected by low cavity compliance, whereby the regurgitant energy is transformed into potential energy (elevated pressure in the receiving chamber) so that rapid equalization of pressure occurs with a low driving force for regurgitation. Consequently, acute severe regurgitation may be brief, with low RVol (low kinetic energy) and little cavity dilatation. In chronic regurgitation, however, cavity dilatation should reflect the regurgitation severity and duration. Cavity dilatation may be specific for significant regurgitation when ventricular function is preserved but loses specificity in conditions such as cardiomyopathy or ischemic ventricular dysfunction. A component of intrinsic dilatation (e.g., cardiomyopathy, atrial dilatation due to atrial fibrillation) may exaggerate the apparent “consequences” of regurgitation. Conversely, in patients with small cavities prior to the onset of regurgitation, an increase in cavity size may be underestimated if preregurgitation cavity size is unknown. Anatomic variability and technical issues may limit the ability to detect cavity dilatation. Measuring cavity diameters rather than volumes has inherent limitations as the diameter-volume relationship is nonlinear. Furthermore, the proposed range of normal values currently available is based on a limited number of subjects, so that for patients with small or very large body size, normalcy is difficult to define. The small body size limitation is of particular concern in evaluating valve regurgitation in females, where normalizing ventricular and regurgitant measurements to body size may provide a more accurate assessment of outcomes. Nevertheless, in a patient with regurgitation, an enlarged ventricle is consistent with significant regurgitation in the chronic setting and in the absence of other modulating factors, particularly when ventricular function is normal. Once a diagnosis of significant regurgitation is established, serial echocardiography with TTE is currently the method of choice to assess the progression of the impact of regurgitation on cardiac chamber structure and function. Careful attention to consistency of measurements and individualized interpretation of results are critical to the assessment of cardiac remodeling as a sign of regurgitation severity. Contrast echocardiography should be used in technically difficult studies for better endocardial visualization, as it enhances overall accuracy of ventricular volume measurements. Three-dimensional TTE can also be used for an overall more accurate assessment of volumes and ejection fraction, as it avoids foreshortening of the left ventricle (LV).
Echocardiography in general tends to underestimate measurements of LV volumes compared to other techniques when the traced endocardium includes ventricular trabeculations; the use of contrast to better visualize the endocardial borders excludes trabeculations and provides larger measurements of cavity size, closer to those by computed tomography and CMR.
3
Color Doppler Imaging
Color flow Doppler is widely used for the detection of regurgitant valve lesions and is the primary method for assessment of regurgitation severity. This technique provides visualization of the origin of the regurgitant jet and its size (VC), the spatial orientation of the regurgitant jet area in the receiving chamber, and, in cases of significant regurgitation, flow convergence into the regurgitant orifice ( Figure 1 ). Experience has shown that attention to these three components of the regurgitation lesion by color Doppler—as opposed to the traditional regurgitant jet area alone with its inherent limitations—significantly improves the overall accuracy of assessment of regurgitation severity. The following are important considerations for color Doppler imaging of regurgitant jets:
a
Jet characteristics and jet area
Since color Doppler visualization of regurgitant jets plays such a significant role in the assessment of valvular regurgitation, it is useful to discuss the underlying basis of color jet formation and display and factors that affect it. A more detailed exposition on color jet formation has been described elsewhere. First, it is important to understand that simply knowing the orifice flow rate is not enough to predict jet size, since the jet will entrain additional flow as it propagates into the receiving chamber and this entrainment strongly depends on the orifice velocity (which in turn is affected by the orifice driving pressure). Rather, jet flow is governed mainly by conservation of momentum. Cardiologists are likely less familiar with momentum as opposed to the other two conserved quantities in fluid flow: mass (manifest in the continuity equation) and energy (found in the Bernoulli equation); but momentum is a critical concept for understanding regurgitant jets. For a jet originating through a regurgitant orifice with effective orifice area A and velocity v , the flow Q is equal to Av , and the momentum M is given by Qv or Av. 2 (By extension, energy is given by Qv 2 or Av 3 ). The amount of momentum that is within a jet at its orifice remains constant throughout the jet. Thus, a 5 m/sec mitral regurgitation (MR) jet with a flow rate of 100 mL/sec should appear the same by color Doppler as a 2.5 m/sec tricuspid regurgitation (TR) jet with a flow rate of 200 mL/sec. For a free turbulent jet, the centerline velocity in the jet drops off inversely with distance from the regurgitant orifice.
To understand how large a jet will appear in color Doppler, one needs to know the minimum velocity that can be detected by the instrument. This is not specifically defined on the echocardiogram but typically is a fraction (around 10%) of the full Nyquist velocity. The jet will appear anywhere the jet velocity is greater than this minimally detectible velocity. The situation is somewhat more complicated in that no jets inside the heart are completely free but are constrained by the chamber walls, causing the velocity to fall off earlier than it would otherwise. The effect of the interplay among momentum, chamber constraint, and minimal displayed velocity on jet area is complex, but for clinical purposes, it suffices to know the following determinants of jet size ( Table 2 ):
- •
Jet momentum ( Av 2 ): a major overall determinant of jet size.
- •
Jet constraint/wall impingement: eccentric wall-hugging jets lose momentum rapidly, thus appearing smaller than nonconstrained jets of the same RVol.
- •
Nyquist limit (velocity scale): reducing the velocity scale emphasizes lower velocities and makes the jet appear larger. In addition, blood cells within the receiving chamber that move in response to or are entrained by the regurgitant jet may reach the minimal velocity and thus appear part of the regurgitant jet.
- •
Orifice geometry: slit-like orifices (particularly imaged along the long axis of the orifice) and multiple separate orifices lead to larger jets than single, relatively round orifices.
- •
Pulse repetition frequency (PRF): affects jet area inversely
- •
Doppler gain: jet size is quite sensitive and proportional to gain.
- •
Ultrasound attenuation: attenuation in the far field, from body habitus, or from an interposing highly reflectant structure such as calcium or metal (interferes with both imaging and Doppler) will decrease jet size.
- •
Transducer frequency: this has a dual effect. The higher frequency experiences a significant Doppler shift at lower velocities, making jets larger, such as in TEE. On the other hand, these higher frequency beams suffer excessive attenuation and jets may appear smaller in the far field, during TTE.
- •
Angle of interrogation: since color Doppler is sensitive only to the component of flow in the direction of the transducer, jets interrogated orthogonally may appear smaller than the same jet imaged axially. This effect actually is lessened as the turbulence within jets leads to high-velocity flow in all directions, thus making the jet visible even when imaged from the side.
- •
Color versus tissue threshold: if the tissue priority is set too high, structures may encroach on the color Doppler signal.
Increases jet area | Reduces jet area |
---|---|
Higher momentum Larger regurgitant orifice area Higher velocity (greater pressure gradient) Higher entrainment of flow | Lower momentum Smaller regurgitant orifice area Lower velocity (lower pressure gradient) Chamber constraint/wall-impinging jet |
Lower Nyquist limit | Higher Nyquist limit |
Higher Doppler gain | Lower Doppler gain |
Far-field beam widening | Far-field attenuation/attenuation by an interposed ultrasound-reflecting structure |
Slit-like regurgitant orifice, imaged along the thin, long shape of the orifice | |
Multiple orifices |
Thus, a larger area of a jet that is central in the cavity may imply more regurgitation, but as discussed, sole reliance on this parameter can be misleading. Figure 2 illustrates examples of modifiers of jet size. Standard technique is to use a Nyquist limit (aliasing velocity [Va]) of 50-70 cm/sec and a high color gain that just eliminates random color speckle from nonmoving regions ( Figure 2 ). Eccentric wall-impinging jets appear significantly smaller than centrally directed jets of similar hemodynamic severity. Their presence however, should also alert to the possibility of structural valve abnormalities (e.g., prolapse, flail, or perforation), frequently situated in the leaflet or cusp opposite to the direction of the jet. A jet may appear larger by increasing the driving pressure across the valve (higher momentum); hence the importance of measuring blood pressure for left heart lesions at the time of the study, particularly in the intraoperative setting or in a sedated patient. Lastly, it is important to note that in cases of very large regurgitant orifice areas, such as in cases of massive TR with a wide, noncoapting valve, a distinct jet may not be seen with color Doppler because of laminar flow and very low blood velocity.
b
Vena contracta
The vena contracta (VC) is the narrowest portion of the regurgitant flow that occurs at or immediately downstream of the regurgitant orifice ( Figure 1 ). It is characterized by high-velocity laminar flow and is slightly smaller than the anatomic regurgitant orifice (ARO). Thus, the cross-sectional area (CSA) of the VC represents a measure of the effective regurgitant orifice area (EROA), a true parameter of lesion severity. The size of the hydraulic VC is independent of flow rate and driving pressure for a fixed orifice. However, if the regurgitant orifice is dynamic, the VC may change during the cardiac cycle. In general, the VC by color Doppler significantly overestimates the hydraulic VC and is dependent on flow rate, likely because of entrainment. Despite these limitations, it remains a helpful semiquantitative measure of valve regurgitation severity. The VC by color Doppler is considerably less dependent on technical factors (e.g., PRF) compared with the jet extent. Imaging of the VC can be achieved using 2D or 3D color-flow Doppler, each presenting different challenges. For 2D VC measurement, it is indispensable to have a linear view of the three components of regurgitant flow (flow convergence, VC, jet area) and to orient the ultrasonic beam as perpendicular to the flow as possible to take advantage of axial measurement accuracy. Hence, it is often necessary to angulate the transducer out of the conventional echocardiographic imaging planes. Proper beam-flow orientation is best achieved for aortic or pulmonary regurgitation (PR), less for MR, and even less for TR. A zoomed view is also indispensable to minimize the measurement inaccuracies for a width of a few millimeters. VCA tracing requires 3D imaging and is achieved offline by reorienting images and using cropping planes to locate the VC. The color flow sector should also be as narrow as possible, to maximize lateral and temporal resolution. Achieving reasonable certainty that the smallest flow area is traced is a tedious process and may be difficult and lengthy; automated processes are being developed to this end. Because of the small values of the width of the VC (usually <1 cm), small errors in its measurement may lead to a large percent error and misclassification of the severity of regurgitation, hence the importance of accurate acquisition of the primary data and measurements.
c
Flow convergence
Locating the flow convergence proximal to the regurgitant orifice provides qualitative information on both the location of the lesion causing the regurgitation and the magnitude of the regurgitant flow. A well-defined small flow convergence combined with a small jet is specific for mild regurgitation, while a large flow convergence recorded at the minimum 50-70 cm/sec range, persisting throughout the duration of flow is specific for severe regurgitation. Flow convergence is amenable to quantitation of regurgitant flow (see below).
4
Pulsed Doppler
Pulsed Doppler is used combined with multiple measures of flow velocity as part of the quantitative assessment of valve regurgitation (see below). However, alterations in forward flow and reversed flow detected with pulsed Doppler associated with regurgitation can also be used as qualitative measures of valve regurgitation severity.
a
Forward flow
Valve regurgitation implies that the forward stroke volume (SV) across the affected valve during the cardiac cycle is increased. For atrioventricular valves, increased forward flow is characterized by increased early E velocity and E/A ratio, generally associated with a short E deceleration time in the absence of stenosis. This supportive sign is mired by the multiple factors affecting ventricular filling including diastolic function, ventricular inflow obstruction (e.g., annular calcification), or alterations in cardiac output. For semilunar valves, ejection velocity is slightly increased with severe regurgitation, but the velocity time integral (VTI) of forward flow at the annulus is generally increased along with a prolongation in ejection time. This supportive sign is nonspecific but is a useful part of the constellation of findings in severe regurgitation.
b
Flow reversal
The RVol, when significant, may cause flow reversal in the receiving or proximal chamber, depending on the valve. In atrioventricular valves, valve regurgitation may result in pulmonary systolic venous flow reversal with MR or hepatic venous systolic flow reversal in cases of TR. Such systolic reversals are considered specific (>85% probability of severe regurgitation when present) but insensitive. Care should be taken to exclude other causes of flow reversal such as atrioventricular dissociation or pacemakers with ventriculoatrial conduction. For aortic regurgitation (AR), reversal of flow is diastolic, noted in the aortic arch and abdominal aorta, and is influenced by multiple factors, particularly peripheral vascular resistance and aortic compliance. Hence, prominent holodiastolic aortic flow reversal is a specific sign of severe AR but insensitive. Other causes of diastolic flow reversal should be sought in the absence of AR such as arteriovenous fistulas, ruptured sinus of Valsalva, or patent ductus arteriosus.
5
Continuous Wave Doppler
Recording of jet velocity with continuous wave Doppler (CWD) provides valuable information as to the velocity and gradient between the two cardiac chambers involved in the regurgitation, its time course, and timing of the regurgitation. The density of the signal is also helpful, provided the Doppler waveform is not overgained.
a
Spectral density
The intensity (amplitude) of the returned Doppler signal is proportional to the number of red blood cells reflecting the signal. Hence, the signal density of the CWD of the regurgitant jet should reflect the regurgitant flow. Thus a faint, incomplete, or soft signal is indicative of trace or mild regurgitation. A dense signal may not be able to differentiate moderate from severe regurgitation. Signal density also depends on spectral recording of the jet throughout the relevant portion of the cardiac cycle. Therefore, a central jet well aligned with the ultrasound beam may appear denser than an eccentric jet of much higher severity, if not well aligned.
b
Timing of regurgitation
The duration and timing of regurgitation can be valuable in the overall assessment of the physiology and hemodynamics of regurgitation. While the majority of regurgitant lesions are holosystolic or holodiastolic, some may occur during a brief period ( Figure 3 ). In patients with MV prolapse (MVP), the regurgitation may be limited to late systole and is rarely severe when not holosystolic, with infrequent cardiac remodeling. MR and TR may be limited to isovolumic contraction and relaxation phases or both, particularly in functional regurgitation, which correspond to mild or trivial regurgitation.
c
Time course of the regurgitant velocity
The spectral velocity profile of a regurgitant jet is determined by the pressure difference between the upstream and downstream chambers, with a general parabolic shape during systole for atrioventricular valves and a trapezoid shape during diastole for semilunar valves. For atrioventricular valves, an early peaking or cutoff sign denotes a large regurgitant wave in the respective atrium and significant regurgitation. A rapid decay of the diastolic slope in semilunar valve regurgitation also can denote significant regurgitation but is exaggerated in cases of poor ventricular compliance and thus may not be specific. Pulmonic regurgitation (PR) may end prior to end diastole and may be related to poor ventricular compliance and/or severe regurgitation. Premature termination of diastolic flow is rarely seen in AR and usually denotes acute severe regurgitation.
6
Quantitative Approaches to Valvular Regurgitation
There are few methods using echo Doppler techniques to quantitate valvular regurgitation. All these methods derive three measures of regurgitation severity:
- •
The EROA, the fundamental measure of lesion severity.
- •
The RVol per beat, which provides a measure of the severity of the volume overload.
- •
The regurgitant fraction (RF) provides a ratio of the RVol to the forward SV specific to the patient.
Prior studies suggest that the absolute measurements of EROA and RVol provide the strongest predictors of outcome. It is uncertain whether normalization of these measures to body size (body surface area or body mass index) is superior to absolute values, particularly in women. Careful attention should be paid to assess whether the regurgitation covers its entire period (systole for atrioventricular, diastole for semilunar valves); for regurgitations limited to part of their flow period, the EROA should be normalized to the entire period of potential regurgitation or ignored, as it would overestimate the severity of regurgitation. Overall, in such partial regurgitations, the RVol is a better measure of regurgitation severity.
There are three methods for quantitative assessment of valvular regurgitation:
a
Quantitative pulsed Doppler method
Doppler recording of VTI can be combined with 2D or 3D measurement of flow area to derive SVs at different sites. The difference between inflow and outflow SVs of the same ventricle is caused by the RVol in single valvular regurgitation. This method is simple in principle, however, accurate results require individual training (e.g., practice in normal patients where SVs at different sites are equal). Briefly, forward SV at any valve annulus—the least variable anatomic area of a valve apparatus—is derived as the product of CSA and the VTI, measured by pulsed Doppler at the annulus. Overall, assumption of a circular geometry has worked well clinically. In this case,
SV = CSA * VTI = ( π d 2 / 4 ) * VTI = 0.785 * d 2 * VTI,
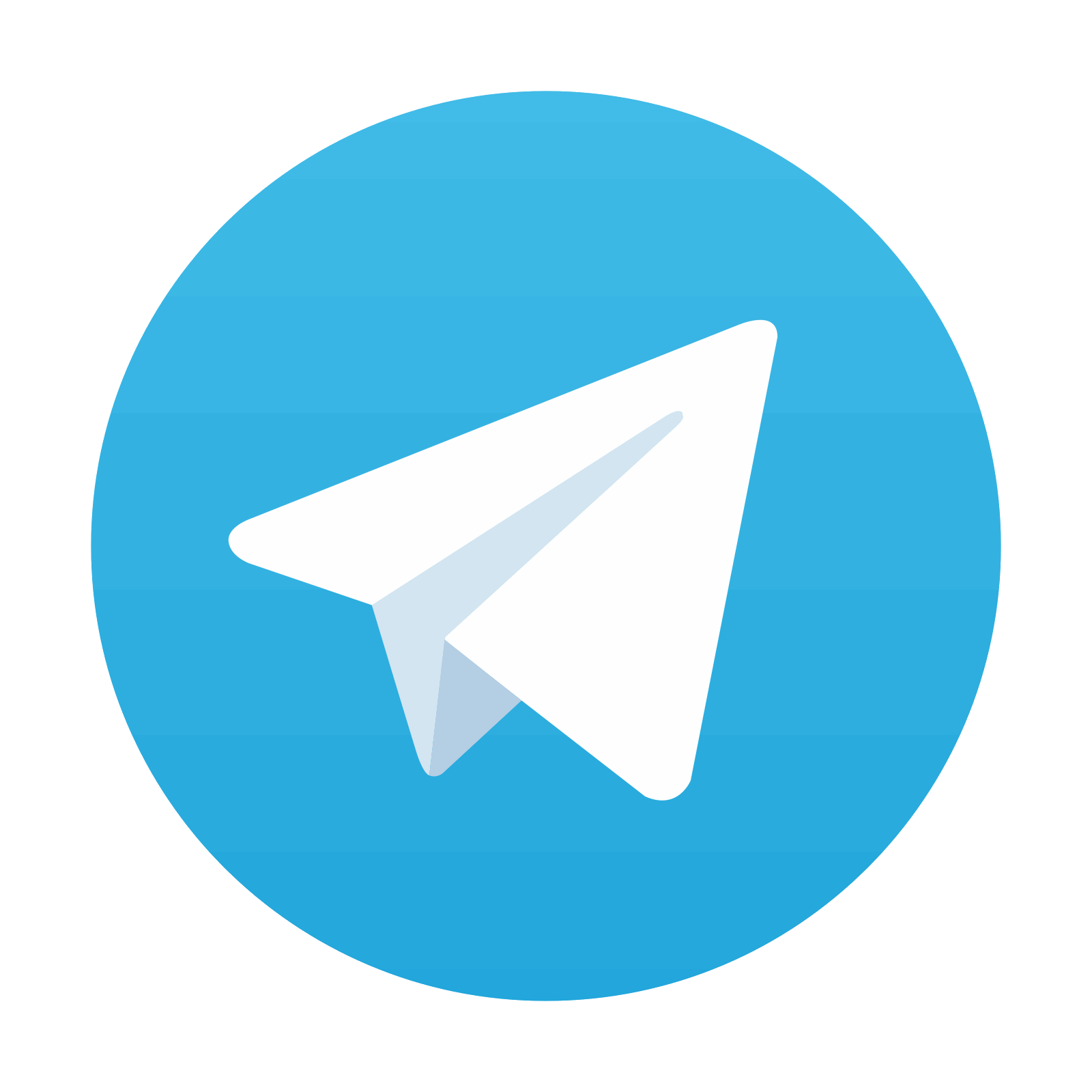
Stay updated, free articles. Join our Telegram channel

Full access? Get Clinical Tree
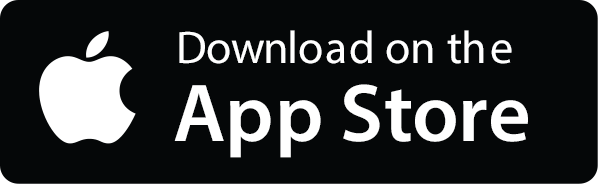
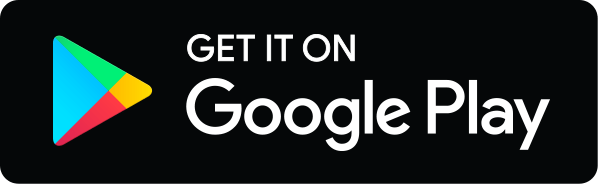