The rapid technological developments of the past decade and the changes in echocardiographic practice brought about by these developments have resulted in the need for updated recommendations to the previously published guidelines for cardiac chamber quantification, which was the goal of the joint writing group assembled by the American Society of Echocardiography and the European Association of Cardiovascular Imaging. This document provides updated normal values for all four cardiac chambers, including three-dimensional echocardiography and myocardial deformation, when possible, on the basis of considerably larger numbers of normal subjects, compiled from multiple databases. In addition, this document attempts to eliminate several minor discrepancies that existed between previously published guidelines.
Attention ASE Members:
The ASE has gone green! Visit www.aseuniversity.org to earn free continuing medical education credit through an online activity related to this article. Certificates are available for immediate access upon successful completion of the activity. Nonmembers will need to join the ASE to access this great member benefit!
I
The Left Ventricle
1
Measurement of LV Size
The most commonly used parameters to describe LV cavity size include linear internal dimensions and volumes. Measurements are commonly reported for end-diastole and end-systole, which are then used to derive parameters of global LV function. To allow comparison among individuals with different body sizes, chamber measurements should be reported indexed to BSA.
1.1
Linear Measurements
It is recommended that linear internal measurements of the left ventricle and its walls be performed in the parasternal long-axis view. Values should be carefully obtained perpendicular to the LV long axis and measured at or immediately below the level of the mitral valve leaflet tips. In this regard, the electronic calipers should be positioned on the interface between the myocardial wall and cavity and the interface between the wall and the pericardium. Internal dimensions can be obtained with a two-dimensional (2D) echocardiography (2DE)–guided M-mode approach, although linear measurements obtained from 2D echocardiographic images are preferred to avoid oblique sections of the ventricle ( Table 1 ).
1.2
Volumetric Measurements
LV volumes are measured using 2DE or 3DE. Volume calculations derived from linear measurements may be inaccurate, because they rely on the assumption of a fixed geometric LV shape such as a prolate ellipsoid, which does not apply in a variety of cardiac pathologies. Accordingly, the Teichholz and Quinones methods for calculating LV volumes from LV linear dimensions are no longer recommended for clinical use.
Volumetric measurements are usually based on tracings of the interface between the compacted myocardium and the LV cavity. At the mitral valve level, the contour is closed by connecting the two opposite sections of the mitral ring with a straight line. LV length is defined as the distance between the bisector of this line and the apical point of the LV contour, which is most distant to it. The use of the longer LV length between the apical two- and four-chamber views is recommended.
LV volumes should be measured from the apical four- and two-chamber views. Two-dimensional echocardiographic image acquisition should aim to maximize LV areas, while avoiding foreshortening of the left ventricle, which results in volume underestimation. Acquiring LV views at a reduced depth to focus on the LV cavity will reduce the likelihood of foreshortening and minimize errors in endocardial border tracings ( Table 1 ). Because the issue of foreshortening is less relevant in 3D data sets, 3D image acquisition should focus primarily on including the entire left ventricle within the pyramidal data set. To ensure reasonably accurate identification of end-systole, the temporal resolution of 3D imaging should be maximized without compromising spatial resolution.
Contrast agents should be used when needed to improve endocardial delineation when two or more contiguous LV endocardial segments are poorly visualized in apical views, as per published guidelines. Contrast-enhanced images may provide larger volumes than unenhanced images that are closer to those obtained with cardiac magnetic resonance (CMR) in head-to-head comparison. Care should be taken to avoid acoustic shadowing, which may occur in LV basal segments in the presence of high concentrations of contrast. Normal reference values for LV volumes with contrast enhancement are not well established.
The most commonly used method for 2D echocardiographic volume calculations is the biplane method of disks summation (modified Simpson’s rule), which is the recommended 2D echocardiographic method by consensus of this committee ( Table 1 ). An alternative method to calculate LV volumes when apical endocardial definition precludes accurate tracing is the area-length method, in which the LV is assumed to be bullet shaped. The mid-LV cross-sectional area is computed by planimetry in the parasternal short-axis view and the length of the ventricle taken from the midpoint of the annular plane to the apex in the apical four-chamber view ( Table 1 ). The shortcoming of this method is that the bullet-shape assumption does not always hold true. One of the advantages of 3D echocardiographic volume measurements is that they do not rely on geometric assumptions. In patients with good image quality, 3D echocardiographic measurements are accurate and reproducible and should therefore be used when available and feasible. The advantages and disadvantages of the various methods are summarized in Table 1 .
1.3
Normal Reference Values for 2DE
Data were extracted from seven databases, including Asklepios (year 0 and year 10), Flemengho, CARDIA5 and CARDIA25, Padua 3D Echo Normal, and the Normal Reference Ranges for Echocardiography study, to obtain reference values in normal subjects for the left ventricle and the left atrium (see section 10). All data were obtained without the use of contrast agents. Data sets for all patients included age, gender, ethnicity, height, and weight. To ensure a normal population, subjects in these studies were excluded if any of the following criteria were met: systolic blood pressure > 140 mm Hg, diastolic blood pressure > 80 mm Hg, history of drug-treated hypertension, diagnosis of diabetes, impaired fasting glucose > 100 mg/dL, body mass index > 30 kg/m 2 , creatinine > 1.3 mg/dL, estimated glomerular filtration rate < 60 mL/min/1.73 m 2 , total cholesterol > 240 mg/dL, low-density lipoprotein cholesterol > 130 mg/dL, and total triglycerides > 150 mg/dL. Details of the statistical analysis are described in the Appendix . Because of varied study aims, not all echocardiographic measurements were available for each database. Supplemental Table 1 summarizes the sources of the data for each measurement group and their baseline characteristics.
Table 2 shows the normal values for 2D echocardiographic parameters of LV size and function according to gender, while Supplemental Table 2 provides expanded data for the same parameters, obtained from different echocardiographic views, and also includes the corresponding number of subjects used to obtain these data. Supplemental Table 3 lists normal ranges and consensus-based partition cutoffs for LV dimensions, volumes, EF, and mass. On multivariate analysis, age, gender, and BSA were found to have a significant independent influence on LV end-diastolic volume (EDV) and LV end-systolic volume (ESV). The results across genders and age deciles subdivided into absolute and BSA-normalized values are shown in Supplemental Table 4 (see Appendix ).
Parameter | Male | Female | ||
---|---|---|---|---|
Mean ± SD | 2-SD range | Mean ± SD | 2-SD range | |
LV internal dimension | ||||
Diastolic dimension (mm) | 50.2 ± 4.1 | 42.0–58.4 | 45.0 ± 3.6 | 37.8–52.2 |
Systolic dimension (mm) | 32.4 ± 3.7 | 25.0–39.8 | 28.2 ± 3.3 | 21.6–34.8 |
LV volumes (biplane) | ||||
LV EDV (mL) | 106 ± 22 | 62–150 | 76 ± 15 | 46–106 |
LV ESV (mL) | 41 ± 10 | 21–61 | 28 ± 7 | 14–42 |
LV volumes normalized by BSA | ||||
LV EDV (mL/m 2 ) | 54 ± 10 | 34–74 | 45 ± 8 | 29–61 |
LV ESV (mL/m 2 ) | 21 ± 5 | 11–31 | 16 ± 4 | 8–24 |
LV EF (biplane) | 62 ± 5 | 52–72 | 64 ± 5 | 54–74 |
Because ethnicity is an important factor, results of analysis by race and gender are presented in Supplemental Table 5 . From the regression analysis, nomograms are provided for plotting observed LV dimensions versus BSA or BSA-indexed LV volumes versus age ( Figures 1 and 2 ). Nomograms for absolute LV measurements against age ( Supplemental Figures 1 and 2 ) and BSA ( Supplemental Figures 3 and 4 ) are also provided (see Appendix ).
1.4
Normal Reference Values for 3DE
Several studies have published 3D echocardiographic reference values for healthy normotensive subjects, which are summarized in Table 3 . The reported variations in the normal ranges from study to study are likely due to differences in populations, echocardiographic equipment, and analysis software, as well as variability in measurement techniques. In patients with good image quality, the accuracy of 3DE is comparable with that of CMR, although volumes tend to be lower on echocardiography.
Aune et al . (2010) | Fukuda et al . (2012) | Chahal et al . (2012) | Muraru et al . (2013) | |
---|---|---|---|---|
Number of subjects | 166 | 410 | 978 | 226 |
Ethnic makeup of population | Scandinavian | Japanese | 51% European white, 49% Asian Indian | White European |
EDVi (mL/m 2 ) | ||||
Men, mean (LLN, ULN) | 66 (46, 86) | 50 (26, 74) | White: 49 (31, 67); Indian: 41 (23, 59) | 63 (41, 85) |
Women, mean (LLN, ULN) | 58 (42, 74) | 46 (28, 64) | White: 42 (26, 58); Indian: 39 (23, 55) | 56 (40, 78) |
ESVi (mL/m 2 ) | ||||
Men, mean (LLN, ULN) | 29 (17, 41) | 19 (9, 29) | White: 19 (9, 29); Indian: 16 (6, 26) | 24 (14, 34) |
Women, mean (LLN, ULN) | 23 (13, 33) | 17 (9, 25) | White: 16 (8, 24); Indian: 15 (7, 23) | 20 (12, 28) |
EF (%) | ||||
Men, mean (LLN, ULN) | 57 (49, 65) | 61 (53, 69) | White: 61 (49, 73); Indian: 62 (52, 72) | 62 (54, 70) |
Women, mean (LLN, ULN) | 61 (49, 73) | 63 (55, 71) | White: 62 (52, 72); Indian: 62 (52, 72) | 65 (57, 73) |
The effects of ethnicity on 3D echocardiographic LV volumes were investigated in one study, which reported that LV volumes were smaller among Asian Indians than white Europeans, but EF did not differ among ethnic groups. In most 3D echocardiographic studies, the relationship between age and 3D echocardiographic LV volumes was examined, and weak to moderate negative correlations were seen between age and LV volumes, while EF did not change significantly with age. This finding is similar to those described in the CMR literature. On the basis of weighted averages of three studies, 3D echocardiographic LV volumes were larger than 2D echocardiographic values, and corresponding upper limits of the normal range were EDVs of 79 mL/m 2 for men and 71 mL/m 2 for women and ESVs of 32 mL/m 2 for men and 28 mL/m 2 for women. Ultimately, a large study in a diverse population will be needed to establish normal reference ranges for 3DE for different ethnic groups.
Recommendation
LV size should be routinely assessed on 2DE by calculating volumes using the biplane method of disks summation technique. In laboratories with experience in 3DE, 3D measurement and reporting of LV volumes is recommended when feasible depending on image quality. When reporting LV linear dimensions, the recommended method is 2D-guided measurements. LV size and volume measurements should be reported indexed to BSA. For general reference, 2D echocardiographic LV EDVs of 74 mL/m 2 for men and 61 mL/m 2 for women and LV ESVs of 31 mL/m 2 for men and 24 mL/m 2 for women should be used as the upper limits of the corresponding normal range.
2
LV Global Systolic Function
Global LV function is usually assessed by measuring the difference between the end-diastolic and end-systolic value of a one-dimensional, 2D, or 3D parameter divided by its end-diastolic value. For this, end-diastole is preferably defined as the first frame after mitral valve closure or the frame in the cardiac cycle in which the respective LV dimension or volume measurement is the largest. End-systole is best defined as the frame after aortic valve closure or the frame in which the cardiac dimension or volume is smallest. In patients with regular heart rhythm, measurements of the timing of valve openings and closures derived from M-mode echocardiography, pulsed-wave (PW) or continuous-wave Doppler may be used for accurate definitions of ventricular time intervals.
2.1
Fractional Shortening
Fractional shortening can be derived from 2D-guided M-mode imaging or preferably from linear measurements obtained from 2D images. Deriving global LV function parameters from linear measurements is problematic when there are regional wall motion abnormalities due to coronary disease or conduction abnormalities. In patients with uncomplicated hypertension, obesity or valvular diseases, such regional differences are rare in the absence of clinically recognized myocardial infarction, and accordingly, this parameter may provide useful information in clinical studies. In patients with normal size of the LV base but enlarged midventricular and distal portions, LV volume would be a better marker of LV size than linear dimension measured at the LV base.
2.2
EF
EF is calculated from EDV and ESV estimates, using the following formula:
LV volume estimates may be derived from 2DE or 3DE, as described above (section 1.2). The biplane method of disks (modified Simpson’s rule) is the currently recommended 2D method to assess LV EF by consensus of this committee. Table 4 lists 2DE-derived biplane LV EF, including normal ranges and consensus-based severity partition cutoffs according to gender. In patients with good image quality, 3DE-based EF measurements are accurate and reproducible and should be used when available and feasible.
Male | Female | |||||||
---|---|---|---|---|---|---|---|---|
Normal range | Mildly abnormal | Moderately abnormal | Severely abnormal | Normal range | Mildly abnormal | Moderately abnormal | Severely abnormal | |
LV EF (%) | 52–72 | 41–51 | 30–40 | <30 | 54–74 | 41–53 | 30–40 | <30 |
Maximum LA volume/BSA (mL/m 2 ) | 16–34 | 35–41 | 42–48 | >48 | 16–34 | 35–41 | 42–48 | >48 |
2.3
Global Longitudinal Strain (GLS)
Lagrangian strain is defined as the change in length of an object within a certain direction relative to its baseline length:
where L t is the length at time t , and L 0 is the initial length at time 0. The most commonly used strain-based measure of LV global systolic function is GLS. It is usually assessed by speckle-tracking echocardiography (STE) ( Table 1 ). On 2DE, peak GLS describes the relative length change of the LV myocardium between end-diastole and end-systole:
where ML is myocardial length at end-systole ( ML s) and end-diastole ( ML d). Because MLs is smaller than MLd, peak GLS is a negative number. This negative nature of GLS can lead to confusion when describing increases or decreases in strain. We recommend that all references to strain changes specifically mention an increase or decrease in the absolute value of strain, to avoid confusion.
After optimizing image quality, maximizing frame rate, and minimizing foreshortening, which are all critical to reduce measurement variability, GLS measurements should be made in the three standard apical views and averaged. Measurements should begin with the apical long-axis view to visualize aortic valve closure, using opening and closing clicks of the aortic valve or aortic valve opening and closing on M-mode imaging. When regional tracking is suboptimal in more than two myocardial segments in a single view, the calculation of GLS should be avoided. In such cases, alternative indices may be used to gain insight into longitudinal LV function, such as mitral annular plane systolic excursion or pulsed Doppler tissue imaging (DTI)–derived mitral annular peak systolic velocity (s′).
There are concurrent definitions as a basis for GLS calculation using endocardial, midwall, or average deformation. This committee refrains from recommendations in this regard and refers to the ongoing joint standardization initiative of the ASE, EACVI, and the ultrasound imaging industry. Because of intervendor and intersoftware variability and age and load dependency, serial assessment of GLS in individual patients should be performed using the same vendor’s equipment and the same software.
The preponderance of currently available data is for midwall GLS. Although the evidence base for its use in routine clinical echocardiography is far smaller than that for EF, measures of midwall GLS have been shown in several studies to be robust and reproducible and to offer incremental predictive value in unselected patients undergoing echocardiography for the assessment of resting function, as well as in predicting postoperative LV function in patients with valve disease.
2.4
Normal Reference Values
Normal reference values for LV EF derived from 2DE have been updated using the population-based studies described in section 1.3 above. Details can be found in Tables 2 and 4 and Supplemental Tables 2-5 (see Appendix ). EF is not significantly related to gender, age, or body size, as measured by BSA. Normal EF was 63 ± 5% using the biplane method of disks. Therefore, in individuals aged > 20 years, EF in the range of 53% to 73% should be classified as normal. Three-dimensional echocardiographic normal values have been recently reported in different ethnic populations ( Table 3 ).
Normal values for GLS depend on the definition of the measurement position in the myocardium, the vendor, and the version of the analysis software, resulting in considerable heterogeneity in the published literature. It is the consensus of this writing committee that differences among vendors and software packages are still too large to recommend universal normal values and lower limits of normal. To provide some guidance, a peak GLS in the range of −20% can be expected in a healthy person. A selection of recently published data is provided in the Appendix together with the lower normal limits ( Supplemental Table 6 ). There is evidence that women have slightly higher absolute values of GLS than men and that strain values decrease with age. GLS is a valuable and sensitive tool for follow-up examinations, provided the same equipment, tracing methodology, and software are used.
Recommendations
LV systolic function should be routinely assessed using 2DE or 3DE by calculating EF from EDV and ESV. LV EFs of <52% for men and <54% for women are suggestive of abnormal LV systolic function. Two-dimensional STE-derived GLS appears to be reproducible and feasible for clinical use and offers incremental prognostic data over LV EF in a variety of cardiac conditions, although measurements vary among vendors and software versions. To provide some guidance, a peak GLS in the range of −20% can be expected in a healthy person, and the lower the absolute value of strain is below this value, the more likely it is to be abnormal.
3
LV Regional Function
3.1
Segmentation of the Left Ventricle
For the assessment of regional LV function, the ventricle is divided into segments. Segmentation schemes should reflect coronary perfusion territories, result in segments with comparable myocardial mass, and allow standardized communication within echocardiography and with other imaging modalities ( Figure 3 ). Accordingly, a 17-segment model is commonly used. Beginning at the anterior junction of the interventricular septum and the RV free wall and continuing counterclockwise, basal and midventricular segments should be labeled as anteroseptal, inferoseptal, inferior, inferolateral, anterolateral, and anterior. In this 17-segment model, the apex is divided into five segments, including septal, inferior, lateral, and anterior segments, as well as the “apical cap,” which is defined as the myocardium beyond the end of the LV cavity ( Figures 3 and 4 ). The 17-segment model may be used for myocardial perfusion studies or when comparing between different imaging modalities, specifically single photon-emission computed tomography, positron emission tomography, and CMR. Figure 5 shows a schematic representation of the perfusion territories of the three major coronary arteries. When using this 17-segment model to assess wall motion or regional strain, the 17th segment (the apical cap) should not be included.
Alternative segmentation models treat the apex differently: the 16-segment model divides the entire apex into the same four segments (septal, inferior, lateral, and anterior; Figure 3 , left). Also, some segmentation schemes divide the apex into six segments, similar to the basal and midventricular levels, resulting in an 18-segment model ( Figure 3 , right) that is simple but results in a slight overrepresentation of the distal myocardium when scoring.
All segments can be visualized by 2DE. On average, the two-chamber view and the apical long-axis view intersect with the four-chamber view at angles of approximately 53° and 129°, respectively, allowing the assessment of the central region of all segments from an apical window, independent of the model used. Although certain variability exists in the coronary artery blood supply to myocardial segments, segments are usually attributed to the three major coronary arteries ( Figure 5 ).
3.2
Visual Assessment
In echocardiography, regional myocardial function is assessed on the basis of the observed wall thickening and endocardial motion of the myocardial segment. Because myocardial motion may be caused by adjacent segment tethering or overall LV displacement, regional deformation (thickening, shortening) should be the focus of the analysis. However, it must be recognized that deformation can also be passive and therefore may not always accurately reflect myocardial contraction.
It is recommended that each segment be analyzed individually in multiple views. A semiquantitative wall motion score can be assigned to each segment to calculate the LV wall motion score index as the average of the scores of all segments visualized. The following scoring system is recommended: (1) normal or hyperkinetic, (2) hypokinetic (reduced thickening), (3) akinetic (absent or negligible thickening, e.g., scar), and (4) dyskinetic (systolic thinning or stretching, e.g., aneurysm).
An aneurysm is a morphologic entity that demonstrates focal dilatation and thinning (remodeling) with either akinetic or dyskinetic systolic deformation. In contrast to the recommendation of previous guidelines, this committee refrains from assigning a separate wall motion score for aneurysm.
3.3
Regional Wall Motion during Infarction and Ischemia
Depending on the regional coronary flow reserve, stress echocardiography may reveal significant coronary artery stenoses by means of inducing a wall motion abnormality. Myocardial scar may also result in regional dysfunction of variable severity. Echocardiography can over- or underestimate the amount of ischemic or infarcted myocardium, depending on the function of adjacent regions, regional loading conditions, and stunning. In stress echocardiography, visual recognition of regional dysfunction can be improved with a synchronized side-by-side comparison of baseline and stress images using digital technology.
3.4
Regional Abnormalities in the Absence of Coronary Artery Disease
Regional wall motion abnormalities may also occur in the absence of coronary artery disease, in a variety of conditions, such as myocarditis, sarcoidosis, and stress-induced (takotsubo) cardiomyopathy. Abnormal motion patterns of the interventricular septum may be found postoperatively or in the presence of a left bundle branch block or RV epicardial pacing, as well as RV dysfunction caused by RV pressure or volume overload. Furthermore, some conduction delays can cause regional wall motion abnormalities in the absence of primary myocardial dysfunction. This regional dysfunction is due to the abnormal sequence of myocardial activation, which causes heterogeneous loading conditions and remodeling. Ideally, the temporal sequence of activation and motion should be described. Characteristic motion patterns, which result from abnormal activation sequences, such as septal bounce (“beaking,” “flash”) or lateral apical motion during systole (“apical rocking”) should be reported.
3.5
Quantification of Regional Wall Motion Using Doppler and STE
Echocardiographic quantification of regional myocardial function is currently based on DTI or speckle-tracking echocardiographic techniques. Both techniques provide comparable data quality, although DTI is known to be angle dependent and prone to underestimating motion that is not parallel to the ultrasound beam. Commonly used parameters include velocity, motion, deformation, and deformation rate. Because velocity and motion are measured relative to the transducer, measurements may be influenced by tethering or overall heart motion. Accordingly, the use of deformation parameters, such as strain and strain rate, is preferable.
The most commonly used deformation parameter is longitudinal strain during LV systole. Similar to global strain, with current technology, regional deformation measurements may vary in amplitude, depending on the myocardial region being investigated, the measurement methodology, the vendor, and sample volume definition. Therefore, no specific normal ranges are provided in this document. These values await the upcoming consensus document of the joint task force of the ASE, EACVI, and the industry for the standardization of quantitative function imaging.
Independent of strain magnitude, characteristic changes in temporal pattern of myocardial deformation can be assessed as well. Longitudinal shortening or radial thickening of the myocardium after aortic valve closure (postsystolic shortening or thickening, sometimes referred to as tardokinesis) of >20% of the total deformation during the cardiac cycle is a consistent sign of regional functional inhomogeneity (e.g., ischemia, scar). The development of postsystolic shortening during a stress test has been proposed as an indicator of regional ischemia. The value of regional deformation parameters and temporal patterns of strain derived by speckle-tracking from either 2D or 3D echocardiographic data sets is the subject of ongoing research and remains to be determined.
Recommendations
Different LV segmentation models are used in clinical practice. The 17-segment model is recommended to assess myocardial perfusion with echocardiography and other imaging techniques. The 16-segment model is recommended for routine studies assessing wall motion, because endocardial excursion and thickening of the tip of the apex are imperceptible. To assess wall motion, each segment should be evaluated in multiple views and a four-grade scoring should be applied: (1) normal or hyperkinetic, (2) hypokinetic (reduced thickening), (3) akinetic (absent or negligible thickening), and (4) dyskinetic (systolic thinning or stretching). Despite promising data, quantitative assessment of the magnitude of regional LV deformation cannot be recommended at this stage because of lack of reference values, suboptimal reproducibility, and considerable intervendor measurement variability.
4
LV Mass
LV mass is an important risk factor for, and a strong predictor of, cardiovascular events. There are several methods that effectively calculate LV mass from M-mode echocardiography, 2DE, and 3DE ( Table 5 ). All measurements should be performed at the end of diastole (the frame before mitral valve closure or the frame in the cardiac cycle in which the ventricular dimension or volume is largest). Those that use M-mode (either blinded or 2D-guided) and 2D echocardiographic linear measurements of LV diastolic diameter and wall thickness rely on geometric formulas to calculate the volume of LV myocardium, while 3DE can measure it directly. All methods then convert the volume to mass by multiplying the volume of myocardium by the myocardial density (approximately 1.05 g/mL).
When the entire ventricle is measured from 2D echocardiographic images, either the area-length or truncated ellipsoid technique is used. Each method for LV mass measurement has advantages, disadvantages, and value in specific situations ( Table 5 ).
To measure LV mass in an individual patient over time, especially those with cardiac disease, the 2D echocardiographic methods have advantages compared with the linear dimension technique. There are, however, fewer studies of the prognostic value of LV mass calculated by these methods compared with the linear dimension method described below. Unlike the linear dimension or M-mode method, the 2D echocardiographic methods can accommodate for the shape of the ventricle and account for changes in LV size that might occur along the long axis of the chamber. This is an important consideration, because changes in LV geometry are common in various cardiac diseases.
However, when there is a need to screen or study large populations, the M-mode method has advantages, because it is simple, quick, and subject to less measurement variability. There is a large body of evidence to support the accuracy of this method. Most studies that relate LV mass to prognosis are based on this method. However, several caveats need to be mentioned. First, it is critical that the wall thickness and LV dimensions measured be truly perpendicular to the long axis of the left ventricle. Therefore, 2D-guided M-mode imaging or measurements from 2D echocardiographic images are preferred over blind M-mode imaging. Second, the formula includes a correction for the 20% overestimation that was found during the original validation studies of the M-mode technique. Because direct 2D measures of wall thickness may yield smaller values than the M-mode technique, LV mass calculated using this formula may not be directly interchangeable ( Table 5 ). This may be a less important consideration if the method is being used to identify cutoff values for prognosis. It is also important to note that the formula raises the linear dimensions to the power of 3, and thus even small errors in dimensions can have significant effects on the calculated LV mass.
Most studies that have compared 2D-guided M-mode measurements of LV mass with the 2D echocardiographic area-length or truncated ellipsoid methods in normally shaped ventricles have shown subtle differences but no clear advantage of one technique over the other. However, comparison studies have not been performed in the current era, when tremendous gains in 2D echocardiographic image quality have been made. In fact, large population studies confirming or reestablishing normal values for LV mass with harmonic imaging are limited.
Because 3DE is the only echocardiographic method that directly measures myocardial volume, it is an appropriate approach. Numerous validation studies have been performed. However, to date, there have been few studies assessing its practical use, feasibility, variability, or prognostic value in large-scale clinical environments. Accordingly, it is the consensus of this committee that the 3D echocardiographic LV mass data available in normal subjects are not sufficient to recommend normal reference values. It must also be noted that continuous improvements in the spatial and temporal resolution of 3D echocardiographic imaging will also influence normal values and measurement variability.
In patients with upper septal hypertrophy, the linear dimension methods, which use basal ventricular measurements, result in overestimation of the true mass, because the thickest region of the interventricular septum is incorporated in the measurement. In contrast, the area-length method, which uses mid-ventricular measurements, underestimates LV mass, because the thickest part of the interventricular septum is not included in the measurement. In the setting of discrete upper septal or asymmetric hypertrophy, if these methods are used to serially assess LV mass in a patient, it is critical to use the same methodology over time and to measure the walls at the same level of the ventricle. The 3D method has the advantage of accommodating regional differences in wall thickness and therefore can provide the most accurate measurements of LV mass in this setting.
The values for LV mass vary according to gender, age, body size, obesity, and region of the world. Therefore, uniform reference values are difficult to define. LV mass is higher in men independent of body size and increases with body size. Since the publication of the 2005 recommendations, several studies, mostly using linear measurements, have reported normal values of LV mass in normal populations. The larger studies reported values close to those recommended in the previous guidelines. Therefore, the same reference values and abnormality partition cutoffs as reported in the previous guidelines continue to be recommended ( Table 6 ). However, characterization of the population being studied, and differences in mass between different ethnic populations should be taken into account when determining normal values.
Women | Men | |
---|---|---|
Linear method | ||
LV mass (g) | 67–162 | 88–224 |
LV mass/BSA (g/m 2 ) | 43–95 | 49–115 |
Relative wall thickness (cm) | 0.22–0.42 | 0.24–0.42 |
Septal thickness (cm) | 0.6–0.9 | 0.6–1.0 |
Posterior wall thickness (cm) | 0.6–0.9 | 0.6–1.0 |
2D method | ||
LV mass (g) | 66–150 | 96–200 |
LV mass/BSA (g/m 2 ) | 44–88 | 50–102 |
The indexing of LV mass allows comparisons in subjects with different body sizes. However, whether to use height, weight, or BSA as the indexing term remains controversial. Studies suggest that indexing to height raised to allometric powers such as 1.7, 2.13, and 2.7 has advantages over indexing to BSA, especially when attempting to predict events in obese patients. However most large population studies reporting LV mass have indexed to BSA.
Finally, calculation of relative wall thickness (RWT) with the formula (2 × posterior wall thickness)/(LV internal diameter at end-diastole) permits categorization of an increase in LV mass as either concentric (RWT > 0.42) or eccentric (RWT ≤ 0.42) hypertrophy and allows the identification of concentric remodeling (normal LV mass with increased RWT) ( Figure 6 ).
Recommendations
In the normally shaped left ventricle, both M-mode and 2D echocardiographic formulas to calculate LV mass can be used. Normal values for these techniques remain unchanged from the previous guidelines and should be reported indexed to BSA. Reference upper limits of normal LV mass by linear measurements are 95 g/m 2 in women and 115 g/m 2 in men. Reference upper limits of normal LV mass by 2D measurements are 88 g/m 2 in women and 102 g/m 2 in men with 2D methods. Because 3DE is the only echocardiographic technique that measures myocardial volume directly, without geometric assumptions regarding LV shape and distribution of wall thickening, this technique is promising and may be used in abnormally shaped ventricles or in patients with asymmetric or localized hypertrophy. Limited upper normal limits of 3D echocardiographic LV mass data are currently available in the literature but are insufficient to substantiate recommendations for reference values.
I
The Left Ventricle
1
Measurement of LV Size
The most commonly used parameters to describe LV cavity size include linear internal dimensions and volumes. Measurements are commonly reported for end-diastole and end-systole, which are then used to derive parameters of global LV function. To allow comparison among individuals with different body sizes, chamber measurements should be reported indexed to BSA.
1.1
Linear Measurements
It is recommended that linear internal measurements of the left ventricle and its walls be performed in the parasternal long-axis view. Values should be carefully obtained perpendicular to the LV long axis and measured at or immediately below the level of the mitral valve leaflet tips. In this regard, the electronic calipers should be positioned on the interface between the myocardial wall and cavity and the interface between the wall and the pericardium. Internal dimensions can be obtained with a two-dimensional (2D) echocardiography (2DE)–guided M-mode approach, although linear measurements obtained from 2D echocardiographic images are preferred to avoid oblique sections of the ventricle ( Table 1 ).
1.2
Volumetric Measurements
LV volumes are measured using 2DE or 3DE. Volume calculations derived from linear measurements may be inaccurate, because they rely on the assumption of a fixed geometric LV shape such as a prolate ellipsoid, which does not apply in a variety of cardiac pathologies. Accordingly, the Teichholz and Quinones methods for calculating LV volumes from LV linear dimensions are no longer recommended for clinical use.
Volumetric measurements are usually based on tracings of the interface between the compacted myocardium and the LV cavity. At the mitral valve level, the contour is closed by connecting the two opposite sections of the mitral ring with a straight line. LV length is defined as the distance between the bisector of this line and the apical point of the LV contour, which is most distant to it. The use of the longer LV length between the apical two- and four-chamber views is recommended.
LV volumes should be measured from the apical four- and two-chamber views. Two-dimensional echocardiographic image acquisition should aim to maximize LV areas, while avoiding foreshortening of the left ventricle, which results in volume underestimation. Acquiring LV views at a reduced depth to focus on the LV cavity will reduce the likelihood of foreshortening and minimize errors in endocardial border tracings ( Table 1 ). Because the issue of foreshortening is less relevant in 3D data sets, 3D image acquisition should focus primarily on including the entire left ventricle within the pyramidal data set. To ensure reasonably accurate identification of end-systole, the temporal resolution of 3D imaging should be maximized without compromising spatial resolution.
Contrast agents should be used when needed to improve endocardial delineation when two or more contiguous LV endocardial segments are poorly visualized in apical views, as per published guidelines. Contrast-enhanced images may provide larger volumes than unenhanced images that are closer to those obtained with cardiac magnetic resonance (CMR) in head-to-head comparison. Care should be taken to avoid acoustic shadowing, which may occur in LV basal segments in the presence of high concentrations of contrast. Normal reference values for LV volumes with contrast enhancement are not well established.
The most commonly used method for 2D echocardiographic volume calculations is the biplane method of disks summation (modified Simpson’s rule), which is the recommended 2D echocardiographic method by consensus of this committee ( Table 1 ). An alternative method to calculate LV volumes when apical endocardial definition precludes accurate tracing is the area-length method, in which the LV is assumed to be bullet shaped. The mid-LV cross-sectional area is computed by planimetry in the parasternal short-axis view and the length of the ventricle taken from the midpoint of the annular plane to the apex in the apical four-chamber view ( Table 1 ). The shortcoming of this method is that the bullet-shape assumption does not always hold true. One of the advantages of 3D echocardiographic volume measurements is that they do not rely on geometric assumptions. In patients with good image quality, 3D echocardiographic measurements are accurate and reproducible and should therefore be used when available and feasible. The advantages and disadvantages of the various methods are summarized in Table 1 .
1.3
Normal Reference Values for 2DE
Data were extracted from seven databases, including Asklepios (year 0 and year 10), Flemengho, CARDIA5 and CARDIA25, Padua 3D Echo Normal, and the Normal Reference Ranges for Echocardiography study, to obtain reference values in normal subjects for the left ventricle and the left atrium (see section 10). All data were obtained without the use of contrast agents. Data sets for all patients included age, gender, ethnicity, height, and weight. To ensure a normal population, subjects in these studies were excluded if any of the following criteria were met: systolic blood pressure > 140 mm Hg, diastolic blood pressure > 80 mm Hg, history of drug-treated hypertension, diagnosis of diabetes, impaired fasting glucose > 100 mg/dL, body mass index > 30 kg/m 2 , creatinine > 1.3 mg/dL, estimated glomerular filtration rate < 60 mL/min/1.73 m 2 , total cholesterol > 240 mg/dL, low-density lipoprotein cholesterol > 130 mg/dL, and total triglycerides > 150 mg/dL. Details of the statistical analysis are described in the Appendix . Because of varied study aims, not all echocardiographic measurements were available for each database. Supplemental Table 1 summarizes the sources of the data for each measurement group and their baseline characteristics.
Table 2 shows the normal values for 2D echocardiographic parameters of LV size and function according to gender, while Supplemental Table 2 provides expanded data for the same parameters, obtained from different echocardiographic views, and also includes the corresponding number of subjects used to obtain these data. Supplemental Table 3 lists normal ranges and consensus-based partition cutoffs for LV dimensions, volumes, EF, and mass. On multivariate analysis, age, gender, and BSA were found to have a significant independent influence on LV end-diastolic volume (EDV) and LV end-systolic volume (ESV). The results across genders and age deciles subdivided into absolute and BSA-normalized values are shown in Supplemental Table 4 (see Appendix ).
Parameter | Male | Female | ||
---|---|---|---|---|
Mean ± SD | 2-SD range | Mean ± SD | 2-SD range | |
LV internal dimension | ||||
Diastolic dimension (mm) | 50.2 ± 4.1 | 42.0–58.4 | 45.0 ± 3.6 | 37.8–52.2 |
Systolic dimension (mm) | 32.4 ± 3.7 | 25.0–39.8 | 28.2 ± 3.3 | 21.6–34.8 |
LV volumes (biplane) | ||||
LV EDV (mL) | 106 ± 22 | 62–150 | 76 ± 15 | 46–106 |
LV ESV (mL) | 41 ± 10 | 21–61 | 28 ± 7 | 14–42 |
LV volumes normalized by BSA | ||||
LV EDV (mL/m 2 ) | 54 ± 10 | 34–74 | 45 ± 8 | 29–61 |
LV ESV (mL/m 2 ) | 21 ± 5 | 11–31 | 16 ± 4 | 8–24 |
LV EF (biplane) | 62 ± 5 | 52–72 | 64 ± 5 | 54–74 |
Because ethnicity is an important factor, results of analysis by race and gender are presented in Supplemental Table 5 . From the regression analysis, nomograms are provided for plotting observed LV dimensions versus BSA or BSA-indexed LV volumes versus age ( Figures 1 and 2 ). Nomograms for absolute LV measurements against age ( Supplemental Figures 1 and 2 ) and BSA ( Supplemental Figures 3 and 4 ) are also provided (see Appendix ).
1.4
Normal Reference Values for 3DE
Several studies have published 3D echocardiographic reference values for healthy normotensive subjects, which are summarized in Table 3 . The reported variations in the normal ranges from study to study are likely due to differences in populations, echocardiographic equipment, and analysis software, as well as variability in measurement techniques. In patients with good image quality, the accuracy of 3DE is comparable with that of CMR, although volumes tend to be lower on echocardiography.
Aune et al . (2010) | Fukuda et al . (2012) | Chahal et al . (2012) | Muraru et al . (2013) | |
---|---|---|---|---|
Number of subjects | 166 | 410 | 978 | 226 |
Ethnic makeup of population | Scandinavian | Japanese | 51% European white, 49% Asian Indian | White European |
EDVi (mL/m 2 ) | ||||
Men, mean (LLN, ULN) | 66 (46, 86) | 50 (26, 74) | White: 49 (31, 67); Indian: 41 (23, 59) | 63 (41, 85) |
Women, mean (LLN, ULN) | 58 (42, 74) | 46 (28, 64) | White: 42 (26, 58); Indian: 39 (23, 55) | 56 (40, 78) |
ESVi (mL/m 2 ) | ||||
Men, mean (LLN, ULN) | 29 (17, 41) | 19 (9, 29) | White: 19 (9, 29); Indian: 16 (6, 26) | 24 (14, 34) |
Women, mean (LLN, ULN) | 23 (13, 33) | 17 (9, 25) | White: 16 (8, 24); Indian: 15 (7, 23) | 20 (12, 28) |
EF (%) | ||||
Men, mean (LLN, ULN) | 57 (49, 65) | 61 (53, 69) | White: 61 (49, 73); Indian: 62 (52, 72) | 62 (54, 70) |
Women, mean (LLN, ULN) | 61 (49, 73) | 63 (55, 71) | White: 62 (52, 72); Indian: 62 (52, 72) | 65 (57, 73) |
The effects of ethnicity on 3D echocardiographic LV volumes were investigated in one study, which reported that LV volumes were smaller among Asian Indians than white Europeans, but EF did not differ among ethnic groups. In most 3D echocardiographic studies, the relationship between age and 3D echocardiographic LV volumes was examined, and weak to moderate negative correlations were seen between age and LV volumes, while EF did not change significantly with age. This finding is similar to those described in the CMR literature. On the basis of weighted averages of three studies, 3D echocardiographic LV volumes were larger than 2D echocardiographic values, and corresponding upper limits of the normal range were EDVs of 79 mL/m 2 for men and 71 mL/m 2 for women and ESVs of 32 mL/m 2 for men and 28 mL/m 2 for women. Ultimately, a large study in a diverse population will be needed to establish normal reference ranges for 3DE for different ethnic groups.
Recommendation
LV size should be routinely assessed on 2DE by calculating volumes using the biplane method of disks summation technique. In laboratories with experience in 3DE, 3D measurement and reporting of LV volumes is recommended when feasible depending on image quality. When reporting LV linear dimensions, the recommended method is 2D-guided measurements. LV size and volume measurements should be reported indexed to BSA. For general reference, 2D echocardiographic LV EDVs of 74 mL/m 2 for men and 61 mL/m 2 for women and LV ESVs of 31 mL/m 2 for men and 24 mL/m 2 for women should be used as the upper limits of the corresponding normal range.
2
LV Global Systolic Function
Global LV function is usually assessed by measuring the difference between the end-diastolic and end-systolic value of a one-dimensional, 2D, or 3D parameter divided by its end-diastolic value. For this, end-diastole is preferably defined as the first frame after mitral valve closure or the frame in the cardiac cycle in which the respective LV dimension or volume measurement is the largest. End-systole is best defined as the frame after aortic valve closure or the frame in which the cardiac dimension or volume is smallest. In patients with regular heart rhythm, measurements of the timing of valve openings and closures derived from M-mode echocardiography, pulsed-wave (PW) or continuous-wave Doppler may be used for accurate definitions of ventricular time intervals.
2.1
Fractional Shortening
Fractional shortening can be derived from 2D-guided M-mode imaging or preferably from linear measurements obtained from 2D images. Deriving global LV function parameters from linear measurements is problematic when there are regional wall motion abnormalities due to coronary disease or conduction abnormalities. In patients with uncomplicated hypertension, obesity or valvular diseases, such regional differences are rare in the absence of clinically recognized myocardial infarction, and accordingly, this parameter may provide useful information in clinical studies. In patients with normal size of the LV base but enlarged midventricular and distal portions, LV volume would be a better marker of LV size than linear dimension measured at the LV base.
2.2
EF
EF is calculated from EDV and ESV estimates, using the following formula:
LV volume estimates may be derived from 2DE or 3DE, as described above (section 1.2). The biplane method of disks (modified Simpson’s rule) is the currently recommended 2D method to assess LV EF by consensus of this committee. Table 4 lists 2DE-derived biplane LV EF, including normal ranges and consensus-based severity partition cutoffs according to gender. In patients with good image quality, 3DE-based EF measurements are accurate and reproducible and should be used when available and feasible.
Male | Female | |||||||
---|---|---|---|---|---|---|---|---|
Normal range | Mildly abnormal | Moderately abnormal | Severely abnormal | Normal range | Mildly abnormal | Moderately abnormal | Severely abnormal | |
LV EF (%) | 52–72 | 41–51 | 30–40 | <30 | 54–74 | 41–53 | 30–40 | <30 |
Maximum LA volume/BSA (mL/m 2 ) | 16–34 | 35–41 | 42–48 | >48 | 16–34 | 35–41 | 42–48 | >48 |
2.3
Global Longitudinal Strain (GLS)
Lagrangian strain is defined as the change in length of an object within a certain direction relative to its baseline length:
where L t is the length at time t , and L 0 is the initial length at time 0. The most commonly used strain-based measure of LV global systolic function is GLS. It is usually assessed by speckle-tracking echocardiography (STE) ( Table 1 ). On 2DE, peak GLS describes the relative length change of the LV myocardium between end-diastole and end-systole:
where ML is myocardial length at end-systole ( ML s) and end-diastole ( ML d). Because MLs is smaller than MLd, peak GLS is a negative number. This negative nature of GLS can lead to confusion when describing increases or decreases in strain. We recommend that all references to strain changes specifically mention an increase or decrease in the absolute value of strain, to avoid confusion.
After optimizing image quality, maximizing frame rate, and minimizing foreshortening, which are all critical to reduce measurement variability, GLS measurements should be made in the three standard apical views and averaged. Measurements should begin with the apical long-axis view to visualize aortic valve closure, using opening and closing clicks of the aortic valve or aortic valve opening and closing on M-mode imaging. When regional tracking is suboptimal in more than two myocardial segments in a single view, the calculation of GLS should be avoided. In such cases, alternative indices may be used to gain insight into longitudinal LV function, such as mitral annular plane systolic excursion or pulsed Doppler tissue imaging (DTI)–derived mitral annular peak systolic velocity (s′).
There are concurrent definitions as a basis for GLS calculation using endocardial, midwall, or average deformation. This committee refrains from recommendations in this regard and refers to the ongoing joint standardization initiative of the ASE, EACVI, and the ultrasound imaging industry. Because of intervendor and intersoftware variability and age and load dependency, serial assessment of GLS in individual patients should be performed using the same vendor’s equipment and the same software.
The preponderance of currently available data is for midwall GLS. Although the evidence base for its use in routine clinical echocardiography is far smaller than that for EF, measures of midwall GLS have been shown in several studies to be robust and reproducible and to offer incremental predictive value in unselected patients undergoing echocardiography for the assessment of resting function, as well as in predicting postoperative LV function in patients with valve disease.
2.4
Normal Reference Values
Normal reference values for LV EF derived from 2DE have been updated using the population-based studies described in section 1.3 above. Details can be found in Tables 2 and 4 and Supplemental Tables 2-5 (see Appendix ). EF is not significantly related to gender, age, or body size, as measured by BSA. Normal EF was 63 ± 5% using the biplane method of disks. Therefore, in individuals aged > 20 years, EF in the range of 53% to 73% should be classified as normal. Three-dimensional echocardiographic normal values have been recently reported in different ethnic populations ( Table 3 ).
Normal values for GLS depend on the definition of the measurement position in the myocardium, the vendor, and the version of the analysis software, resulting in considerable heterogeneity in the published literature. It is the consensus of this writing committee that differences among vendors and software packages are still too large to recommend universal normal values and lower limits of normal. To provide some guidance, a peak GLS in the range of −20% can be expected in a healthy person. A selection of recently published data is provided in the Appendix together with the lower normal limits ( Supplemental Table 6 ). There is evidence that women have slightly higher absolute values of GLS than men and that strain values decrease with age. GLS is a valuable and sensitive tool for follow-up examinations, provided the same equipment, tracing methodology, and software are used.
Recommendations
LV systolic function should be routinely assessed using 2DE or 3DE by calculating EF from EDV and ESV. LV EFs of <52% for men and <54% for women are suggestive of abnormal LV systolic function. Two-dimensional STE-derived GLS appears to be reproducible and feasible for clinical use and offers incremental prognostic data over LV EF in a variety of cardiac conditions, although measurements vary among vendors and software versions. To provide some guidance, a peak GLS in the range of −20% can be expected in a healthy person, and the lower the absolute value of strain is below this value, the more likely it is to be abnormal.
3
LV Regional Function
3.1
Segmentation of the Left Ventricle
For the assessment of regional LV function, the ventricle is divided into segments. Segmentation schemes should reflect coronary perfusion territories, result in segments with comparable myocardial mass, and allow standardized communication within echocardiography and with other imaging modalities ( Figure 3 ). Accordingly, a 17-segment model is commonly used. Beginning at the anterior junction of the interventricular septum and the RV free wall and continuing counterclockwise, basal and midventricular segments should be labeled as anteroseptal, inferoseptal, inferior, inferolateral, anterolateral, and anterior. In this 17-segment model, the apex is divided into five segments, including septal, inferior, lateral, and anterior segments, as well as the “apical cap,” which is defined as the myocardium beyond the end of the LV cavity ( Figures 3 and 4 ). The 17-segment model may be used for myocardial perfusion studies or when comparing between different imaging modalities, specifically single photon-emission computed tomography, positron emission tomography, and CMR. Figure 5 shows a schematic representation of the perfusion territories of the three major coronary arteries. When using this 17-segment model to assess wall motion or regional strain, the 17th segment (the apical cap) should not be included.
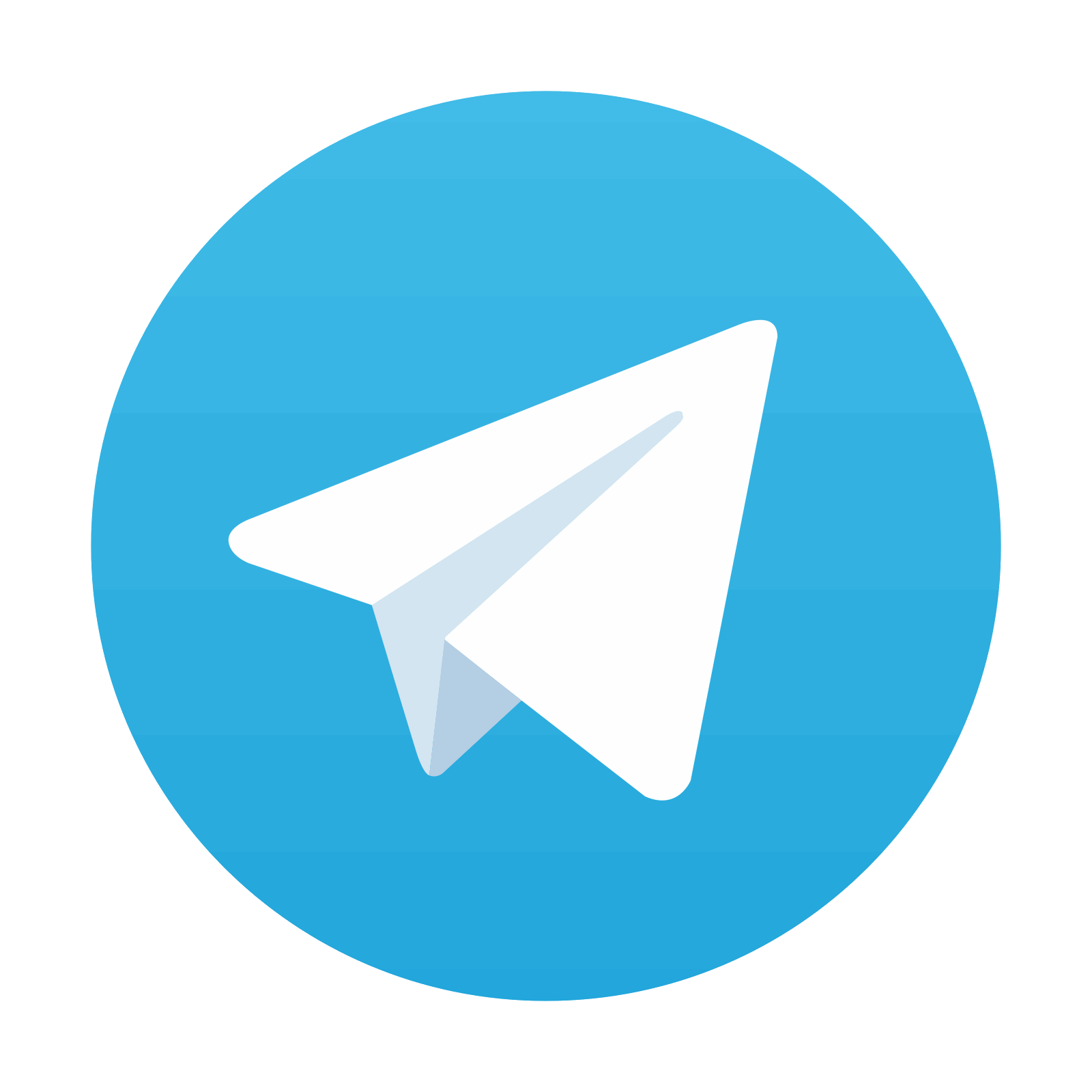
Stay updated, free articles. Join our Telegram channel

Full access? Get Clinical Tree
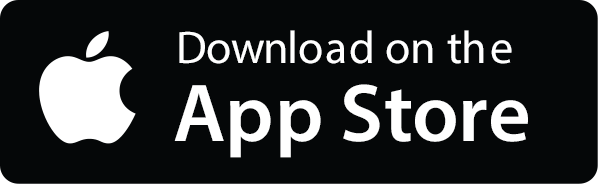
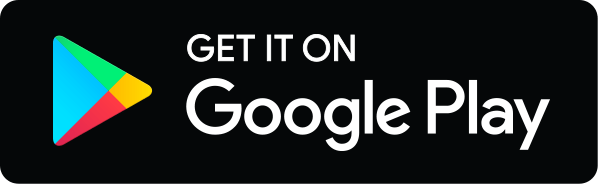
