This chapter reviews the most important characteristics of the various single-photon emission computed tomography (SPECT) and positron emission tomography (PET) radiopharmaceuticals that are currently used in clinical practice. Myocardial perfusion scintigraphy has significantly evolved1 since its introduction more than four decades ago. Three major factors have specifically contributed to this evolution: (1) technical improvements in scintigraphic data acquisition and analysis, (2) introduction of new technetium-99m (Tc-99m)-labeled MPI radiopharmaceuticals with different properties than thallium-201 (Tl-201), and (3) availability of PET radiotracers and PET-dedicated cameras. This chapter reviews the most important characteristics of the various SPECT and PET MPI radioactive agents that are currently used in clinical practice. The knowledge of the characteristics and kinetics of the various radiotracers is clinically relevant and essential for designing and implementing optimized imaging protocols.2 The optimal timing of imaging after injection either at stress or at rest is determined by rate of uptake in the heart and adjacent organs, as well as the residence time of radiotracers within the myocytes. The efficiency of myocardial extraction over a wide range myocardial blood flows is relevant for reliable detection of obstructive coronary artery disease and absolute quantification of regional myocardial blood flow. Therefore, knowledge of the basic characteristics of MPI radiotracers is essential for optimal clinical use of these agents. In addition, a section on 99mTc-pyrophosphate cardiac imaging is included, given its role in the diagnosis of cardiac amyloidosis, while other more specific agents such as 123I-MIBG and 123I-IPPA are covered in another chapter (Chapter 23). Radiation safety has become a more sensitive concern more recently. Therefore, data on radiation dosimetry are also discussed for each radiopharmaceutical.
Although many different classes of radioactive MPI agents exist, they should all present a minimum of common basic characteristics.4
The myocardial uptake of the radiotracer must be proportional to the regional myocardial blood flow over a relatively wide range of blood flows.
The myocardial uptake should be high enough to allow for detection of regional inhomogeneity by external gamma scintigraphy.
The initial myocardial distribution of the radiotracer at the time of injection must remain stable during the acquisition time of the images.
The effect of blood flow on myocardial transport of the radiotracer must be predominant to the effect of metabolic cellular alterations.
Finally, the agent should be labeled to a radionuclide having adequate physical characteristics to provide high photon flux and optimal counting statistics.
Information on basic properties of radionuclide MPI agents is generally obtained from cultured myocardial cells, isolated perfused hearts, or in vivo animal models.5 Precise measurements of cellular or capillary-tissue tracer kinetics are usually obtained from cell cultures and isolated perfused heart models, whereas regional tracer distribution and uptake in other organs are studied with in vivo animal models. The two most important physiologic factors that affect the myocardial uptake of an MPI agent are the variations in regional myocardial blood flow and the myocardial extraction of the radiotracer. Three major parameters (Enet, Emax, and PS cap) can be determined using indicator-dilution techniques and radiolabeled albumin used as an intravascular reference. The difference between the intravascular albumin reference and the radiopharmaceutical that is evaluated (i.e., a diffusible perfusion agent) on a venous dilution curve is used to calculate its instantaneous cardiac extraction. The early peak of the curve, or Emax, represents the maximum fractional tissue extraction of the diffusible agent. This value is used to calculate the capillary permeability surface area product, PS cap. The net extraction (Enet) is the integral of the curve and is used as a measure of myocardial radiotracer retention, including both initial extraction and subsequent back-diffusion. A high value for Emax and PS cap indicates a rapid blood-tissue exchange and suggests that the diffusible radiotracer will be able to assess high levels of hyperemic flow accurately. Table 3-1 summarizes and compares these values for the commonly used radiopharmaceuticals.
Tl–201 | Tc-99m-Sestamibi | Tc-99m-Tetrofosmin | |
---|---|---|---|
Class | Element | Isonitrile | Diphosphine |
Charge | Cation | Cation | Cation |
Emax | 0.73 ± 0.10 | 0.38 ± 0.09 | 0.32 ± 0.07 |
Enet | 0.57 ± 0.13 | 0.41 ± 0.15 | 0.23 |
PS cap (mL/mg) | 1.30 ± 0.45 | 0.44 ± 0.23 | 0.40 |
Myocardial redistribution | Yes | Very partial | No |
Myocardial uptake (%ID) | 3.0–4.0 | 1.4 ± 0.3 | 1.0–1.2 |
IV imaging interval | 5–10 minutes | 15–60 minutes | 15 minutes |
Target organ | Kidney | Upper large intestine | Gallbladder wall |
The criteria necessary for a radiopharmaceutical to be used to determine regional perfusion were elaborated by Saperstein in the mid-1950s.3 As described by the Saperstein principle, a given type of radiopharmaceutical will be distributed in proportion to regional perfusion if its extraction by the organ of interest is high and if its clearance from the blood is rapid. One of the first classes of radiopharmaceuticals used to image myocardial perfusion was the potassium analogs—cesium, rubidium, and thallium, which enter the myocardium by the sodium–potassium–ATPase pump mechanism. Although different radioisotopes of these cations have been evaluated, Tl-201 was found to have the best physical and biological characteristics for imaging and has been the most popular radionuclide MPI agent used in noninvasive detection and evaluation of patients with known or suspected coronary artery disease for more than two decades.
Thallium-201 is a cyclotron-produced monovalent cation with a physical half-life of 73.1 hours. It decays by electron capture to mercury-201. Thallium-201 is a low-energy gamma emitter with principal photopeaks at 135.3 keV (2.7% abundance) and 167.4 keV (10% abundance) and x-rays emitted from the mercury daughter at 68 to 80.3 keV (95% abundance). Some contaminants such as Tl-200 (<0.3%), Tl-202 (<1.2%), and Pb-202 (<0.2%) can be present, but that usually represents less than 2% of the total Tl-201 activity at the time of calibration. Contrary to the Tc-99m-labeled MPI agents, there is no in-house preparation and no quality control procedure required before injection in humans.
The myocardial transmicrovascular transport of Tl-201 was evaluated by Leppo and Meerdink6 in a blood-perfused, isolated rabbit heart model. The averaged myocardial extraction (Emax) of Tl-201 was 0.73 ± 0.10. The net myocardial extraction measured over a 2- to 5-minute period was 0.57 ± 0.13. The mean PS cap of Tl-201 was 1.30 ± 0.45 mL/g/min. Although Tl-201 is considered biologically similar to potassium, its myocardial uptake is greater than that of potassium. The myocardial extraction fraction of Tl-201 in in vivo model is approximately 87% at normal flow rates.7 Studies on the relationship of myocardial uptake of Tl-201 to regional coronary blood flow as determined by radiolabeled microspheres showed that there is a nearly linear correlation over a wide range of coronary blood flows (Fig. 3-1). However, at high flow rates (at approximately 2.5 mL/min/g), there is a diffusion limitation and a fall in tracer extraction fraction and also an increased cellular washout, resulting in a plateau in myocardial uptake. This results in an overall underestimation of higher levels of coronary blood flows. Conversely, at very low flow rates (usually less than 10% of the baseline blood flow), there is an increased myocardial extraction fraction of Tl-201 relative to blood flow, resulting in an overestimation of coronary blood flow.
Figure 3-1
Schematic representation of the relationship between myocardial blood flow levels and net myocardial radiotracer extraction for commonly used SPECT and PET myocardial perfusion imaging agents. The line of identity indicates the experimental gold standard for measuring blood flow, that is, trapped radiolabeled microspheres with a linear relationship between coronary blood flow and radiotracer uptake at various levels of blood flows.

One of the most clinically important characteristics of Tl-201 is its myocardial redistribution. Myocardial uptake of Tl-201 is not static over time. This property forms the basis of the stress-redistribution imaging protocol used to diagnose the presence of coronary artery disease with Tl-201. The redistribution or the filling in of a myocardial perfusion defect generally occurs between 3 and 5 hours after the injection of Tl-201 at peak stress and is related to two factors: the rate of influx of Tl-201 into the myocardium from whole-body blood pool activity and the rate of clearance or washout of Tl-201 from the myocardium. A myocardial perfusion defect related to ischemic disease will show a normalization of the Tl-201 uptake at 3 to 4 hours after the injection at stress because of a delayed accumulation into the ischemic segment and a more rapid washout from normal myocardial segments than from hypoperfused segments.
The greatest concentration of Tl-201 is found in kidneys, heart, and liver. The activity in these organs remains high for few hours after the injection. Maximal myocardial uptake, which is approximately 3.7% to 4.0% of the injected dose, is achieved by 10 to 15 minutes after the administration of the radiopharmaceutical. Tl-201 myocardial uptake has two components: an early component with a half-life of approximately 4 hours (80%) and a delayed component with a half-life of 40 hours (20%). Disappearance of Tl-201 from the blood compartment is rapid with two components: 92% of the blood activity disappears with a half-life of 5 minutes, and the other 8% will be cleared from the blood with a half-life of approximately 40 hours. This rapid clearance of Tl-201 from the blood results in a decreased blood pool background when MPI is performed. Table 3-2 summarizes the radiation dose estimates for Tl-201 along with the two Tc-99m-labeled MPI agents. Kidneys are the target organs.8 Based on the dosimetric data, the recommended adult dose of intravenous Tl-201 for SPECT MPI is 74 to 111 MBq (2–3 mCi), although several authors are using doses up to 4.0 mCi.
Tc-99m-Sestamibi | Tc-99m-Tetrofosmin | ||||
---|---|---|---|---|---|
Tl-201 Stress (Rads/3 mCi) | Rest | Stress | Rest | Stress | |
Adrenals | 0.7 | — | — | 0.5 | 0.5 |
Brain | 0.7 | — | — | 0.2 | 0.3 |
Breasts | 0.4 | 0.2 | 0.2 | 0.2 | 0.2 |
Gallbladder wall | 0.9 | 2.0 | 2.8 | 5.4 | 3.7 |
LLI | 1.60 | 3.9 | 3.3 | 2.5 | 1.7 |
ULI | 0.80 | 5.4 | 4.5 | 3.4 | 2.2 |
Small intestine | 0.20 | 3.0 | 2.4 | 1.9 | 1.3 |
Stomach | 0.3 | 0.6 | 0.5 | 0.5 | 0.5 |
Heart wall | 0.3 | 0.5 | 0.5 | 0.4 | 0.5 |
Kidneys | 5.1 | 2.0 | 1.7 | 1.4 | 1.2 |
Liver | 1.1 | 0.6 | 0.4 | 0.5 | 0.4 |
Lungs | 0.5 | 0.3 | 0.3 | 0.2 | 0.2 |
Muscles | 0.5 | — | — | 0.4 | 0.4 |
Ovaries | 1.1 | 1.5 | 1.2 | 1.1 | 0.9 |
Pancreas | 0.8 | — | — | 0.5 | 0.6 |
Red marrow | 0.6 | 0.5 | 0.5 | 0.4 | 0.5 |
Bone surface | 1.0 | 0.7 | 0.6 | 0.6 | 0.7 |
Spleen | 2.0 | — | — | 0.4 | 0.5 |
Testes | 0.9 | 0.3 | 0.3 | 0.3 | 0.4 |
Thymus | 0.5 | — | — | 0.3 | 0.3 |
Thyroid | 3.0 | 0.7 | 0.3 | 0.6 | 0.5 |
Bladder wall | 0.6 | 2.0 | 1.5 | 2.1 | 1.7 |
Uterus | 1.0 | — | — | 0.9 | 0.2 |
Total body | — | 0.5 | 0.4 | 0.4 | 0.4 |
Effective dose equivalent (rem/30 mCi) | 3.9 (rem/3 mCi) | 1.5 | 1.3 | 1.0 | 0.8 |
Thallium-201 was the major radiopharmaceutical in nuclear cardiology for almost two decades. Although hundreds of studies have demonstrated the clinical value of Tl-201 myocardial perfusion scintigraphy, the physical characteristics of this radionuclide are suboptimal for scintillation camera imaging. Therefore, in the late 1970s and early 1980s, many investigators attempted to develop an MPI agent labeled with Tc-99m to circumvent the physical limitations of Tl-201. The potential advantages of a Tc-99m-labeled agent over Tl-201 are significant and include the following:
The 140-keV photon energy of Tc-99m, which is optimal for standard gamma camera imaging, results in an improved resolution due to less Compton scatter and less tissue attenuation in the patient (in comparison to the low photon energy of 68–80 keV for Tl-201).
The much shorter physical half-life of Tc-99m (6 hours vs. 73 hours for Tl-201) and the better radiation dosimetry permit the administration of a 10 times higher dose of a Tc-99m-labeled compound than Tl-201. This yields better image quality, and images can be performed in a shorter time period.
The resulting overall better counting statistics of Tc-99m allows for the perfusion images to be optimally obtained in a gated mode. Simultaneous assessment of perfusion and function (global and regional wall motion) can thus be obtained.
It is possible to perform first-pass function studies (if the initial lung transit is rapid enough) with a Tc-99m-labeled radiopharmaceutical agent.
Because Tc-99m is constantly available from a molybdenum generator in a nuclear medicine laboratory, special deliveries from a distribution center or a commercial radiopharmacy are not required. A Tc-99m-labeled MPI agent can thus be available almost 24 hours a day.
For the above reasons, Tc-99m-labeled agents have enormous potential to assess myocardial perfusion and could be very useful clinically.9–11
The first member of the Tc-99m-isonitrile family to be evaluated in humans was the hexakis (t-butyl-isonitrile)-technetium (I), also known as Tc-99m-TBI.12,13 Although the myocardial uptake of Tc-99m-TBI was proportional to myocardial blood flow and was satisfactory for imaging purposes, its routine clinical use was limited by an increased lung uptake and prominent and persistent liver uptake. The initial lung uptake and subsequent washout of Tc-99m-TBI from the lungs also created significant imaging problems. A subsequently developed Tc-99m-isonitrile compound emerged from intensive search,14–16 initially known by the coded name of RP-30A (nonlyophilized form) or RP-30 (lyophilized form), and then as either Tc-99m-hexakis 2-methoxyisobutyl isonitrile, Tc-99m-hexakis-2-methoxy-2-methylpropyl-isonitrile, Tc-99m-hexamibi, or Tc-99m-MIBI, and currently by its generic name Tc-99m-sestamibi or Cardiolite™. Tc-99m-sestamibi has favorable biological characteristics for clinical applications with transient liver uptake and rapid hepatobiliary excretion, along with minimal lung uptake. Tc-99m-sestamibi was approved by the U.S. Food and Drug Administration for clinical application in December 1990.
Intravenous injections of Tc-99m-sestamibi have been associated with very few adverse reactions. According to the product monograph, during clinical trials (Phase III study) approximately 5% to 10% of patients experienced transient parosmia and/or taste perversion (metallic or bitter taste) occurring a few seconds after the injection. Usually, this side effect disappears within 15 to 30 seconds. This adverse effect seems to be related to the presence of the copper salt in the kit formulation, and its incidence may be related to the concentration of Tc-99m-sestamibi used.
Tc-99m-sestamibi is a cationic complex that is taken up by myocytes in proportion to regional myocardial blood flow. The cationic charge of the compound provides hydrophilic properties, and the six isonitrile groups allow hydrophobic interaction with cell membranes.
Tc-99m-sestamibi, which is retained within cells because of the negative charge generated on the mitochondria, has a high affinity for cytoplasm and shows very little extracellular exchange. Thus, metabolic derangements affecting myocytes’ viability would also result in decreased Tc-99m-sestamibi uptake, independently of myocardial blood flow. Using aerobic metabolic blockade, Beanlands et al.17 showed that an irreversible cellular injury resulted in a marked increase in Tc-99m-sestamibi clearance rate. They concluded that the accumulation and clearance kinetics of Tc-99m-sestamibi were dependent on sarcolemmal integrity and on aerobic metabolism and were significantly affected by cell viability.
Okada et al.18 investigated the myocardial kinetics of Tc-99m-sestamibi in dogs undergoing a partial occlusion of the left circumflex coronary artery. They showed that Tc-99m-sestamibi was rapidly taken up by nonischemic and ischemic myocardium at rest in proportion to regional myocardial blood flow. There was a good correlation between the initial myocardial flow at normal resting flow rates and the Tc-99m-sestamibi myocardial distribution. Another study from the same group of investigators19 using the same animal model evaluated the myocardial kinetics of Tc-99m-sestamibi after pharmacologic vasodilation with dipyridamole and showed that the initial myocardial uptake was closely related in a linear fashion to the regional myocardial blood flow at rates up to approximately 2.0 mL/min/g. However, at higher flow rates, there is a plateau in the myocardial distribution versus flow curve, resulting in an underestimation of coronary blood flow. Similar findings have been reported other investigators, which parallel the kinetics of thallium-201.20,21 There is overestimation of myocardial blood flow at low flows which is probably related to an increased extraction seen with diffusible indicators. Thus myocardial uptake of Tc-99m-sestamibi is proportional to regional myocardial blood flow over the physiological flow range with decreased extraction at hyperemic flows and increased extraction at low flows.
In contrast to Tl-201, Tc-99m-sestamibi shows a very slow myocardial clearance after its initial myocardial uptake. A fractional Tc-99m-sestamibi clearance of 10% to 15% over a period of 4 hours has been measured by Okada et al.18 in a canine model of partial coronary occlusion. The clearance was similar in the hypoperfused and normal zones. Animal studies have shown that after Tc-99m-sestamibi injection during brief periods (6–15 minutes) of coronary occlusion in dogs, the occluded zone shows a continued myocardial uptake during the reperfusion phase. Thus, following transient ischemia and reperfusion, there is some degree of myocardial redistribution of Tc-99m-sestamibi, although it is slower and less complete than Tl-201.22
Sinusas et al.23 studied the myocardial uptake of Tc-99m-sestamibi and Tl-201 showed that as long as myocardial cells were still viable, the myocardial uptake of the tracer was not affected by an ischemia. These data suggest that the agent can also assess myocardial viability. Tc-99m-sestamibi uptake is maintained in viable myocardium but reduced in necrotic tissue. Using a dog model with coronary occlusion and reperfusion, Verani et al.24 demonstrated that the size of the perfusion defect during occlusion as detected by scintigraphic images correlated with the amount of myocardium supplied by the occluded vessel, the area at risk. A smaller perfusion defect was detected on Tc-99m-sestamibi imaging during reperfusion. This defect correlated with the amount of infarcted myocardium and the area showing improved perfusion pattern after reflow represented the salvaged myocardium.
The blood clearance, biodistribution, dosimetry, and safety of Tc-99m-sestamibi were initially reported by Wackers et al.15 Both rest and stress blood clearance curves approximate a dual exponential curve with an initial fast and later slow component. The maximal activity at rest was noted at 1 minute after injection (36 ± 18% of injected dose), and the maximal activity after injection during exercise was measured at 0.5 minute. At 1 hour after the intravenous injection of Tc-99m-sestamibi, the blood pool activity progressively decreased to 1.10 ± 0.01% and 0.7 ± 0.1% of the injected dose at rest and after stress, respectively. At 60 minutes after the injection at rest, the uptake in the heart was 1.0 ± 0.4% of injected dose. The 24-hour urinary excretion was 29.5% of injected dose, whereas the 48-hour fecal excretion was 36.9% of injected dose. The study of the upper-body organ distribution showed that the highest initial Tc-99m-sestamibi concentration (counts/pixel) is found in the gallbladder and liver followed by heart, spleen, and lungs. The myocardial activity remained relatively stable over time (27 ± 4% of initial activity has cleared from the heart at 3 hours), whereas activity in the spleen and lung decreased gradually. The maximal accumulation in the gallbladder occurred approximately at 60 minutes after the injection. Similar biodistribution and radiation dosimetry was noted after exercise.
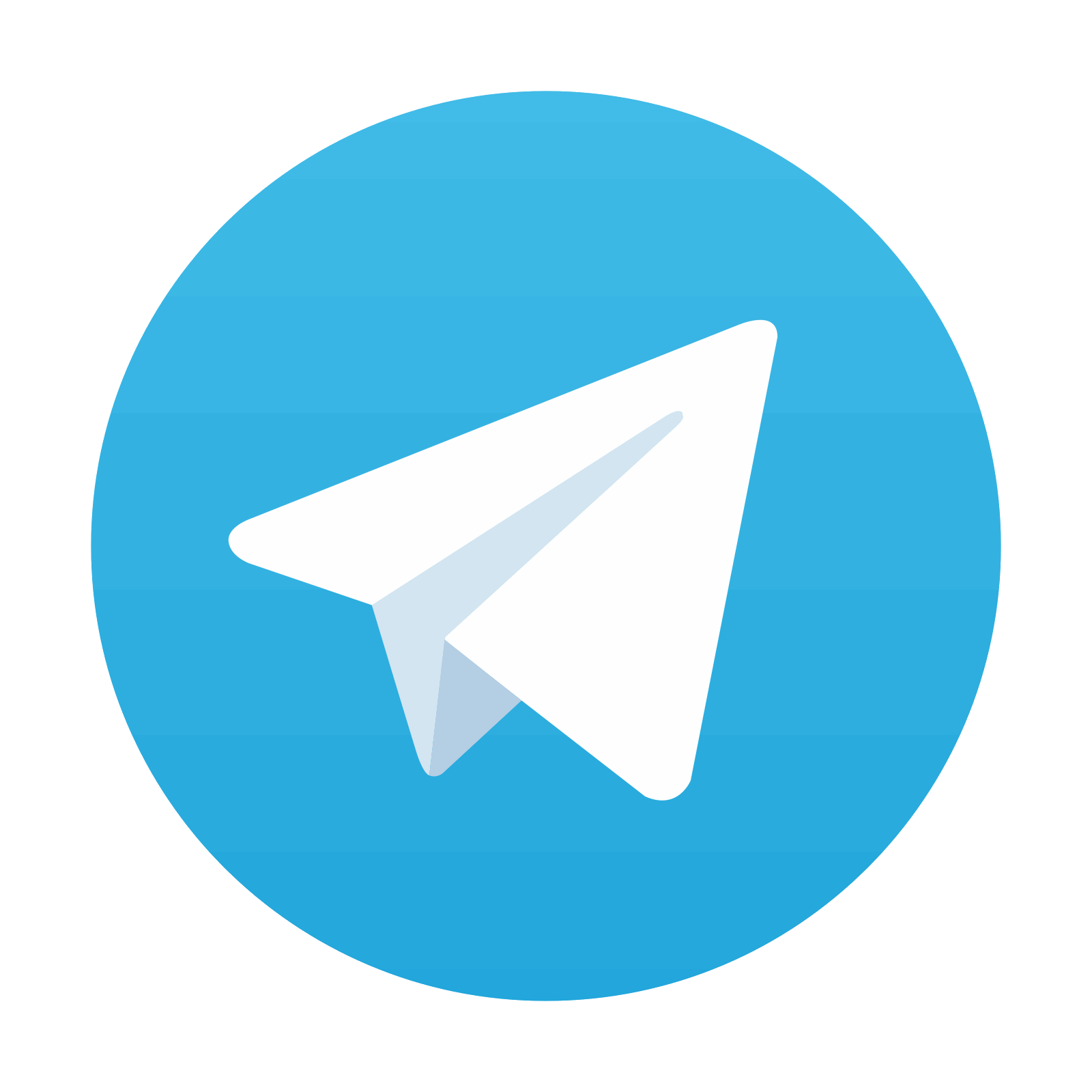
Stay updated, free articles. Join our Telegram channel

Full access? Get Clinical Tree
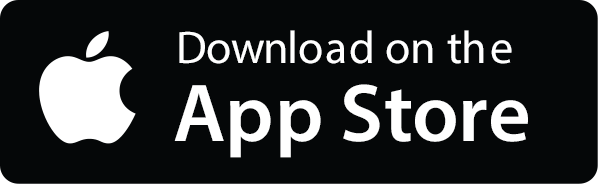
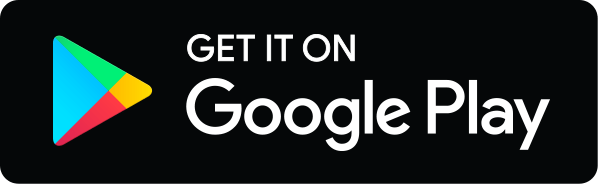