© Springer International Publishing Switzerland 2017
Gretchen G. Kimmick, Daniel J. Lenihan, Douglas B. Sawyer, Erica L. Mayer and Dawn L. Hershman (eds.)Cardio-Oncology10.1007/978-3-319-43096-6_77. Radiation Therapy and Cardiotoxicity
(1)
Department of Radiation Oncology, Duke University Medical Center, Morris Clinic Building, DUMC 3085, Research Drive, Durham, NC 27710, USA
(2)
Department of Radiation Oncology, Duke University Medical Center, 450 Research Drive, LSRC Building, Rm. B324, Durham, NC 27708, USA
(3)
Department of Cardiology, University of Texas MD Anderson Cancer Center, Houston, TX 77030, USA
Electronic supplementary material: The online version of this chapter (doi:10.1007/978-3-319-43096-6_7) contains supplementary material, which is available to authorized users.
Keywords
Radiation therapyCardiotoxicityRadiation-induced heart diseaseBreast cancerHodgkin lymphomaIntroduction
Radiotherapy is an integral part of achieving long-term cure in a number of intrathoracic malignancies. In this chapter the authors provide an overview of the epidemiology of heart disease after radiotherapy, with specific focus on data from Hodgkin lymphoma and breast cancer survivors. As clinical manifestation of heart disease can take years, if not decades, to manifest, animal models can assist in our understanding of the pathogenesis as well as the cellular and molecular mechanisms of radiation-induced heart disease, elucidating opportunities for intervention and prevention. Finally, recommendations for monitoring patients after radiotherapy and management of cardiac disease in patients with prior thoracic/breast neoplasms will be discussed.
Pathogenesis of Radiation-Induced Heart Disease in Experimental Animals
The pathological effects of ionizing radiation on the heart have been studied in different species of laboratory animals including mice, rats, rabbits, and dogs [1–8]. Sequential necropsies to evaluate histological changes to the heart were performed after either a single fraction (single dose of ≥15 Gy) or multiple fractions (cumulative dose of ≥36 Gy) of radiation to the thorax. Results from these animal studies demonstrate that focal irradiation results in time-dependent structural damage in various anatomical areas of the heart. Here, we will focus on animal studies that examine pathogenesis of radiation-associated cardiovascular problems commonly observed in the clinic, including pericarditis, cardiomyopathy, and coronary artery disease [9, 10].
Pericarditis
Acute pericarditis can develop in experimental animals within days to weeks after irradiation with 20–40 Gy due to inflammation [2, 3, 8]. Although acute inflammation may resolve with time, chronic changes have been observed over 20 months, which include a thickened pericardium with edema, inflammatory cells, fibroblast proliferation, and collagen deposition [5, 6]. The underlying mechanism of chronic pericarditis is not well understood, but it may be associated with increased inflammation secondary to microvascular injury [11].
Cardiomyopathy
Radiation-induced cardiomyopathy results from severe injury to the myocardium that subsequently impairs global function of the heart [9]. Animal studies reveal that radiation causes long-term tissue remodeling of the myocardium [1–3, 6]. For example, a large cohort study of radiation-induced heart disease (RIHD) using New Zealand white rabbits exposed to a single dose of 20 Gy showed progressive pericardial fibrosis, pericardial effusion , myocardial degeneration, and diffuse myocardial fibrosis 70 days after irradiation [1, 2]. Myocardial injury after irradiation is known to be associated with damage to the microvasculature, which is demonstrated by decreased microvessel density, focal loss of endothelial alkaline phosphatase, and increased expression of von Willebrand factor [1, 5]. In addition, infiltration of certain immune cells, such as mast cells, may also modulate the formation of myocardial degeneration and fibrosis [12]. The contribution of microvascular injury and mast cells to RIHD will be discussed in more detail later in this chapter.
Coronary Artery Disease
Epidemiological studies show that patients who received a mean radiation dose of 2 Gy to the heart have a significantly increased risk of developing ischemic heart disease more than 10 years after irradiation [10]. However, coronary artery disease associated with radiation exposure has not often been reported in animal studies of RIHD. This is likely because normal rodents are known to be relatively resistant to atherosclerosis as they have very low levels of low-density lipoprotein (LDL) in the plasma [10].
Several studies have used atherosclerosis-prone animal models to define the interaction of radiation with additional risk factors for cardiovascular disease, such as hypertension and hypercholesterolemia [13–16]. For example, Gabriels and colleagues studied RIHD in hypercholesterolemic and atherosclerosis-prone ApoE−/− mice, which received a single dose of focal heart irradiation up to 16 Gy. They found that radiation significantly increased the number of inflammatory cells, decreased microvascular density, and increased von Willebrand factor expression in the myocardium of the left ventricle. Most importantly, they found accelerated coronary atherosclerotic lesions that developed at 20 weeks after 16 Gy. Interestingly, despite these pronounced effects, cardiac gated SPECT and ultrasound measurements showed only minor changes in functional cardiac parameters at 20 weeks [15]. It is possible that surviving cardiomyocytes compensated for the radiation damage to the myocardium , and therefore these mice may slowly progress to develop cardiac dysfunction and heart failure with further follow-up .
Cellular and Molecular Mechanisms of Radiation-Induced Heart Disease
Endothelial Cells
Damage to vascular endothelial cells has generally been considered an underlying mechanism of RIHD [10, 17–19]. Radiation doses ≥2 Gy can substantially alter endothelial cell functions [10, 19]. After irradiation, endothelial cells significantly upregulate the expression of several cell adhesion molecules, including E-selectin , P-selectin , intercellular adhesion molecule-1 (ICAM-1) , PECAM-1 (CD31), and CD44 [10, 20]. Increased expression of these adhesion molecules promotes leukocyte adhesion and transmigration, which subsequently elicits a pro-inflammatory response [10].
In addition to causing functional alterations to endothelial cells, radiation also leads to cell death of endothelial cells in vivo as radiation decreases microvessel density and increases permeability of microvessels in the heart. Several studies have used primary endothelial cells isolated from different types of blood vessels to study endothelial cell death after irradiation in vitro. Results from these studies indicate that radiation can trigger various forms of endothelial cell death in vitro including apoptosis [21], mitotic catastrophe [22], and senescence [20, 22–24]. It is possible that the spectrum of endothelial cell death observed in vitro might be influenced by the origin of the endothelial cells (species, anatomic location, etc) and the dose of radiation . Given the diversity of gene expression profiles in human endothelial cells isolated from different tissues [25], how endothelial cells in the heart die from radiation in vivo remains to be fully understood.
An important question in the field is whether endothelial cell damage following radiation triggers cardiomyocyte injury or whether cardiomyocyte injury occurs independently of endothelial cell death. To dissect the role of endothelial cells in mediating radiation-induced injury to the heart in vivo, investigators can use genetically engineered mice [26]. For example, Lee and colleagues have used the site-specific recombinase system, Cre-loxP , to study the role of the tumor suppressor p53 specifically in endothelial cells in a mouse model of RIHD [11]. The tumor suppressor p53 is a transcription factor that serves as a master regulator of cellular response to radiation [27–29]. Upon radiation exposure, activation of the DNA damage response increases the level of p53 protein to induce a variety of downstream signaling pathways that mediate cellular response to stress [30, 31]. After whole-heart irradiation, mice in which both alleles of p53 were deleted specifically in endothelial cells were sensitized to radiation-induced myocardial injury and compared to mice that retained one allele of p53 in endothelial cells [11]. After whole-heart irradiation, mice with endothelial cells lacking p53 showed a focal decrease in microvessel density in the myocardium, which led to cardiac ischemia and myocardial necrosis [11]. The progression of myocardial necrosis resulted in systolic dysfunction and heart failure. Together, these results not only demonstrate a critical role for p53 in protecting cardiac endothelial cells from radiation in vivo but also provide compelling genetic evidence to show that damage to the myocardial vasculature leads to cardiac ischemia and myocardial necrosis, which can cause systolic dysfunction and heart failure [11].
Mast Cells
In rat models of RIHD , focal irradiation to the heart induces infiltration of mast cells [32, 33], which are tissue-resident sentinel cells that can both positively and negatively regulate immune responses [34]. It has been shown that infiltration of mast cells is associated with coronary atherosclerosis and myocardial fibrosis in animal models [35, 36]. Interestingly, experiments using mast cell-deficient rats reveal that mast cells play a protective role in RIHD in rats [12, 37]. After focal heart irradiation, mast cell-deficient rats showed more severe changes in cardiac function with increased deposition of collagen type III compared to their mast cell-competent littermates. These results suggest that mast cells may regulate cardiac dysfunction after irradiation by modulating collagen type III deposition.
It has been hypothesized that mast cells may protect rats against RIHD by activating the kallikrein-kinin pathway [37], as mast cell-derived proteases have been shown to elicit the release of kinins from their precursors, kininogens. To test this hypothesis, kininogen-deficient rats have been used to investigate the role of the kallikrein-kinin pathway in RIHD [38]. In contrast to results from studies using mast cell-deficient mice, changes in cardiac function were less severe in kininogen-deficient rats after local heart irradiation compared to their kininogen-intact littermates. Irradiated kininogen-deficient rats also showed a significant decrease in the number of CD68-positive macrophages but showed no difference in the number of mast cells in the heart compared to their kininogen-intact littermates. These results suggest that the cardioprotective effect of mast cells is not mediated by the kallikrein-kinin pathway. Mechanisms by which mast cells protect rats from RIHD remain to be better defined.
Future Directions in Preclinical Study
Accumulating data from animal models of radiation-induced heart disease have significantly advanced our knowledge of the pathogenesis of cardiac complications associated with focal radiation to the thorax or to the whole heart. However, the etiology of radiation-induced heart disease after partial heart irradiation, which is more clinically relevant to patients treated for breast cancer and lung cancer, remains largely unexplored. With the development of image-guided irradiators for small laboratory animals, it is now possible to more precisely deliver radiation to part of the heart. For example, in a proof-of-concept study, Lee and colleagues have developed a mouse model of radiation-induced myocardial injury after partial heart irradiation [39]. They utilized novel dual-energy microCT and 4D microCT with nanoparticle-based contrast agents to noninvasively assess the change in myocardial vascular permeability and cardiac function of mice after irradiation. This study revealed that animal models of RIHD after partial heart irradiation can serve as a platform for the development of biomarkers for noninvasive imaging , such as echocardiography and CT scans, to identify surrogates of cardiac injury that can be measured in cancer survivors before severe heart disease occurs. Such studies may shed light on the critical clinical question of the relative importance of mean radiation dose to the entire heart versus a high radiation dose to part of the heart in mediating RIHD. Ultimately, findings from animal models of RIHD have the potential to define new approaches for assessing and treating heart disease from radiation therapy and to increase the quality and quantity of life for cancer survivors.
Epidemiology of Heart Disease After Radiotherapy
Radiotherapy can affect all cardiac structures, resulting in myocardial infarction (MI) , valvular disease, pericardial disease, conduction abnormalities, and cardiomyopathy. While radiotherapy is associated with cardiac toxicity, various chemotherapeutic agents typically delivered sequentially may compound these effects on the heart. In addition patients with known cardiac comorbidities, such as hypertension, diabetes, smoking, and hyperlipidemia, are at higher risk of developing cardiac complications compared to patients with no known risk factors. The incidence of radiation-induced heart disease (RIHD) is best described from long-term clinical data in Hodgkin lymphoma and breast cancer survivors. Data suggest that CD and associated cardiac-related mortality does not manifest until nearly a decade posttreatment [40–42].
RIHD in Hodgkin Lymphoma Survivors
In Hodgkin lymphoma (HL) , cardiac complications appear to be associated with radiotherapy (RT) dose and radiation field. Historically radiotherapy has been widely used in the treatment of lymphoma since the 1960s after promising data from Stanford University. Total nodal field radiotherapy included a classic mantle (treatment of the supradiaphragmatic nodes including cervical, supraclavicular, and mediastinal/hilar regions) and inverted Y field (encompassing para-aortic, pelvic, and inguinofemoral lymph nodes) was the definitive curative approach prior to utilization of systemic therapy [43]. Over time, systemic therapy has become more effective, and as a result the radiotherapy dose has reduced. In addition, the size of radiotherapy volumes have decreased over time from extended field to involved field (radiation to involved anatomic nodal region) and more recently involved site radiotherapy [44–47].
Five-year survivors of HL treated prior to age 40 were found to have a higher relative risk (RR) of 3–5 for cardiac disease (CD) compared with the general population [48]. A report from the US Childhood Cancer Survivor Study included 2717 5-year HL survivors, and the RR of death from all cardiac causes compared with the general population was 11.9. The RR of death from MI secondary to mediastinal RT was estimated to be as high as 41.5 with historically higher radiation doses [49]. More recent studies of low-dose thoracic RT are associated with a lower incidence of CD [50–52]. A study of 1132 HL survivors who received treatment before age 18 between the years 1978–1995 evaluated CD incidence. Although the dose of doxorubicin 160 mg/m2 remained uniform during this time period, the mediastinal RT dose was 0, 20, 25, 30, or 36 Gray (Gy). A central, expert panel reviewed all reported cardiac abnormalities. CD was diagnosed in 50 patients, and valvular defects were reported most frequently, followed by CAD, cardiomyopathies, conduction disorders, and pericardial abnormalities. The 25-year cumulative incidence of CD was 21 % in the 36 Gy RT dose, decreasing to 10 %, 6 %, 5 %, and 3 % in the respective lower mediastinal RT dose cohorts (p < 0.001) [53].
Coinciding with decreasing RT dose, radiation fields have evolved from total nodal radiotherapy . With the use of combined modality therapy (chemotherapy and radiation), chemotherapy is paramount in eradicating subclinical disease. As such, there is now a shift toward involved field RT (IFRT) with randomized data demonstrating similar control rates with smaller treatment fields [46, 54, 55]. Ongoing studies are evaluating yet smaller treatment fields including involved site RT and involved nodal RT based on retrospective data suggesting equivalent outcomes compared to IFRT [56, 57]. As data emerge suggesting disease control with smaller RT fields, less heart in RT fields should result in a reduction of RIHD. This assertion has been corroborated by a series from Stanford in which 2232 HL patients from 1960 to 1991 were treated, and the associated RR for non-MI cardiac death fell from 5.3 to 1.4 once subcarinal blocking was introduced which decreased cardiac dose [50].
RIHD in Breast Cancer Survivors
Breast radiotherapy has evolved over time. Use of lower energy radiation, larger dose per fraction, and routine treatment of the internal mammary nodes were common practice in the 1970s and associated with increased risk of CD, but are no longer standard practice [40, 58]. In current treatment of breast cancer, the use of higher energy photons, CT-based planning to assess cardiac position, and techniques, such as prone positioning and breathing control (in breath hold the lungs insufflate and the heart is displaced away from the chest wall and inferiorly), help to minimize cardiac dose and thus decrease treatment-related toxicity. The long-term impact of these modifications on mitigating risk of CD is unknown as the associated cardiac-related mortality does not manifest until nearly a decade post-treatment. One key limitation of the data evaluating cardiovascular toxicity is that patients evaluated on these studies were typically treated decades ago with now antiquated techniques.
Long-term follow-up data from breast cancer randomized studies and large population databases demonstrate that RT can increase the risk of CD, specifically the risk of ischemic heart disease. A sample of over 4000 10-year breast cancer survivors treated in randomized studies prior to 1975 demonstrated no increased all-cause mortality rate; however, an increase in cardiac-related deaths was seen [58]. A large population-based case-control study of breast cancer patients in Denmark and Sweden assessed incidence of major coronary events in patients treated with radiotherapy between 1958 and 2001. Individual patient data were obtained from hospital records including the mean RT dose to the whole heart and left anterior descending (LAD) coronary artery. Major coronary events increased linearly with mean heart dose by 7.4 % per Gy [59].
Imaging tests have been utilized to objectively quantify RT effects on the heart. Single-photon emission computed tomography (SPECT) scans have been used to assess subclinical cardiac injury. A prospective study from Duke University assessed SPECT changes post-RT in women with left-sided breast cancer. SPECT abnormalities post-RT were visualized in 50 % of patients and were consistent with decreased perfusion in the volume of the irradiated left ventricle [60]. The clinical significance of these imaging findings, however, has not been fully elucidated. An analysis of a Swedish breast cancer cohort sought to determine the distribution of coronary artery stenosis and RT. Patients with a diagnosis of breast cancer were linked to registers of coronary angiography. The odds ratio of grade 3–5 stenosis (5 = complete occlusion) in the mid and distal LAD and distal diagonal for patients receiving left-sided radiotherapy was 4.38 compared to right-sided breast cancer treatment [61].
Although the aforementioned studies clearly suggest higher rates of cardiac disease associated with RT, additional data suggest that the incidence of RIHD has decreased over time with advances in RT planning and delivery. An analysis of patients treated at New York University calculated the excess absolute risk of radiation-induced coronary events as defined by Darby et al. with the use of more modern radiation techniques. For supine-positioned left breast RT, the cardiac dose was 2.17 Gray (Gy) and 1.03 Gy for prone positioning. Based on more modern data, the estimated lifetime risk of coronary events for patients receiving radiotherapy for breast cancer ranges from 0.05 to 3.5 % based on baseline risk (determined by total cholesterol, high-density lipoprotein, systolic blood pressure, and serum C-reactive protein) [62]. A Surveillance, Epidemiology, and End Results (SEER) analysis of patients treated between 1973 and 1989 estimated the risk of death from ischemic heart disease comparing women receiving left- to right-sided breast radiation. For women diagnosed between 1973 and 1979, there was a statistically significant 15-year mortality from ischemic heart disease of 13.1 % in left-sided patients compared to 10.2 % in right-sided. No differences between left- and right-sided cancers were seen in the cohorts of patients treated between 1980 and 1984 or 1985 and 1989 [40]. These data suggest that advances in radiation technique and delivery over time are responsible for the decline in late cardiac toxicities.
Advances in radiotherapy simulation and treatment planning have reduced the dose of radiation to the heart. In addition, radiation-associated CD is likely to be reduced by addressing concurrent cardiac risk factors through lifestyle modification and medication [62]. Understanding the pathophysiology of RIHD , particularly with the use of animal models may help clarify our understanding of this toxicity and create the opportunity for intervention and prevention.
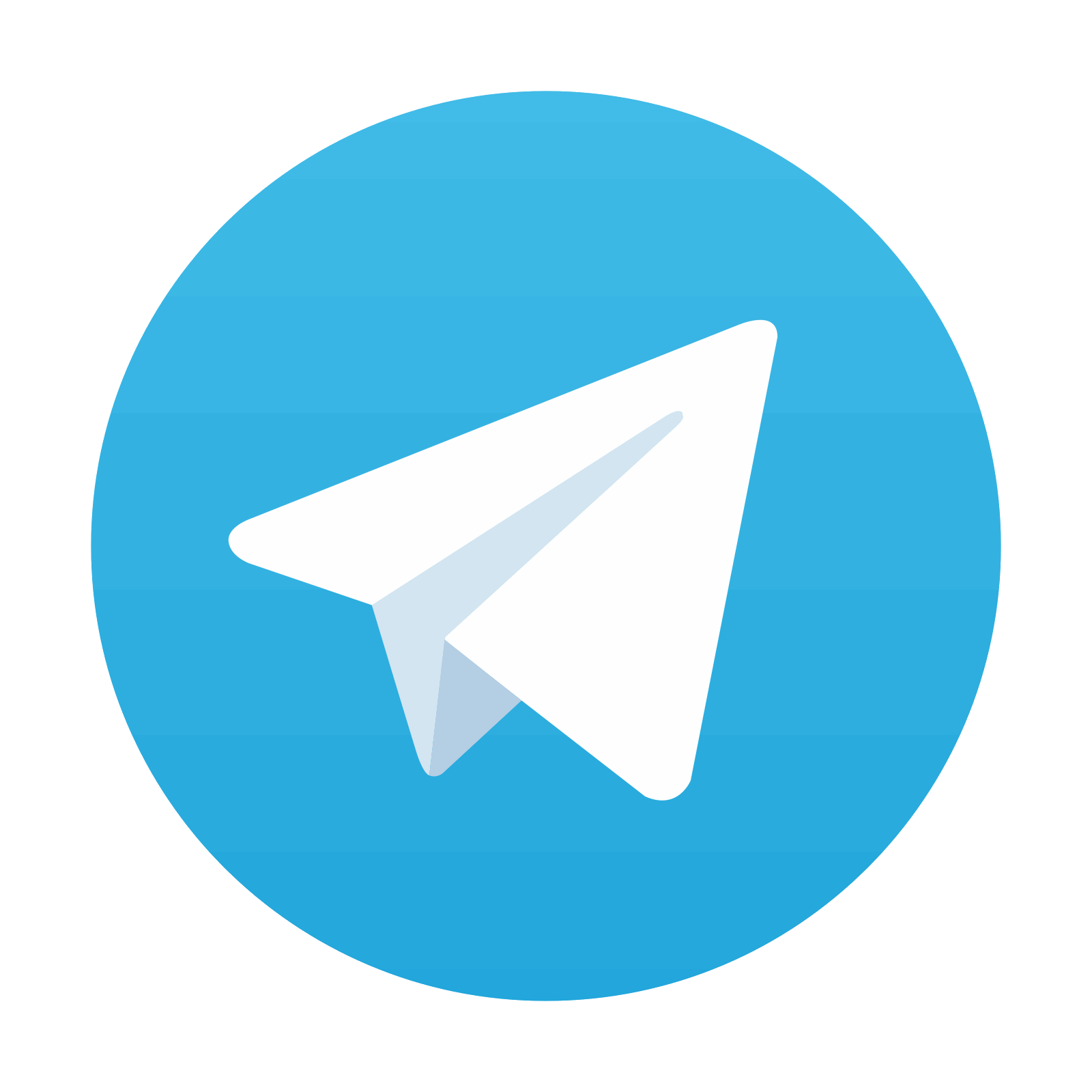
Stay updated, free articles. Join our Telegram channel

Full access? Get Clinical Tree
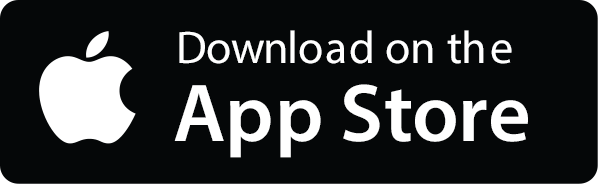
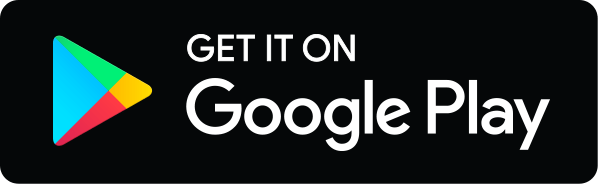