Attention ASE Members:
The ASE has gone green! Visit www.aseuniversity.org to earn free continuing medical education credit through an online activity related to this article. Certificates are available for immediate access upon successful completion of the activity. Nonmembers will need to join the ASE to access this great member benefit!
Types of Radiation and Basic Radiation Principles
Wilhelm Röntgen discovered x-rays in 1895 and created the first x-ray image (of his wife’s hand). The first practical and commercially available fluoroscope was developed by Thomas Edison in 1896. Other than skin burns, few adverse effects from ionizing radiation were understood until Edison’s assistant, Clarence Dally, succumbed in 1904 to the cumulative effects of repeated exposure. Since that time, the understanding of ionizing radiation has mandated its safe and effective use in medical imaging.
When x-rays pass through the human body to produce a radiographic image, the x-ray photons interact with human tissue in three ways: photoelectric absorption, coherent scattering, and Compton scattering. The photons that escape interaction travel on to the image receptor to produce the radiographic image. The principal interaction responsible for creating this image is photoelectric absorption. In photoelectric absorption, an x-ray photon transfers all its energy to an electron in an atom, and the photon disappears (it is totally absorbed). The electron is ejected from the atom and deposits its energy in the nearby tissue. In coherent scattering, an x-ray photon interacts with an entire atom, and changes its direction, but loses no energy in the process. When scattered photons reach the image receptor at random locations, they cause some image degradation by producing an overall haze in the image. Only a very trivial amount of coherent scatter exits the patient in a direction other than toward the image receptor, and it is not a significant concern for radiation exposure to those near the patient during the x-ray exposure. Compton scattering presents the greatest potential danger to a cardiac sonographer. This type of scatter is produced when an x-ray photon interacts with an electron in an atom and transfers some of its energy to the electron, ejecting it from the atom. The photon, however, retains most of its energy and may scatter in any direction. These scattered photons may exit the patient and become an exposure risk to those near the patient.
Types of Radiation and Basic Radiation Principles
Wilhelm Röntgen discovered x-rays in 1895 and created the first x-ray image (of his wife’s hand). The first practical and commercially available fluoroscope was developed by Thomas Edison in 1896. Other than skin burns, few adverse effects from ionizing radiation were understood until Edison’s assistant, Clarence Dally, succumbed in 1904 to the cumulative effects of repeated exposure. Since that time, the understanding of ionizing radiation has mandated its safe and effective use in medical imaging.
When x-rays pass through the human body to produce a radiographic image, the x-ray photons interact with human tissue in three ways: photoelectric absorption, coherent scattering, and Compton scattering. The photons that escape interaction travel on to the image receptor to produce the radiographic image. The principal interaction responsible for creating this image is photoelectric absorption. In photoelectric absorption, an x-ray photon transfers all its energy to an electron in an atom, and the photon disappears (it is totally absorbed). The electron is ejected from the atom and deposits its energy in the nearby tissue. In coherent scattering, an x-ray photon interacts with an entire atom, and changes its direction, but loses no energy in the process. When scattered photons reach the image receptor at random locations, they cause some image degradation by producing an overall haze in the image. Only a very trivial amount of coherent scatter exits the patient in a direction other than toward the image receptor, and it is not a significant concern for radiation exposure to those near the patient during the x-ray exposure. Compton scattering presents the greatest potential danger to a cardiac sonographer. This type of scatter is produced when an x-ray photon interacts with an electron in an atom and transfers some of its energy to the electron, ejecting it from the atom. The photon, however, retains most of its energy and may scatter in any direction. These scattered photons may exit the patient and become an exposure risk to those near the patient.
Sources of Radiation for Sonographers
Two sources of radiation exist for sonographers: patients and procedures. Sonographers sit very close to their patients and frequently drape their arms and bodies over patients who have recently received radioactive agents for diagnostic nuclear studies, thereby rendering the patients transiently radioactive. Proximity to this radioactive source and the relatively long duration of the exposure are two important determinants of potential radiation dose absorption by sonographers. This may be even more significant for novice and in-training sonographers, who may require more time to complete a study.
Over the past 10 years, there has also been a significant increase in demand for TEEFPs. Sonographers who assist with transesophageal echocardiography during transcatheter aortic valve replacement, percutaneous mitral valve repair, left atrial occluder device implantation, and atrial septal defect or patent foramen ovale device closure spend significant time in cardiac catheterization and electrophysiology laboratories and hybrid cardiac surgical suites in close proximity to x-ray sources while they are emitting radiation.
Chronic exposure to ionizing radiation is known to cause cataracts, leukemia, and several other types of cancer. Potential sources of radiation for a cardiac sonographer are participating in TEEFPs and performing TTE on patients who recently received ionizing radiation with agents that continue to emit radiation (“hot” patients). A basic understanding of personal protection techniques can greatly reduce radiation dose absorption by a cardiac sonographer from both the x-ray photons from x-ray units and gamma rays produced by the decay of radioisotopes given to patients as diagnostic tracers or part of the therapeutic regimen. Exposure to patient sources of radiation has been reported as being within what is considered acceptable limits for nurses and radiologic technologists, whereas prolonged close exposure of cardiac sonographers to the radioactive sources has not been adequately investigated.
It is imperative that sonographers be aware of their radiation exposure and take necessary steps to protect and monitor themselves. All sources of ionizing radiation in the clinical setting need to be acknowledged.
Personal Protection Techniques
Cardiac sonographers can significantly reduce radiation exposure from patients receiving radioisotopes and from patients undergoing TEEFPs by applying the cardinal principles of radiation safety: time, distance, and shielding ( Figure 1 ). Application of these principles incorporates methods for (1) decreasing exposure time, (2) increasing the distance from the radiation source, (3) increasing the time from isotope administration to the cardiac ultrasound procedure, and (4) using personal protective devices (PPD) and laboratory shielding ( Table 1 ). In this section we address each of these areas.
1. Decrease exposure time |
2. Increase time from isotope administration to cardiac ultrasound procedure |
3. Increase distance from the radiation source |
4. Use personal and laboratory shielding |
Decreasing Exposure Time
Sonographers traditionally have not been considered as being “exposed to radiation” while performing TTE on “hot” patients despite having close contact with the patients (the radiation source) for prolonged periods of time. Because the volume of patients receiving radioisotopes for nuclear scans shortly preceding the performance of TTE has increased, this exposure, along with the exposure during TEEFPs, merits consideration. Scheduling should include rotation of the cardiac sonography staff through these areas to minimize or dilute the risk for exposure. These patients and procedures should ideally be avoided by pregnant sonographers. However, if circumstances necessitate participation by a pregnant sonographer, she must strictly adhere to radiation safety regulations.
Increasing the Distance from the Radiation Source
Increasing the distance from the radiation source markedly reduces radiation exposure. Doubling the distance from a radiation source reduces the radiation exposure to one-fourth of the original dose. Therefore, small increases in distance very significantly reduce exposure. It is important to be mindful that the source of radiation is the patient, as a radioactive source if the patient has recently received a radioisotope or as a source of x-ray scatter if the patient is undergoing a TEEFP. In the latter case, radiation to the sonographer directly from the x-ray tube should be negligible. However, close proximity to the patient along with table and x-ray tube positioning and associated spatial limitations to the optimal use of shielding during TEEFPs potentially place the TEE operator and sonographer at increased risk for significant radiation exposure. It is important in positioning oneself and shielding to realize that scattered radiation intensity is greatest on the x-ray beam entrance side of the patient. Optimal operator and sonographer positioning to reduce radiation exposure is ideally on the side opposite to the x-ray tube. Positioning should be considered in advance of the procedure.
Exposure can be calculated by the inverse square law: I 1 / I 2 = ( D 2 / D 1 ) 2 , where I denotes the intensity of the beam and D denotes the distance from the source, wherein the x-ray beam intensity is inversely proportional to the distance squared. Therefore, and worthy of emphasis, small increases in distance from the radiation source substantially reduce radiation exposure. This principle applies to patients who have been injected with radioisotopes, those undergoing TEEFP, and brachytherapy (internal radiotherapy) patients.
Increasing the Time between Isotope Administration and the Ultrasound Procedure
Performing TTE on a patient before the administration of a radioisotope or delaying TTE in a patient who recently received a radioisotope can eliminate and reduce radiation exposure, respectively. Delaying echocardiography in a patient who has very recently received a radioisotope for nuclear medicine imaging will enable some radioactive decay, thereby reducing the potential dose to the sonographer. Therefore, as is possible, an ultrasound examination of a patient who has very recently received a radioisotope for nuclear medicine imaging should be delayed. Technetium-99m is used for the vast majority of cardiac nuclear studies ( Table 2 ). The far less frequently used isotope 201 Tl, although having a much longer half-life compared with 99m Tc, has a lower exposure rate constant and thereby less potential dose to the sonographer (by at least an order of magnitude).
Isotope (radiopharmaceutical) | Physical half-life | Procedure |
---|---|---|
99m Tc-labeled agent (e.g., 99m Tc-sestamibi) | 6 h | SPECT MPI (for myocardial perfusion, function, viability) |
201 Tl | 73 h | SPECT MPI (for myocardial perfusion, viability, function) |
99m Tc-pertechnetate | 6 h | Radionuclide ventriculography, also referred to as a MUGA scan (for myocardial function) |
18 F-FDG | 110 min | PET (for myocardial metabolism, viability, inflammation) |
82 Rb | 75 sec | PET (for myocardial perfusion, blood flow, function) |
Ammonia 13 N | 10 min | PET (for myocardial perfusion, blood flow, function) |
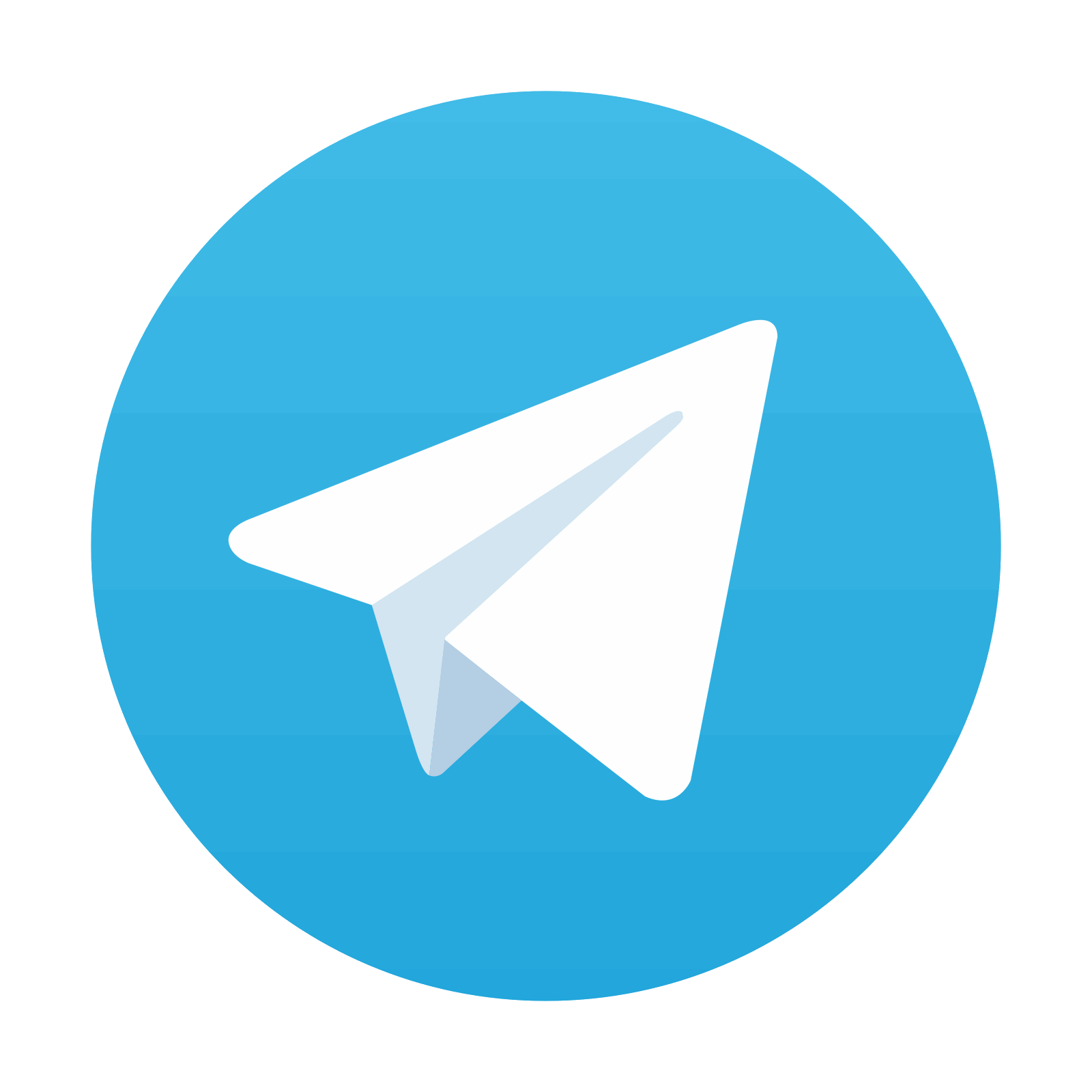
Stay updated, free articles. Join our Telegram channel

Full access? Get Clinical Tree
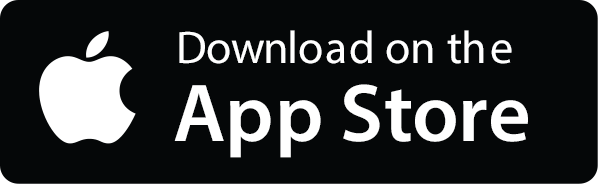
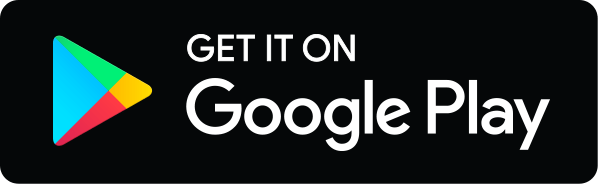
