Measurement of blood flow to the myocardium represents a quantum leap forward for the field of nuclear cardiology. Quantification of myocardial blood flow as a routine part of rest/stress myocardial perfusion imaging is a unique attribute of cardiac PET. Although the quantification is actually measured in mL/min/g of myocardium, it is usually expressed as a ratio between peak hyperemia and rest. This ratio is termed myocardial blood flow reserve (MBFR) or coronary flow reserve (CFR). In this chapter we use the term MBFR to be in agreement with a recently published joint position paper of the American Society of Nuclear Cardiology (ASNC) and the Society of Nuclear Medicine and Molecular Imaging (SNMMI).1 MBFR can be measured on a pixel-by-pixel basis if there are sufficient counts, but for clinical applications MBFR is reported globally (an average for the entire myocardium), segmentally, or by coronary territory. Some patients have high resting flows that can artifactually lower MBFR; therefore, it is important to also consider peak hyperemic myocardial blood flow (MBF) in some cases, especially in patients with low MBFR and high resting MBF. There has not been a consensus as to whether peak hyperemic MBF or MBFR is superior for assessing epicardial and microvascular health.2,3 A recent study examined cardiovascular mortality in more than 4000 consecutive patients, and found that MBFR was a stronger predictor of outcomes than peak flow.4 In keeping with many studies in the literature, in this chapter we focus primarily on MBFR.
Why is myocardial perfusion PET and the ability to quantify MBFR such an important advance for nuclear cardiology? One of the important attributes of SPECT myocardial perfusion imaging is its ability to risk-stratify populations based on the size and severity of perfusion defects.5,6 Those whose findings suggest moderate to high risk are often referred to coronary angiography with the expectation of revascularization.7 Unfortunately, clinicians and interventionists have recognized the shortfalls of traditional spatially relative interpretations of myocardial perfusion studies. When in the catheterization laboratory with an individual patient, decisions need to be made when the coronary angiography appearances deviate from those anticipated from the MPI reports. Publications from the nuclear cardiology community acknowledge the deficiencies of spatially relative interpretation, in which image appearances may suggest limited CAD in patients found to have multivessel CAD at angiography.8,9 Other publications emphasize the false-positive potential of MPI due primarily to attenuation artifacts.10,11 Hence, the issues with strong population-based evidence and yet management challenges with application of this population-based evidence to a significant number of individual patients. Cardiac PET MPI has several attributes that can address these problems. First, every study is attenuation corrected. Second, with 82Rb, the gated stress images are acquired under peak hyperemia conditions, such that regional changes in wall thickening or motion indicate ischemia and hence highly narrowed coronaries. Under conditions of increased myocardial blood flow, global increases in function such as LVEF are anticipated; drops in LVEF correlate with extensive CAD independent of the spatially relative MPI appearances.12,13 Finally, PET studies permit a segment-centric as opposed to a spatially relative assessment of changes in myocardial blood flow, by virtue of its ability to quantify changes in blood flow between resting and peak hyperemic conditions. Numerous studies have shown the independent and incremental value of this information for both diagnosis and prognosis after consideration of all other clinical and imaging data.14–24 This chapter examines many aspects of myocardial blood flow with cardiac PET, including acquisition of flow data, patient selection, evaluation of validity of flow data, assessing the importance of the findings, and reporting of the findings.
Most commonly a patient is positioned in the PET camera, and vital signs and a rest ECG are acquired, followed by an infusion of 82Rb, the most common PET tracer used in the United States. Acquisition begins at the start of the 82Rb infusion, so that the infusion can be tracked as it travels through the heart chambers. Then the patient is “stressed” with an arteriolar vasodilator, such as dipyridamole, adenosine, or regadenoson, or a beta-adrenergic agonist, such as dobutamine, followed by the 82Rb infusion with the same acquisition sequence. After completion of image acquisition, the data are transferred to work stations for processing. This involves two different operations: one for preparation of the images, and one for measurement of blood flow. Prior chapters have described the technical details of these steps.
If the PET tracer used is NH3-ammonia, the rest study is completed and then this longer-lived tracer (9.8 minutes vs 75 seconds for 82Rb) is given time to decay prior to starting the stress component of the test. Therefore, usually the patient is removed from the camera after the rest acquisition and returns later for the stress study. Otherwise, the study is similar to a 82Rb PET MPI.
Regardless of tracer used, it is important to appreciate that acquiring the data to permit flow quantification can be done routinely as part of a rest/stress PET MPI study. It does not prolong image acquisition and does not expose the patient to any additional radiation. What it contributes is a full interrogation of the integrity of the delivery system to the myocardium, including the epicardial coronary arteries and the microvasculature, and adequacy of the stressor agent.
From the clinical perspective, there are several important considerations when the interpreter is presented with the MBF measurements. These include an assessment of whether the measurements would have any useful clinical value for a particular patient, an evaluation for quality and face-validity, a conceptual framework for understanding the meaning of the measurements, and finally a useful way of reporting the information as a guide to next steps in patient management.
Many labs acquire flow data as part of the routine performance of a rest/stress myocardial perfusion PET study. However, there are several circumstances where the flow data is likely to be inaccurate. Some examples include patients with moderate to severe valvular regurgitation or large shunts, such as with VSDs or ASDs, and patients who cannot lie still such that precise registration for attenuation correction is not possible. Recent guidelines also caution against the routine reporting of flow values in cases where the information may not add diagnostic value and could be misleading to a referring physician.25 The guidelines specifically mention patients with severe cardiomyopathies, large prior infarctions, advanced kidney disease, and those post-CABG. These are all examples where flows will usually be abnormal and in patients already known to be high-risk (Table 5-1). Obviously, some of these patients would benefit from reporting of flows, especially if there was found to be no flow augmentation (suggesting the likelihood that the vasodilator stress was ineffective). However, interpreters should always carefully consider the individual patient before incorporating a flow value because the flow information can sometimes lead to confusion or wrongful patient management.
|
Most other patients will have potential benefit of combining the image appearances with flow quantification. The guidelines specifically focus on patients with no known CAD but with symptoms suggestive of CAD, patients with known CAD in whom more definitive information about physiologic significance is desired, posttransplant patients, those in whom multivessel CAD is a concern, and in symptomatic patients with normal coronaries when microvascular disease is a possibility (Table 5-2).
|
A unique, relatively small but important group of patients are those who have no change in MBF in response to vasodilator stress.25 Patients whose stress MBF is not changed from baseline may not have responded to the vasodilator applied. In essence, then, there was no real stress. This may reflect the inhibiting effects of caffeine or excess adrenergic tone. Only the flow measurements will be helpful in these situations, because changes in neither blood pressure nor heart rate carry certainty about changes in MBF.26 The mechanisms that augment myocardial blood flow are different from those that affect symptoms, heart rate, or blood pressure. Patients who have no augmentation in MBF with a vasodilator need consideration for either repeat vasodilator testing after a confirmed 24-72–hour abstinence from caffeine or testing with dobutamine. This situation arises most frequently with emergency department patients who have arrived unprepped for an MPI; it is this same group of patients who are at high risk for acute myocardial infarctions if discharged on the basis of a normal-appearing MPI but who in reality did not have a valid rest/stress MPI. Figure 5-1 shows such an example. One of the major benefits of flow quantification is assuredness that the vasodilator actually “worked.” It is essential that the report include language about the test being nondiagnostic when there is no difference in flows at rest and following injection of the vasodilator.
Figure 5-1
37-Year-Old Male Presenting to the Emergency Department. He had 20 minutes of chest pain, relieved en route with2 SL NTG. Serum TnI was normal. The scan was normal. However, the CACS was 250 and the MBFR was only 1.1. The patient was dismissed from the ED but returned 24 hours later with a STEMI caused by an occluded right coronary artery. This is an example of a non-stressed MPI. The essentially absent MBFR was the only indicator that the vasodilator stress was ineffective.

The reading physician needs some guidance in order to be confident in the robustness of the MBF measurement. Just as with other forms of cardiac imaging, there needs to be careful attention to detail or the flow measurements can be erroneous. The reading physician expects cases where the flow data will be concordant with other clinical information, such as perfusion appearances, regional and global function at rest and at peak stress, and clinical parameters. However, flow information is often the only clue warranting a change or modification in diagnosis and prognosis. For example, abnormal flow with normal perfusion and function nonetheless places a patient at higher risk for events20 and in many cases will identify otherwise unsuspected multivessel CAD.24 Most patients with globally low-flow reserve will need further testing with either coronary CTA or invasive coronary angiography to rule in or out multivessel CAD. Multivessel CAD will be present in less than 50% according to one study,24 while moderate diffuse CAD with or without microvascular disease is also a common cause (Figure 5-2). On the contrary, globally normal flow in a patient with a perfusion defect can serve to rule out multivessel CAD23 and establish a relatively low likelihood for mortality.20,22 Numerous studies have shown the independent and incremental information about both the diagnosis and prognosis of patients based on flow measurements acquired as part of PET MPI, independent of whether the images appear normal or contain perfusion defects.14–24
Figure 5-2
While probability of multivessel CAD increases as MBFR decreases, there are other causes of reduced MBFR so that down-stream testing is positive for multivessel CAD in <50% of patients. (Reproduced with permission from Ziadi MC, Williams K, Guo A, et al: Does quantification of myocardial flow reserve using rubidium-82 positron emission tomography facilitate detection of multivessel coronary artery disease? J Nucl Cardiol. 2012 Aug;19(4):670-680)

There are a number of common factors that can easily lead to an erroneous flow measurement. Some of these include beginning the acquisition too late; interruption of the radionuclide travel by line kinking, anatomic causes of slowing, or extravasation; scanner count saturation; patient motion; improper placement of the blood pool region of interest; or poor delineation of the myocardial uptake. These can be investigated by the interpreting physician by careful inspection of the blood pool and myocardial uptake curves, and visual inspection of the blood pool region of interest and the myocardial uptake contours. Figures 5-3, 5-4, 5-5, 5-6 show some examples of adequate and inadequate time-activity curves, correct and incorrect blood pool regions of interest, and proper identification of the myocardium. Further information is available from several other sources.1,27–32 This topic is also discussed in Chapter 4.
Figure 5-3
Example of Good Rest and Stress Time-Activity Curves. Note that the acquisition started prior to the tracer arriving at the blood pool region of interest. The rate of rise of the time-activity curves is relatively steep and the curves are relatively narrow. The stress peak is usually lower than the rest peak. The downward portion of the curves is smooth. Given sufficient time, the curves will approach zero.

Figure 5-4
Example of major technical problems identified by inspection of time-activity input and myocardial curves. Note that the flow measurements by themselves provide no clues that there has been a technical problem likely making the flow measurements highly erroneous. Only by inspecting the curves would an interpreting physician be aware that the flow measurements are likely inaccurate. In this case, the likely problem is a combination of kinking of the intravenous line as well as patient motion.

Figures 5-5
Input Regions of Interest for MBF Program Utilized Were Intended to Be in the Left. Atrium Note that the placement in (A) is different at stress compared to rest. Note also the global MBFR measurement for (A) showed under the image and compare this with the measurement shown in (B), when the regions of interest are properly positioned.
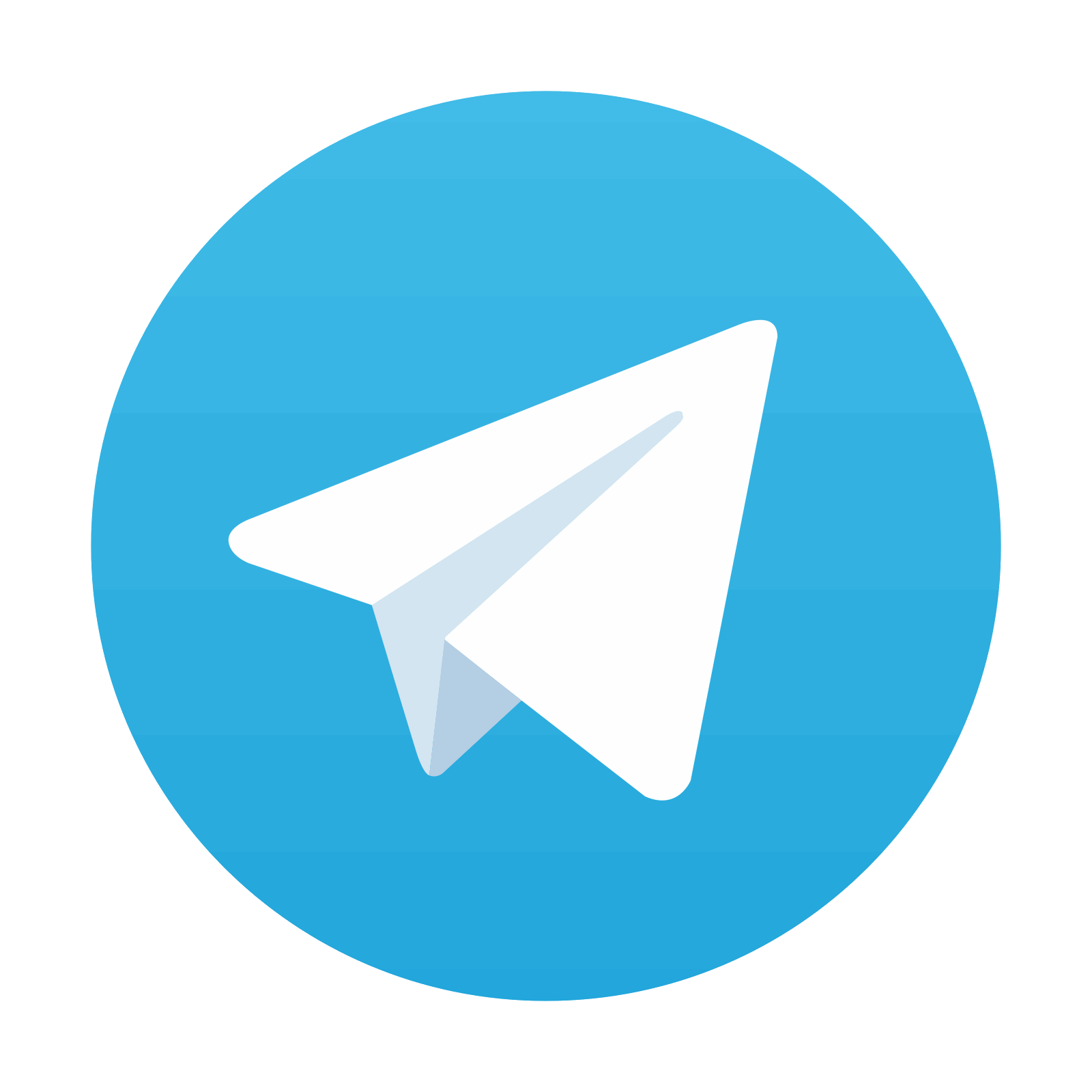
Stay updated, free articles. Join our Telegram channel

Full access? Get Clinical Tree
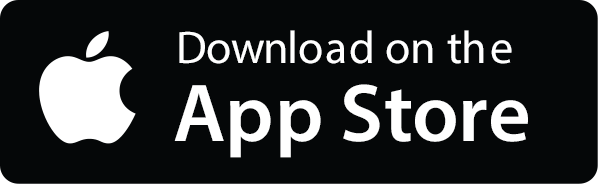
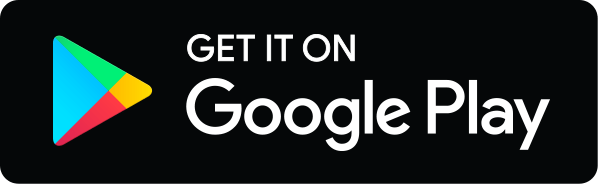
