Background
Many echocardiographic features of constrictive pericarditis (CP) have been reported, but each alone has a limitation either in sensitivity or in specificity. Continuous-wave Doppler–derived flow velocity of pulmonary regurgitation can reflect the diastolic right ventricular pressure pattern characteristic of CP and be useful for its detection.
Methods
Fifteen patients with CP, 18 patients with restrictive cardiomyopathy, and 20 normal subjects were studied retrospectively. Using continuous-wave Doppler echocardiography, pulmonary regurgitation velocities were measured at the early diastolic peak (V MAx ), mid-diastolic inflection point (V IFL ), and late diastolic minimal point (V MIN ).
Results
V IFL , V IFL /V MAx , V MIN , and V MIN /V MAx were significantly lower in the CP group compared with the restrictive cardiomyopathy and normal groups. Prevalence rates of patients with early mid-diastolic inflection, V IFL /V MAx < 0.5, V MIN < 50 cm/sec, and V MIN /V MAx < 0.33 were significantly greater in the CP group compared with the other groups. Sensitivity and specificity for the diagnosis of CP were 93% and 74%, respectively, for the presence of early mid-diastolic inflection, 73% and 100% for V IFL /V MAx < 0.5, 73% and 97% for V MIN < 50 cm/sec, and 93% and 92% for V MIN /V MAx < 0.33.
Conclusions
The quantitative and pattern analyses of continuous-wave Doppler–derived pulmonary regurgitation velocity could enhance the accuracy of echocardiographic detection of CP.
Echocardiography has become a mainstay for the diagnosis of constrictive pericarditis (CP) in modern clinical practice. However, it is not easy to detect CP in a routine echocardiographic examination. Representative echocardiographic findings of CP include pericardial thickening, dilation of the inferior vena cava, early diastolic notch formation of interventricular septal motion, diastolic plateau observed in the left ventricular (LV) posterior wall motion, inspiratory movement of the interventricular septum toward the left ventricle, an increase in respiratory variation of early diastolic transmitral flow velocity, and an increase in respiratory variation of early diastolic transtricuspid flow velocity.
These classic qualitative and quantitative parameters are usually used in combination, because each alone has a limitation either in sensitivity or in specificity. Pericardial thickening and inferior vena cava dilatation are sensitive findings for CP, but they are entirely lacking in specificity. Other parameters, relatively specific, are not as sensitive. In addition, M-mode echocardiographic findings, such as early diastolic notch of the interventricular septum, diastolic flattening of the LV posterior wall, and inspiratory movement of interventricular septum, can be overlooked because M-mode examinations are now frequently omitted. Pulsed-wave Doppler measurements of respiratory variation of transmitral and transtricuspid flow velocities require the observation and recording of multiple cardiac cycles and can also be overlooked during busy routine echocardiographic examinations. Thus, it would be valuable to obtain a new index of CP with better diagnostic power but without adding to the burden of the routine echocardiographic examination.
Flow velocities of pulmonary regurgitation (PR) measured using continuous-wave Doppler echocardiography has been shown to be useful for the noninvasive estimation of the mean and end-diastolic pulmonary artery pressure. Recently, Gilman et al . reported that the cessation of PR velocity at mid-diastole was often observed in patients with CP. This valuable pilot study suggested the usefulness of PR flow observation for the diagnosis of CP; however, the investigators used a qualitative approach only, and flow abnormality was not strictly defined. We thus consider that further study is clearly needed to establish the usefulness of an abnormal PR velocity pattern in the diagnosis of CP. In this study, we performed quantitative analysis of continuous-wave Doppler–derived PR velocity to obtain useful criteria for CP that can be easily found even during a busy routine echocardiographic examination.
Methods
Subjects
Among 22,774 patients who underwent echocardiographic examinations between June 2005 and January 2013 in our laboratory, 34 were diagnosed with CP on the basis of echocardiographic findings. Among them, we excluded 12 patients with atrial fibrillation, one with severe tricuspid regurgitation (vena contracta width > 7 mm) and two with inadequate PR waveforms. Of the remaining 19 patients, 15 who met the following inclusion criteria were studied retrospectively: (1) pericardial thickening with maximal pericardial thickness ≥ 4 mm on a computed tomographic image ; (2) hemodynamic abnormalities characteristic of CP, which were (2a) a dip-and-plateau pattern of LV or right ventricular (RV) pressure or equalization of biventricular end-diastolic pressure (<5 mm Hg) by cardiac catheterization or (2b) the presence of three of six relatively specific echocardiographic findings of CP, such as early diastolic notch of the interventricular septum, diastolic flattening of the LV posterior wall, movement of the interventricular septum toward the LV side during inspiration, increase in respiratory variation of pulsed-wave Doppler–derived transmitral flow (≥25%), that of transtricuspid flow (≥40%), and annulus reversus of tissue Doppler mitral annular velocities ; and (3) the presence of right heart failure, that is, at least four of six clinical and imaging findings including edema, hepatomegaly, pleural effusion, ascites, inferior vena cava dimension > 21 mm, and long-term use of loop diuretics.
Patients were included in the CP group only when all three criteria were satisfied. For criterion 2, seven patients fulfilled condition 2a; dip-and-plateau patterns of LV or RV pressure were seen in six and equalization of biventricular end-diastolic pressure in five. The remaining eight patients fulfilled condition 2b; ventricular septal notch was present in eight, LV posterior wall flattening in six, respiration-related ventricular septal shift in eight, respiratory variation in mitral flow in five, respiratory variation in tricuspid flow in four, and annulus reversus in three. Patients with CP included 12 men and three women ranging in age from 28 to 82 years (mean, 63 ± 18 years). Etiologies of CP included postpericardiotomy in six patients, viral or bacterial infections in two, post–radiation therapy in two, trauma in one, and undefined in four. New York Heart Association functional class was III in six patients, II in eight patients, and I in one patient.
Twenty normal subjects and 18 patients with restrictive cardiomyopathy (RCM) were also studied. The normal group consisted of patients who had undergone echocardiographic examinations to rule out cardiac diseases and were judged not to have any structural cardiac abnormalities by expert cardiologists. They included 12 men and eight women ranging in age from 31 to 82 years (mean, 59 ± 15 years). The RCM group included patients who were diagnosed as having myocardial disease with transmitral early to late diastolic peak velocity ratios > 1.5, deceleration times of early diastolic velocity < 160 msec, isovolumic relaxation times < 70 msec, transmitral atrial systolic flow durations shorter than the duration of pulmonary vein atrial flow reversal, and LV ejection fractions > 50%. Patients with pericardial thickening (≥4 mm) on computed tomographic images were excluded. This group consisted of five patients with cardiac amyloidosis, two with eosinophilic heart disease, two with mitochondrial cardiomyopathy, one with connective tissue disease, one with Fabry’s disease, and seven with undetermined etiologies. They included nine men and nine women, ranging in age from 24 to 79 years (mean, 56 ± 17 years). New York Heart Association functional class was III in seven patients and II in 11 patients.
This study was approved by the Research Ethics Committee of Hokkaido University Hospital as a retrospective observational study.
Echocardiographic Examination
Echocardiography was performed using an Aplio XG/Artida equipped with a 2.5/3.0 MHz probe (Toshiba Medical Systems, Otawara, Japan), a Vivid7/E9 with an M3S/M4S probe (GE Healthcare, Little Chalfont, United Kingdom), or a Sonos 5500 with an S3/S4 probe (Philips Medical Systems, Eindhoven, The Netherlands).
Using two-dimensional echocardiography, the LV end-diastolic dimension, left atrial end-systolic dimension, and inferior vena cava dimension were measured. Following the guidelines of the American Society of Echocardiography, LV volume (milliliters) and ejection fraction (percentage) were measured using the biplane method of disks, and LV mass index (grams per square meter) was calculated from two-dimensional linear measurements of LV end-diastolic dimension, interventricular septal thickness and LV posterior wall thickness. Left atrial volume (milliliters) was also measured using the biplane area-length method from apical four- and two-chamber images.
Apical pulsed-wave Doppler echocardiography was performed to measure peak early diastolic and late diastolic transmitral flow velocities (E and A, respectively; centimeters per second), and the ratio of early to late peak velocities (E/A) was calculated. Deceleration time of the E wave (milliseconds) was also measured. LV isovolumic relaxation time (milliseconds) was measured from the apical continuous-wave Doppler recording, which clearly depicted both LV inflow and outflow. The early diastolic mitral annular velocities at the interventricular septal side (septal e′; centimeters per second) and at the lateral side (lateral e′; centimeters per second) were measured from the apical tissue pulsed-Doppler recording. Using continuous-wave Doppler echocardiography the peak tricuspid regurgitant velocity was measured to calculate peak systolic gradient between the right ventricle and right atrium (millimeters of mercury).
Continuous-wave Doppler was also used to obtain the flow velocity recording of PR during a breath-hold at shallow expiration or in a cardiac cycle at the intermediate expiratory position under quiet respiration ( Figure 1 ). To minimize the incident angle of the beam to the PR jet, the color flow signal of the PR jet was visualized in two mutually orthogonal planes from several different left parasternal echocardiographic windows, and the PR flow velocity was recorded from the echocardiographic window providing the least incident angle. The peak early-diastolic velocity (V MAx ; centimeters per second) and deceleration time after the peak (milliseconds) were measured. We assessed the presence or absence of an early mid-diastolic inflection just after the sharp early diastolic descent and, when present, the velocity at the inflection point (V IFL ; centimeters per second) was measured. In addition, we measured the minimal velocity from the early-diastolic peak to end-diastole (V MIN ; centimeters per second), which usually corresponded to the bottom of the atrial systolic dip or the end-diastolic angle of the PR velocity envelope.

Statistical Analysis
Statistical analyses were performed using standard statistical software (SPSS II for Windows; SPSS, Chicago, IL). All numeric data are presented as a mean ± SD. Differences among the three groups were tested by using Scheffé’s method when there was a significant difference among groups by one-way analysis of variance. Receiver operating characteristic curve analysis was used to evaluate the accuracy of various echocardiographic parameters for diagnosing CP. The χ 2 test was used to compare the frequencies of abnormal flow patterns. For all statistical tests, P values < .05 were considered statistically significant.
Results
Basic Echocardiographic Features
The basic echocardiographic features of the study subjects are presented in Table 1 . No significant differences were observed in age, heart rate, LV end-diastolic dimension, and LV end-diastolic volume index among the normal, RCM, and CP groups. In the CP group, left atrial dimension, left atrial volume index, inferior vena cava dimension, mitral E velocity, and E/A ratio were significantly greater, whereas LV ejection fraction, deceleration time, and isovolumic relaxation time were significantly smaller compared with the normal group. Inferior vena cava dimension, mitral A velocity, septal e′, and lateral e′ were significantly greater, and left atrial volume index, interventricular septal thickness, LV posterior wall thickness, LV mass index, and E/septal e′ were significantly lower in the CP group compared with the RCM group. The lateral e′/septal e′ ratio was not significantly different between the CP and RCM groups, although it was significantly smaller in the CP group than in the normal group.
Parameter | Normal ( n = 20) | RCM ( n = 18) | CP ( n = 15) | Overall P value |
---|---|---|---|---|
Age (y) | 59 ± 15 | 56 ± 17 | 63 ± 18 | .488 |
Heart rate (beats/min) | 67 ± 12 | 70 ± 14 | 77 ± 15 | .139 |
LV end-diastolic dimension (mm) | 46 ± 5 | 47 ± 6 | 42 ± 5 | .062 |
LV end-diastolic volume index (mL/m 2 ) | 56 ± 11 | 61 ± 22 | 48 ± 13 | .063 |
Left atrial dimension (mm) | 37 ± 5 | 46 ± 6 † | 48 ± 10 ‡ | <.001 |
Left atrial volume index (mL/m 2 ) | 25 ± 4 | 67 ± 27 ‡ | 50 ± 16 †§ | <.001 |
Interventricular septal thickness (mm) | 10 ± 1 | 13 ± 4 ‡ | 9 ± 1 ¶ | <.001 |
LV posterior wall thickness (mm) | 9 ± 1 | 11 ± 2 † | 9 ± 1 || | .001 |
LV mass index (g/m 2 ) | 87 ± 15 | 142 ± 57 ‡ | 73 ± 20 ¶ | <.001 |
LV ejection fraction (%) | 69 ± 5 | 61 ± 10 ∗ | 61 ± 10 ∗ | .001 |
Systolic RV-RA pressure gradient (mm Hg) | 23 ± 5 | 34 ± 13 ∗ | 26 ± 12 | .017 |
Inferior vena cava dimension (mm) | 13 ± 3 | 15 ± 5 | 22 ± 3 ‡¶ | <.001 |
E (cm/sec) | 77 ± 16 | 107 ± 17 † | 103 ± 45 ∗ | .003 |
A (cm/sec) | 73 ± 16 | 46 ± 15 † | 71 ± 35 § | .001 |
E/A ratio | 1.11 ± 0.35 | 2.52 ± 0.82 ‡ | 1.88 ± 1.23 ∗ | <.001 |
Deceleration time (msec) | 225 ± 35 | 144 ± 23 ‡ | 174 ± 44 ‡ | <.001 |
Isovolumic relaxation time, (msec) | 84 ± 16 | 60 ± 12 ‡ | 61 ± 20 ‡ | <.001 |
Septal e′ (cm/sec) | 9.1 ± 2.6 | 6.5 ± 1.6 ∗ | 10.9 ± 3.4 ¶ | <.001 |
Lateral e′ (cm/sec) | 12.0 ± 3.2 | 7.8 ± 2.7 ‡ | 11.2 ± 2.4 || | <.001 |
E/septal e′ | 8.9 ± 1.8 | 18.0 ± 6.6 ‡ | 11.5 ± 8.7 § | <.001 |
Lateral e′/septal e′ ratio | 1.36 ± 0.31 | 1.21 ± 0.24 | 1.09 ± 0.27 ∗ | .022 |
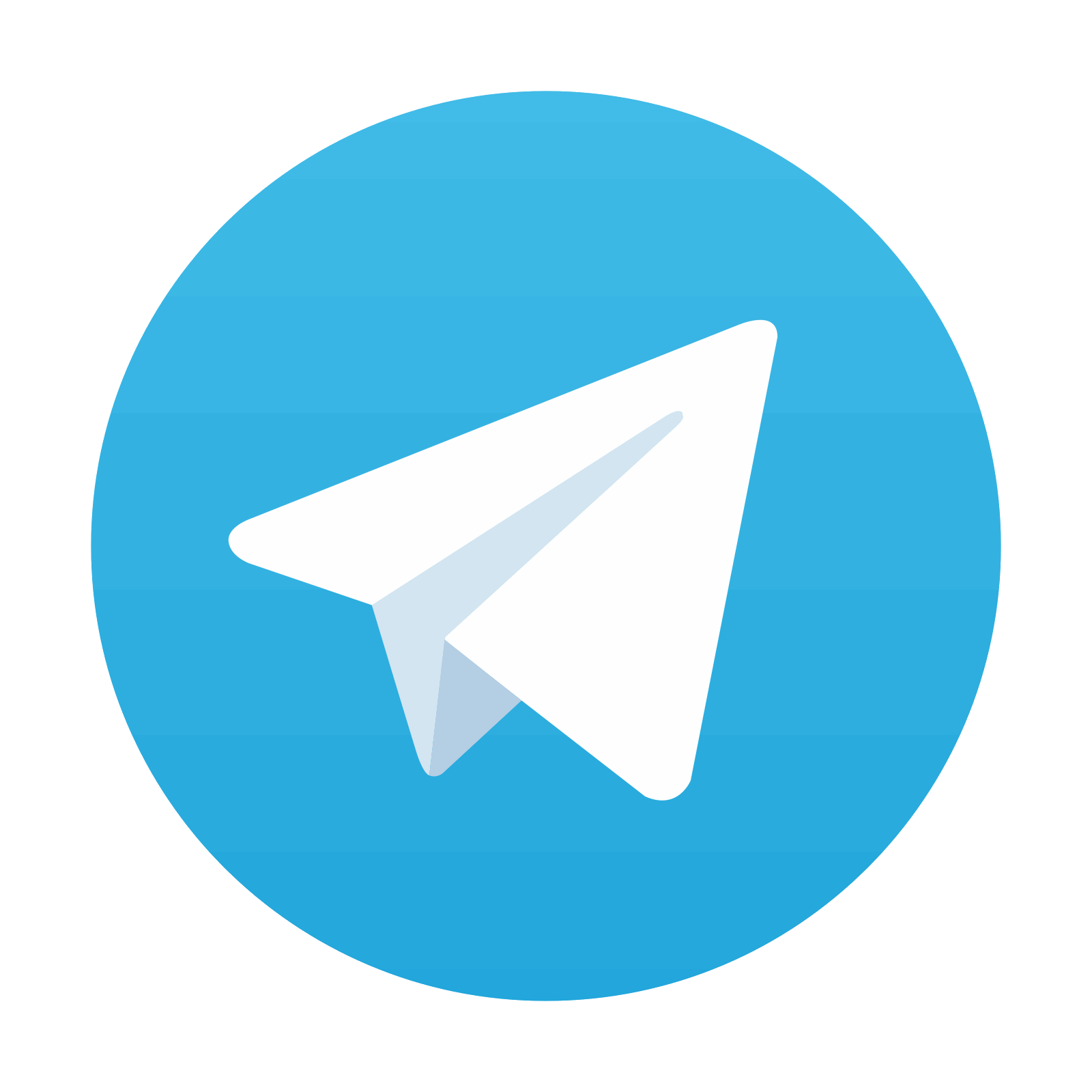
Stay updated, free articles. Join our Telegram channel

Full access? Get Clinical Tree
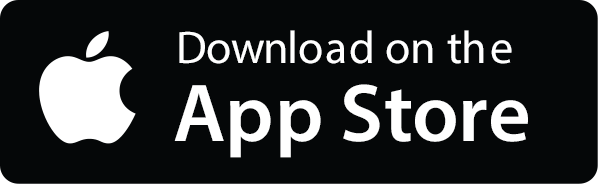
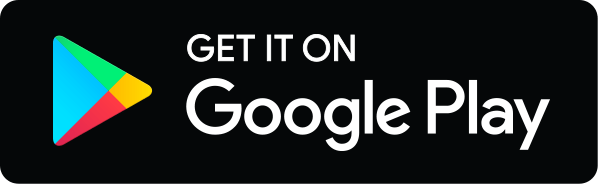
