Background
Quantitative assessment of the mitral annulus provides information regarding the pathophysiology of mitral regurgitation and aids in the planning of reparative surgery. Three-dimensional (3D) transthoracic echocardiographic data sets acquired with current scanners have enough spatial and temporal resolution to allow the quantitative analysis of the mitral annulus. Accordingly, the authors performed (1) a validation study to assess the agreement of quantitative analysis of the mitral annulus performed on 3D transthoracic echocardiography (TTE) and 3D transesophageal echocardiography (TEE) and (2) a normative study to obtain the reference values of 3D transthoracic echocardiographic parameters for mitral annular (MA) geometry and dynamics.
Methods
Mitral valve data sets were obtained by 3D TEE and 3D TTE in 30 consecutive patients with clinically indicated TEE (validation study) and 3D TTE in 224 healthy volunteers (aged 18–76 years) (normative study).
Results
In the validation study, MA measurements obtained by 3D TTE were similar to those obtained by 3D TEE ( P = NS). In the normative study, MA analysis by 3D TTE was feasible (94.5%) and reproducible (intraclass correlation coefficient = 0.78–0.97). MA diameters, area, and circumference were correlated with body surface area ( r > 0.50 for all) but not with age. Men had larger MA areas than women (4.9 ± 1.0 vs 4.5 ± 0.7 cm 2 /m 2 , P = .004). During systole, MA area decreased by 29 ± 5%. This decrease was related mainly to anteroposterior diameter shortening (20 ± 7%).
Conclusions
MA quantitative analysis by 3D TTE was accurate compared with 3D TEE in unselected patients with mitral valve disease. In healthy subjects, it was highly feasible and reproducible. The availability of reference values for MA geometry and dynamics may foster the implementation of MA quantitative analysis by 3D TTE in clinical settings.
Transthoracic echocardiography (TTE) is the standard clinical tool for the initial assessment and longitudinal evaluation of patients with mitral regurgitation (MR). Changes in the size, shape, and dynamics of the normal mitral annulus are closely related to the development of MR, and mitral annuloplasty is the most common surgical procedure to repair a regurgitant mitral valve (MV). Therefore, the quantitative assessment of mitral annular (MA) geometry seems to be important for a better understanding of MR pathophysiology and planning of reparative surgery. However, MA size is only rarely reported in clinical routine, and according to current recommendations regarding the echocardiographic assessment of patients with MR, the characterization of MA geometry is limited only to the measurement of MA anterior-posterior (A-P) diameter.
Linear or area measurements used to describe MA geometry using tomographic imaging techniques (e.g., two-dimensional [2D] echocardiography or cardiac magnetic resonance [CMR]) depend on the correct alignment of imaging planes and on the recognition of anatomic landmarks. In addition, they are unsuitable to fully characterize the complex nonplanar geometry of the mitral annulus and mitral leaflets. Conversely, three-dimensional (3D) echocardiography has the ability to provide anatomically sound images of the MV apparatus and to analyze the geometry and dynamics of the mitral annulus without geometric assumptions. Indeed, measurements of MV anatomy using 3D transesophageal echocardiography (TEE) have been reported to be accurate compared with surgical measurements and superior to those obtained by 2D TEE.
Until recently, MA quantitative assessment was feasible only with 3D data sets acquired from the transesophageal approach, so it was not practical for the routine assessment and follow-up of patients with MR. Third-generation 3D scanners have significantly improved the spatial and temporal resolution of 3D data sets acquired by 3D TTE, making feasible both the qualitative and quantitative analysis of the mitral annulus by 3D TTE. To the best of our knowledge, there are no data regarding the feasibility and accuracy of MA quantitative analysis performed on 3D transthoracic echocardiographic data sets, and data on reference values of MA parameters assessed using 3D TTE are quite limited.
To address these issues, we designed two consecutive, prospective studies. In a validation study, we compared MA quantitative assessment by 3D TTE against the same measurements obtained by 3D TEE, and in a normative study, (1) we obtained reference values for static and dynamic MA analysis from a large cohort of healthy volunteers, (2) we analyzed the relationships of normal MA geometry with age, gender, and body size, and (3) we assessed the feasibility and reproducibility of quantitative analysis of MA geometry using 3D TTE.
Methods
Study Population
Between July 2011 and October 2011, we enrolled consecutive patients in sinus rhythm with clinical indications for TEE to conduct the validation study.
To obtain normative values for MA size and geometry, healthy Caucasian volunteers were prospectively recruited among hospital employees, fellows-in-training, their relatives, and individuals who underwent medical assessments for driving or working licenses between October 2011 and February 2013. The inclusion criteria were age > 17 years, no history or symptoms of cardiovascular or lung disease, no cardiovascular risk factors (i.e., systemic arterial hypertension, smoking, diabetes, and hypercholesterolemia), normal results on electrocardiography and physical examination, and no cardio- or vasoactive treatment. Exclusion criteria were athletic training, pregnancy, body mass index > 30 kg/m 2 , and a poor apical acoustic window. Blood pressure was measured in all subjects immediately before the echocardiographic examination. The study was approved by the University of Padua Ethics Committee (protocol no. 2380 P), and both patients and volunteers provided informed consent before the study.
Echocardiography
All examinations were performed using standardized protocols and a commercially available Vivid E9 system (GE Vingmed Ultrasound AS, Horten, Norway) equipped with 4V and 6VT probes for 3D TTE and 3D TEE, respectively.
In the validation study, 3D full-volume MV data sets were acquired by 3D TTE from the apical approach in all patients, immediately before TEE. TEE was performed according to the specific clinical indication by the same experienced operator (L.P.B. or D.M.), and at the end, a 3D full-volume MV data set was acquired using the 3D zoom option ( Videos 1 and 2 ; available at www.onlinejase.com ).
In the normative study, all 224 healthy subjects underwent complete TTE to exclude subclinical heart diseases and poor apical acoustic windows. Two 3D full-volume acquisitions (i.e., one for the MV and a separate one for the left ventricle) were recorded by combining six consecutive electrocardiographically triggered subvolumes during a breath-hold ( Videos 3 and 4 ; available at www.onlinejase.com ).
Image Analysis
Three-dimensional transthoracic and transesophageal echocardiographic data sets for the MV and the left ventricle were stored digitally in raw-data format for offline analysis. Quantification of 3D left ventricular (LV) volumes and ejection fraction and 3D longitudinal strain was performed using a commercially available software package (4D AutoLVQ, EchoPAC BT 12; GE Vingmed Ultrasound AS) previously described and validated against CMR.
For the validation study, 3D transthoracic and transesophageal echocardiographic MV data sets were converted to Digital Imaging and Communications in Medicine format and analyzed using a dedicated software package for MV quantitative analysis (4D-MV Assessment version 2.3; TomTec Imaging Systems, Unterschleissheim, Germany) by a single observer, who performed the quantitative analysis of transesophageal and transthoracic echocardiographic data sets in random order, in a blinded fashion, with an interval of 1 week.
For the normative study, a single observer (S.M.) analyzed the 3D transthoracic echocardiographic MV data sets of the 224 healthy volunteers to obtain reference values for MA geometry and dynamics, using the same software package. The quality of MV data sets was judged subjectively as excellent, good, fair, or poor, considering the signal-to-noise ratio, the degree of blood-tissue contrast, and the quality of MA tracking. Poor-quality data sets were excluded from the study.
MA analysis on 3D transthoracic echocardiographic data sets started by identifying three time points: early systole (the frame after MV closure [MVC]), end-systole (the frame just before the MV begins to open, and mid-systole (the frame midway between MVC and end-systole). After adding anatomic landmarks for the mitral annulus, aorta, and leaflet coaptation point, the software created a static 3D model of the mitral annulus and leaflets at mid-systole. Afterward, the mitral annulus was tracked in each systolic frame (dynamic analysis) ( Video 5 ; available at www.onlinejase.com ). Manual edits of the dynamic models were performed as needed. Quantitative parameters of MV geometry ( Figure 1 ) were 3D and 2D (projected) MA areas; MA circumference; MA A-P diameter, as the shortest distance between the highest anterior and posterior points of the mitral annlulus; MA anterolateral-posteromedial (AL-PM) diameter, as the longest diameter of the mitral annulus; MA sphericity index, as the ratio between A-P and AL-PM diameters; MV commissural diameter, measured through the two MV commissures; MV anterior leaflet area (ALA) and length; MV posterior leaflet area (PLA) and length; MA nonplanarity angle (NPA), quantifying the “saddle shape” of the mitral annulus; annular height, as the distance between the lowest and the highest points of the mitral annulus; MV tenting height, area, and volume; and the angle between the aortic valve and mitral annulus along the A-P direction (Ao-AP angle). For all quantitative parameters, the values at MVC, mid-systole, and end-systole, the minimal absolute value, and the time interval from MVC to its minimal value (expressed as a percentage of the total duration of systole) were recorded. MA diameters, area, and circumference were normalized to body surface area (BSA).

The software provided MA displacement, displacement velocity, and MA area fractional change. In addition, the fractional changes (the difference between the maximal and minimal values, divided by the maximal value and expressed as percentages) of MA circumference, A-P diameter, and AL-PM diameter were also calculated ( Figure 2 ).

Statistical Analysis
Normal distribution of variables was checked using the Kolmogorov-Smirnov test. Continuous variables are summarized as mean ± SD, and categorical variables are reported as percentages. Variables were compared between men and women using unpaired t tests. In the validation study, 3D transesophageal and transthoracic echocardiographic measurements in the same subjects were compared using paired t tests and Bland-Altman analysis.
Comparison of MA measurements obtained at different reference frames during systole was made using analysis of variance for repeated measurements. Pearson’s correlation was used to analyze the relationships between age, BSA, and MA parameters, as well as the correlation between 3D transthoracic and transesophageal echocardiographic measurements of the mitral annulus.
Interobserver variability for MA assessment using 3D TTE was performed in 17 random healthy subjects by two independent observers (S.M. and D.M.) by blinded offline analysis of the same 3D data set. Intraobserver variability was assessed by one investigator (S.M.), who repeated the measurements of the same data sets 7 days later. Reproducibility was reported as the coefficient of repeatability (1.9 ± SD of the difference between the two measurements) using Bland-Altman analysis and as intraclass correlation coefficients.
All analyses were carried out using SPSS version 20.0 (SPSS, Inc, Chicago, IL) and MedCalc version 10.0.1.0 (MedCalc Software, Mariakerke, Belgium). Differences among variables were considered significant at P < .05.
Results
Validation Study
For the validation study, we enrolled 30 patients with clinical indications for TEE, such as Barlow disease ( n = 4), MV prolapse ( n = 6), patent foramen ovale ( n = 3), rheumatic mitral stenosis ( n = 2), infective endocarditis ( n = 8), and other indications ( n = 7). As expected, 3D transesophageal echocardiographic data sets had superior image quality than 3D transthoracic echocardiographic data sets (good or excellent quality in 81% vs 54%, respectively, P < .001). Twenty-eight data sets obtained by 3D TEE (93%) and 22 data sets by 3D TTE (73%) were adequate for MA quantitative analysis ( P = .08). The mean temporal resolution was 28 ± 13 volumes/sec for 3D TEE and 31 ± 6 volumes/sec for 3D TTE ( P = .408). Close correlations ( r > 0.89) ( Figure 3 ) and good agreement ( Figure 4 ) were found when comparing MA parameters measured by 3D TEE and 3D TTE.
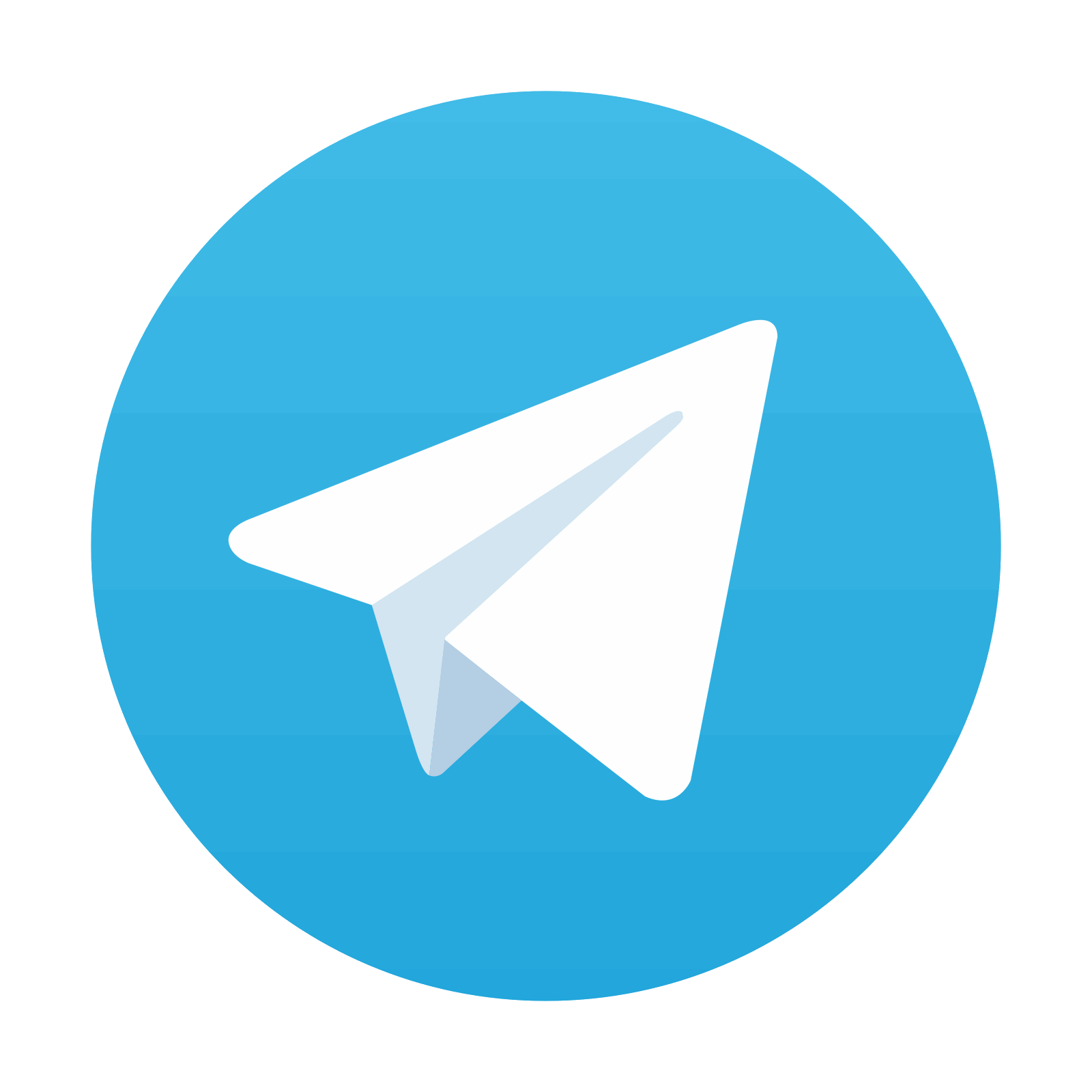
Stay updated, free articles. Join our Telegram channel

Full access? Get Clinical Tree
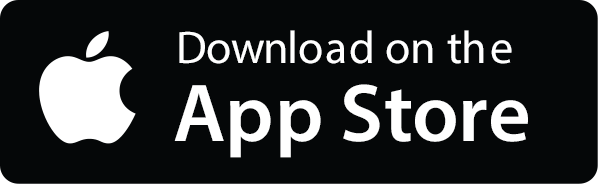
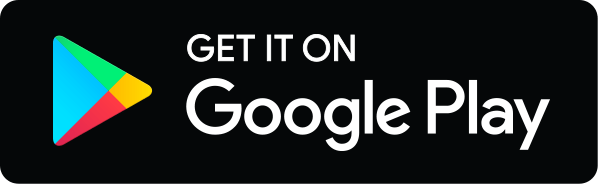
