Background
Myocardial contractility, a significant determinant of cardiac function, is valuable for diagnosis and evaluation of treatment in cardiovascular disorders including heart failure. Shear wave elasticity imaging (SWEI) is a newly developed ultrasound-based elastographic technique that can directly assess the stiffness of cardiac tissue. The aim of this study was to verify the ability of this technique to quantify contractility changes in the myocardium.
Methods
In 12 isolated rabbit hearts, SWEI measurements were made of systolic stiffness at five different coronary perfusion pressures from 0 to 92 mm Hg. The changes in coronary perfusion were used to induce acute stepwise reversible changes in cardiac contractility via the Gregg effect. The Gregg effect is the dependency of contractility on coronary perfusion. In four of the hearts, the measurements were repeated after delivery of gadolinium, which is known to block the Gregg effect.
Results
Systolic stiffness measured by SWEI changed linearly with coronary perfusion pressure, with a slope of 0.27 kPa/mm Hg (mean of 95% CI, R 2 = 0.73). As expected, the change in contractility due to the Gregg effect was blocked by gadolinium, with a significant reduction of the slope to 0.08 kPa/mm Hg.
Conclusions
SWEI measurements of systolic stiffness provide an index of contractility in the unloaded isolated rabbit heart. Although this study was done under ideal imaging conditions and with nonphysiologic loading conditions, it reinforces the concept that this ultrasound technique has the potential to provide a direct and noninvasive index of cardiac contractility.
Highlights
- •
Improved measures of contractility would affect the diagnosis of heart failure.
- •
Ultrasound SWEI can provide direct systolic measurement of myocardial stiffness.
- •
Stepwise changes in cardiac contractility were achieved using the Gregg effect.
- •
SWEI measurements of stiffness linearly correlated with contractile state.
Myocardial contractility or the inotropic state of the heart is one of the major indicators of cardiac function during systole. In many cardiovascular disorders, such as systolic heart failure and cardiomyopathies, the contractility of the heart decreases, and inotropic agents are used to raise the ejection force. Contractility is usually measured using methods such as dP/dt or by evaluating ejection fraction. On rare occasions, it is measured invasively using pressure-volume loop analysis. Recently, echocardiography-based tissue Doppler, tissue strain, and strain rate imaging have been used clinically to assess contractility, but these techniques do not provide contractility measurements of the myocardium independent of factors such as intraventricular volume and cardiac motion. It has been shown that transverse stiffness, measured using small high-frequency indentations, is an index of contractility in beating canine interventricular septum. However, none of the available clinical techniques measure systolic stiffness this directly.
Elastography is a newly developed image-based stiffness measurement technique that involves exciting the tissue mechanically and measuring the response. Imaging of the response has been done using both magnetic resonance and ultrasound imaging. To use the ultrasound-based technique, an acoustic pulse is used to push the tissue, and subsequent acoustic pulses are used to measure the mechanical response. As the tissue moves axially, a shear wave is launched in the transverse direction. The velocity of this wave is related to the stiffness modulus (shear modulus) of the tissue. This method is called shear wave elasticity imaging (SWEI). The technique is being used clinically to diagnose liver disorders by directly measuring liver elastance.
It has been shown that SWEI stiffness of the heart can be measured with intracardiac probes invasively. The acute change in tissue stiffness associated with cardiac ablation lesions has been characterized using this technique. Recently, transthoracic cardiac SWEI measurements in humans have been used to characterize diastolic stiffness. However, few reports describe SWEI measurements to characterize inotropic changes in cardiac tissue. Kolipaka et al. assessed the effect of epinephrine on myocardial contractility using magnetic resonance–based shear wave stiffness measurements. A study by Pernot et al. showed that SWEI can be used as a contractility index in the isolated rat heart with a constant volume balloon in the left ventricle. They demonstrated that the SWEI measurement was relatively independent of preload volume when tested with a constant volume ventricle and that the SWEI-determined modulus of elasticity, maximum dP/dt, and peak systolic pressure changed in a dose-dependent manner after the administration of an inotropic agent (isoproterenol).
In this study, we used the “Gregg effect” phenomenon in a Langendorff perfused rabbit heart model to investigate the potential of SWEI to quantify changes in cardiac contractility in the absence of preload and afterload. Researchers have shown that coronary perfusion pressure affects myocardial contractility during systole; this is known as the Gregg effect. It has been shown that the circumferential stress caused by increased perfusion pressure causes a mechanical deformation in the membrane of cardiomyocytes. This deformation changes ion flux through stretch-activated channels, resulting in increased calcium delivery to the myocytes and increased contractility. Further details about this effect can be found in the second and third paragraphs of the discussion section. This effect can be blocked by gadolinium, a stretch-activated ion channel blocker. The Gregg effect in combination with the control of perfusion afforded by the Langendorff preparation allowed us to induce stepwise and random changes in cardiac contractility. In addition, the contractility changes are acute and reversible, unlike with most inotropic agents. The purpose of this study was to produce multiple reversible levels of contractility to verify the ability of the SWEI elastographic method to quantify the inotropic state of the heart in the unloaded condition.
Methods
Imaging Setup
Ultrasound-based SWEI measurements were made using a Siemens Sonoline Antares scanner (Siemens Healthcare, Mountain View, CA). Imaging was performed using the VF10-5 linear probe. The acoustic radiation force pulse used to push the tissue was 300 cycles at 5.7 MHz. The focus was set at 16 mm and the tracking pulse repetition frequency was 4.3 kHz. Displacement estimation algorithms were used to calculate the shear wave velocity.
The long acoustic pulse produced a radiation force that displaced the tissue in the focal region by transferring momentum from the ultrasound wave to the tissue. This transfer of momentum is also a significant portion of the mechanism of attenuation. The push caused displacements on the order of micrometers in the direction of ultrasound propagation. The rapid “point” displacement generated a shear wave that propagated perpendicular to the push. The velocity was calculated by tracking the displacements caused by the propagating wave using regular ultrasound at several lateral locations. For further information regarding the signal-processing techniques, see the publications by Loupas et al. and Rouze et al. The intrinsic motion of the heart was subtracted using a quadratic motion filter before calculating the shear wave speed of propagation. The following formula (assuming a linearly elastic isotropic medium) relates the shear modulus of stiffness to the shear wave velocity, C t (m/sec):
C t = μ ρ = E 2 ( 1 + v ) ρ ,
Animal Model
An isolated heart preparation was created using hearts from New Zealand white rabbits ( n = 12; mean weight, 3.68 ± 0.64 kg). The study was performed according to the Institutional Animal Care and Use Committee at Duke University and conformed to the Guide for the Care and Use of Laboratory Animals. Rabbits were heparinized and anaesthetized using intramuscular and intravenous xylazine and ketamine until the reflexes disappeared. A bilateral thoracotomy was performed, and the heart removed and placed in Tyrode’s solution at 0°C to 4°C. The aorta was cannulated and perfused with Tyrode’s solution in Langendorff mode. The Langendorff preparation keeps the heart alive and beating for several hours by retrograde aortic perfusion. The Langendorff apparatus was built using a combination of Radnoti glassware (Radnoti, Monrovia, CA) and custom-built parts. Tyrode’s solution was made fresh on the day of the experiment, and the concentrations of Ca 2+ , Na + , and K + and pH were maintained in the normal range. P o 2 was maintained at >300 mm Hg. The isolated heart was submerged in a saline bath maintained at 37°C to 38°C.
The coronary arteries in an isolated heart can be perfused in two configurations: Langendorff and working. In the Langendorff configuration, the aorta is perfused retrogradely and the aortic valve remains closed, the coronary arteries, located above the valve, are perfused, and the left ventricle does not receive fluid through the patent aortic valve. In the working configuration, the heart is perfused similarly to a normal beating heart, in which the left atrium receives flow and the left ventricle ejects fluid out through the aorta and into the coronary arteries. Our experiments were performed in the Langendorff configuration.
Experimental Design
A schematic of the experimental setup is shown in Figure 1 . The preparation was designed to avoid fluid buildup in the left ventricle to minimize the load dependency of our measurements. This was done by retrogradely perfusing the aorta, keeping the atrium above the fluid level of the bath as much as possible, and placing a vent through the mitral valve to keep it open and prevent a pressure buildup in the left ventricle. Thus, the preload and afterload in these hearts were near zero.

Short-axis images of the LV free wall were taken from a distance of 10 to 15 mm. Shear wave velocity was calculated by tracking the wave as it moved through 5 mm of myocardium laterally. To minimize the effects of anisotropy, displacements were averaged over the wall thickness axially (3–5 mm) before the velocity was calculated. Electrodes were placed in the bath, and the electrocardiogram was recorded using a LabChart/PowerLab data acquisition system (ADInstruments, Sydney, Australia). The maximum shear wave velocity recorded through the cardiac cycle was identified, and its systolic timing was confirmed by simultaneous electrocardiography. Occasionally the algorithm could not determine an accurate shear velocity, because of noise in the displacement measurement; in these cases (six of 96 measurements), the data point following the noisy maximum point during that cardiac cycle was used. The noisy points were identified as points with shear wave speeds >9 m/sec or by a tissue displacement profile that did not follow a wave propagation pattern.
The Langendorff configuration can be perfused in one of two modes, constant pressure or constant flow, in which perfusion of the aorta and the coronary arteries is achieved by maintaining a constant pressure or a constant flow, respectively. In these experiments, the hearts were perfused in constant-pressure mode, set by the height of the perfusion chamber connected to the aorta. The identical random sequence of perfusion steps was used in each heart: [75, 25, 75, 50, 75, 0, 75, 92] mm Hg. Coronary perfusion pressure changes were achieved by adjusting the height of the perfusion fluid chamber. After each change, the coronary perfusion pressure was returned to normal (75 mm Hg), and the measurements were repeated. The normal perfusion pressure for Langendorff rabbit hearts has been reported in the literature to range between 60 and 100 mm Hg. We chose 75 mm Hg for all the hearts to maintain consistency for the measured perfusion pressure steps between the hearts. The total time required to obtain measurements for the entire sequence of pressures was <30 min. In four of the hearts, after the initial measurements, gadolinium (GdCl 3 ·6H 2 O) at a concentration of 10 μmol/L was added to the perfusate, and the hearts were perfused for 30 min. The SWEI measurements at each perfusion pressure step were then repeated.
Statistical Analysis
Statistical analysis was performed using R (R Foundation for Statistical Computing, Vienna, Austria) and MATLAB (The MathWorks, Natick, MA). A multivariate linear regression model was used with coronary perfusion pressure steps as an independent continuous variable and rabbit hearts as a categorical variable. Rabbits were treated as a categorical variable to account for between animal differences including imaging site and intrinsic myocardial characteristics.
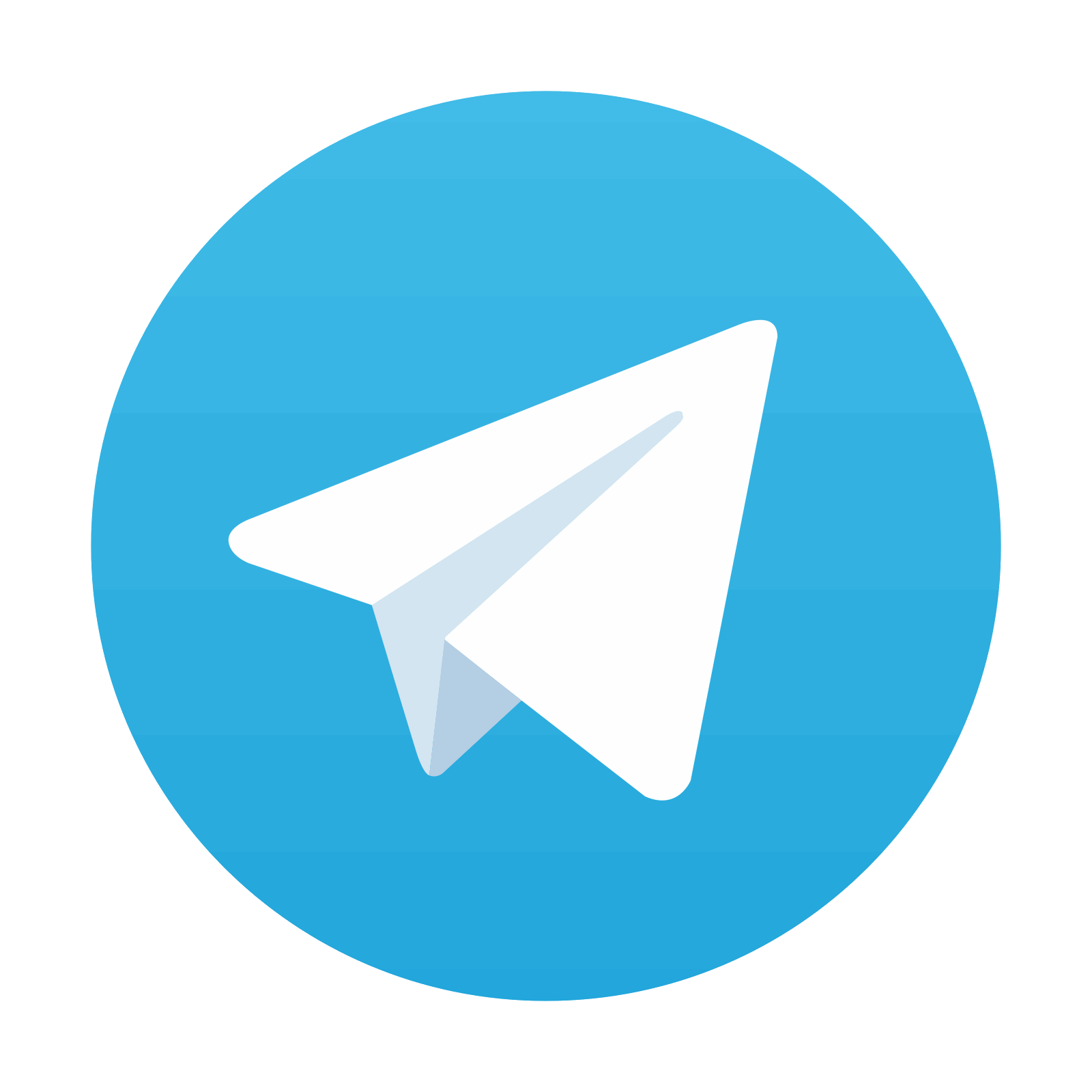
Stay updated, free articles. Join our Telegram channel

Full access? Get Clinical Tree
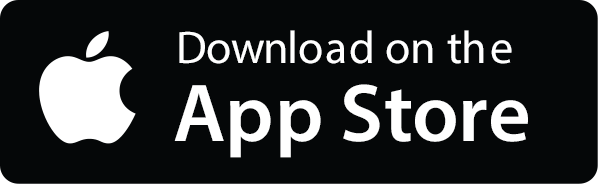
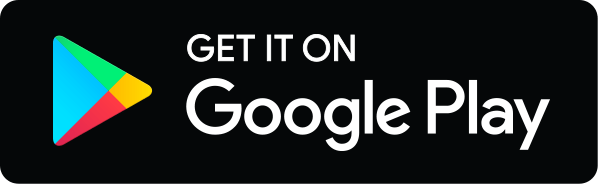