Mitral valve regurgitation (MR) is among the most prevalent and most significant valve problems in the Western world. Yet the accurate quantification of MR remains a significant challenge for cardiologists. Within a single echocardiography lab, the interobserver variability for the assessment of MR severity may be quite humbling. Despite the publication of extensive guidelines by the American Society of Echocardiography and evidence of the prognostic value of quantification of regurgitation, a busy clinician must still decide, Is this degree of MR important for this patient? Undoubtedly, a small regurgitant volume can be considered a mild condition if no clinical consequence is expected. Likewise, a very large regurgitant volume that is predicted to directly cause symptoms or adverse cardiopulmonary adaptation could be called a severe lesion. But what does a moderate or moderate to severe designation really mean to patient and physician? Do such labels really help us make clinical predictions or treatment decisions? At least for those patients in this “moderate” gray zone, quantification (not qualification) of the regurgitant severity is the first step in rational and consistent decision making. Through the years, we have developed many new tools to provide the numbers. The newest of these tools are discussed below.
Evaluation of the zone of flow convergence proximal to the regurgitant valve, using the proximal isovelocity surface area (PISA) method, is a terrific concept but thus far a disappointing clinical tool. In theory, flow accelerating toward a regurgitant orifice will form a series of concentric isovelocity shells, each smaller in surface area and higher in velocity as they approach the orifice. If the orifice is a very small circle, the shells will be hemispheres. By calculating the shell area (from its radius) and multiplying by its velocity (the color Doppler aliasing velocity), the regurgitant flow rate and effective regurgitant orifice area (EROA) can be calculated. Unfortunately, clinically encountered MR is usually associated with an irregularly shaped regurgitant orifice, and the PISA shape is often not hemispheric. Most recently, three-dimensional (3D) echocardiography has been used to image the zone of flow convergence and to visualize its shape, thereby allowing a modified PISA method devoid of geometric assumptions. Several recent reports using specialized software have demonstrated improved accuracy in 3D PISA–derived EROA and regurgitant volume. It remains to be seen if proximal flow convergence can be accurately, rapidly, and reproducibly exploited using 3D color Doppler methods in the clinical setting.
Like the regurgitant flow proximal to the orifice, the flow profile immediately downstream (on the left atrial side) of the orifice is also an interesting and perhaps more fruitful target for 3D color Doppler characterization. This zone, the vena contracta (VC), is identified by a narrow, high-velocity spectrum of laminar flow just distal to the regurgitant orifice. On the basis of fluid dynamic principles, the VC should be independent of flow and pressure changes as long as the regurgitant orifice remains constant. As such, the VC represents a near ideal flow component to define the severity of valve regurgitation. In addition, the Doppler representation of this parameter is less sensitive to technical factors. The concept of flow contraction explains why the area of the VC, also described as the EROA, is smaller than the true anatomic orifice area. The VC flow contraction coefficient has been reported as 0.65 to 0.85 ; that is, the EROA is only 65% to 85% the area of the anatomic regurgitant orifice area. Two-dimensional (2D) echocardiographic measurement of the VC width of a regurgitation jet in a parasternal long-axis view (ideal axial Doppler resolution) or apical 4-chamber view (less ideal lateral Doppler resolution) has evolved into an accepted and recommended estimate of MR severity. However this concept is based on the assumption of EROA being constant and nearly circular. Both in vitro and in vivo studies have demonstrated that the measurement of the 2D VC diameter tends to overestimate the EROA. As such, 2D measures of the VC are not truly quantitative but still serve a role as a semiquantitative Doppler assessment tool. Recent reports have demonstrated that the etiology of MR and local leaflet pathology can profoundly affect the shape of the regurgitant orifice and the closely associated VC area. For example, a patient with functional MR on the basis of asymmetric tethering of the chordae tendineae may have a crescent-shaped regurgitant orifice and an eccentric MR jet direction. A regurgitant lesion with such complex geometry and flow would not be well approximated by a simple VC width measurement. Investigators are now using 3D color Doppler imaging to explore and better quantify these complex flow events.
Real-Time Three-Dimensional Echocardiography
In general, 3D echocardiography has fulfilled its early promise. With transthoracic or transesophageal probes, we can now readily acquire large volumetric data sets and apply relatively simple processing tools to assess cardiac chamber volumes, congenital malformations, and valve morphology and motion. Over the past few years, many investigators have examined the current or potential role of 3D color flow imaging (CFI) to assess valve function. Although the technology is rapidly evolving, most commonly, a 3D color Doppler data set is acquired using “real-time” applications that capture several (typically 7) electrocardiographically gated sequential subvolumes, each from a cardiac cycle. These subvolumes are stitched together rapidly (<1 second) to create one large volume of color Doppler data. Although the computer processing (stitching time) is fast, it does affect the ultrasound sample frequency; hence the relatively low frame rate (10-30 Hz) for 3D CFI. The reconstruction from subvolumes can create considerable data artifacts if there is significant respiratory motion or heart rate irregularity during data acquisition. Recently developed, transthoracic 3D CFI based on a single heartbeat (without stitching) is likely to be an important technical advance.
For the assessment of MR severity, the 3D CFI data set must be cropped to reveal the regurgitant flow events. From the available systolic frames (usually 4-8 frames), the maximal MR jet is readily identified. As shown in Figure 1 , the data set is cropped and rotated to align orthogonal cut planes along the long axis of flow. Once the long axis is defined, the short axis is inspected to identify its smallest area distal to the orifice. This is the VC area and is also the EROA at that point in the cardiac cycle. Kahlert et al demonstrated that this processing can be done fairly rapidly (2.6 ± 0.7 minutes). The limitations typical of the 2D VC method as well as the strengths of the 3D VC method are clearly evident in Figure 1 , depicting functional MR. Two-dimensional Doppler imaging, lacking the ability to perform free rotation, does not permit the identification of the true short-axis VC area. In addition, a 2D VC diameter measured from any single plane does not relate well to a crescent-shaped flow area.

Novel Approaches to Mitral Regurgitation Quantification
In theory, the path to a quantitative evaluation of MR flow is fairly direct. The regurgitant flow rate (in cubic centimeters per second) we seek to quantify is simply the product of flow area (in square centimeters) and flow velocity (in centimeters per second). With several currently available software packages, it is now possible to rapidly crop a good-quality real-time 3D echocardiographic data set to identify and measure the VC area of any given MR jet. The challenge remaining is to measure the flow velocity within that same region of interest.
In this issue of JASE , Skaug et al present a study in which they used multibeam high–pulse repetition frequency (HPRF) 3D color Doppler to assess VC area and flow rate. This method is based on the recognition that blood flow at the VC is essentially laminar. As such, the backscattered Doppler power is proportional to the volume of blood within a defined ultrasound sample volume. When properly calibrated, this backscattered Doppler power can be used to measure flow volume. As first demonstrated by Buck et al, when a Doppler signal is applied to the VC of a regurgitant jet, the power of the backscattered Doppler signal is linearly proportional to the cross-sectional area of the VC. In addition, the product of power multiplied by velocity and integrated over the VC velocity spectrum is proportional to the total instantaneous flow through the VC. The work reported by Skaug et al is an extension of this principle using multiple 3D Doppler beams to cover the entire VC region. The technique is 3D because the region of interest is 3D and multigated in the radial direction. Because the ultrasound beam with the most Doppler power is likely to be within the regurgitant jet or orifice, novel computing software is used to compare the power of the composite beam to that of the reference beam and solve for the unknown area: the area of the VC.
Skaug et al applied this technique in 27 patients with MR of varying severity. Using a customized transthoracic probe, the VC area was measured from the backscattered Doppler power, while the regurgitant volume was calculated as the product of the VC area and the velocity-time integral of the regurgitant jet found separately by continuous-wave Doppler. The derived VC area was compared with a reference standard of regurgitant volume derived from magnetic resonance imaging (MRI). The authors report a good correlation of derived regurgitant volume between MRI and HPRF multibeam Doppler methods (Spearman’s rank correlation, 0.82), with little measurement bias (95% limits of agreement, −3.0 ± 26 mL). Appropriately, Skaug et al explored the accuracy of this new technique on the basis of the severity and etiology of MR. Similar to previous studies of VC area, Skaug et al demonstrated that their method to derive the VC area correlated less well in the patient group with functional MR and in the subgroup with mild MR. For patients with mild MR (defined by regurgitant volume < 30 mL on MRI), there was a significant difference between techniques, with a mean regurgitant volume difference of −11.8 mL. In patients with moderate and severe MR (defined by regurgitant volume > 30 mL on MRI), the mean difference between the multibeam HPRF and MRI measurements of regurgitant volume was only 5.2 mL. In organic MR, the mean difference in regurgitant volume was 1.1 mL, whereas for functional MR, the mean difference was greater, at −8.8 mL. The authors discuss this finding and suggest that the difficulty in measuring the VC area for small or very irregularly shaped orifices (as encountered with functional MR) likely resulted from the power Doppler reference beam being too large for the orifice. Although the cross-sectional area may be large in functional MR, the orifice may not encompass the entire reference beam. They suggest that this is a solvable problem and expect that the next generation of 3D probes will have an increased aperture size, allowing narrower reference beams to more accurately measure the narrow asymmetric orifice of functional MR.
Importantly, when regurgitant volume was measured by multibeam HPRF color Doppler and compared with the results of MRI in patients with moderate and severe MR (the clinical group most in need of accurate MR quantification), there was no significant measurement bias. Skaug et al conclude that multibeam HPRF color Doppler regurgitant volume, calculated as the product of VC area and the velocity-time integral by continuous-wave Doppler, can supplement 2D Doppler for the quantification of moderate and severe MR.
There are several potential advantages to this new technique. Unlike direct measurement with planimetry of the VC area by 3D CFI, multibeam HPRF color Doppler estimates the VC area on the basis of a calibrated measurement of the backscattered Doppler power and requires no manual tracing, and the calculations can be done semiautomatically, are not affected by display settings such as gain and tissue priority, and are not restricted by a Nyquist limit of <1 m/s. In addition, this novel technique incorporates measures of the VC area from all systolic frames. For regurgitant lesions with a dynamic orifice area, this integration of multiple systolic area assessments is an important advance over 2D and recently described 3D methods that measure only the single largest VC diameter and area, respectively. Although promising, the method described by Skaug et al does have limitations. Like all Doppler methods, the area estimation by multibeam HPRF color Doppler is angle dependent, and misalignment of the Doppler interrogation beams will overestimate the VC area. In addition, this HPRF 3D color Doppler method is entirely novel and will require further validation. However, as a new tool to accurately quantify at least moderate MR, this technique holds significant promise.
In another recent report, Plicht et al described yet another novel method using a real-time 3D echocardiographic color Doppler data set to quantify MR flow volume. Essentially, they developed a method to measure the VC area as well as the VC velocity spectrum from a single set of 3D CFI data. To overcome the limitation of multiple aliasing of regurgitant flow velocities at the regurgitant lesion, they applied a novel dealiasing method based on the concept of a relatively narrow velocity spectrum with laminar flow at the VC, as opposed to turbulent flow within the regurgitant jet. In brief, the narrow color Doppler spectrum of high velocities at the VC can be divided into 2 components: a nonaliased component visualized by color Doppler across the VC area that fits within a Nyquist range with maximum baseline shift and a second component of multiple aliasing that can be unmasked by knowing the maximum regurgitant velocity from continuous-wave Doppler. With this method, total MR flow is calculated as the sum of nonaliased and aliased flow. Plicht et al recently evaluated this approach using an in vitro flow model and described its application is a small group of patients. From the in vitro study, flow rates calculated solely from real-time 3D data sets demonstrated a very good correlation with actual flow rates ( r = 0.99), with a small mean difference of −0.05 ± 0.5 mL/s. Also applied clinically in 23 patients with MR, the dealiased VC flow was compared with MRI-derived mitral regurgitant stroke volume. They also compared this dealiased VC flow method with 2D PISA–derived regurgitant volume using hemispheric or hemielliptical assumptions. In vivo, the correlation between MR stroke volume (by the dealiasing method) and MRI was very good ( r = 0.91; mean difference, −1.8 ± 7.1 mL), better than the correlations between MRI and hemiellipitcal PISA ( r = 0.89; mean difference, −11.7 ± 7.4 mL) and hemispheric PISA ( r = 0.81; mean difference, −17 ± 9.4 mL). From this initial clinical application, it appears that that the real-time 3D echocardiographic dealiasing method is largely independent of the mechanism and morphology of MR, because it determines the true shape and size of the VC area on the basis of color Doppler representation of regurgitant flow without geometric assumptions about the flow field distant to the orifice. A current limitation of this method is the reliance on relatively good quality 3D CFI data, the quality of which is expected to improve as single-beat CFI ultrasound platforms become widely available. Additional potential limitations include Doppler angle error and the temporal resolution of current 3D CFI data sets. Despite these modest limitations, this dealiasing method is well positioned to become a useful quantitative tool if it can be repackaged into a semiautomated method in which all steps of the dealiasing method are automated after the manual definition of the VC and flow direction.
These recent explorations highlight some of the ways in which new technologies and 3D CFI are being applied to address the now chronic problem of reliable MR quantification. Thus far, the clear advantage of these methodologies lies in their ability to directly visualize even a highly asymmetric VC area. Other important advantages of the techniques discussed are the ability to negate the technical and display factors that limit standard Doppler imaging (the method of Skaug et al ) and the ability to define geometric data (VC area) and velocity profile from a single 3D CFI data set (the method of Plicht et al ). Although these early data are compelling, more work must be done to make these tools clinically practical. As we have learned from the introduction of many new imaging applications, the key to clinical impact lies with a rapid and well-defined workflow that incorporates at least semiautomatic measurement protocols. Although the early expectations of 3D echocardiography have been fulfilled, the emerging role of 3D CFI for the quantification of MR severity has begun to redefine those prospects.
Editorial Comments published in the Journal of the American Society of Echocardiography (JASE) reflect the opinions of their author(s), and do not necessarily represent the views of JASE, its editors, or the American Society of Echocardiography.
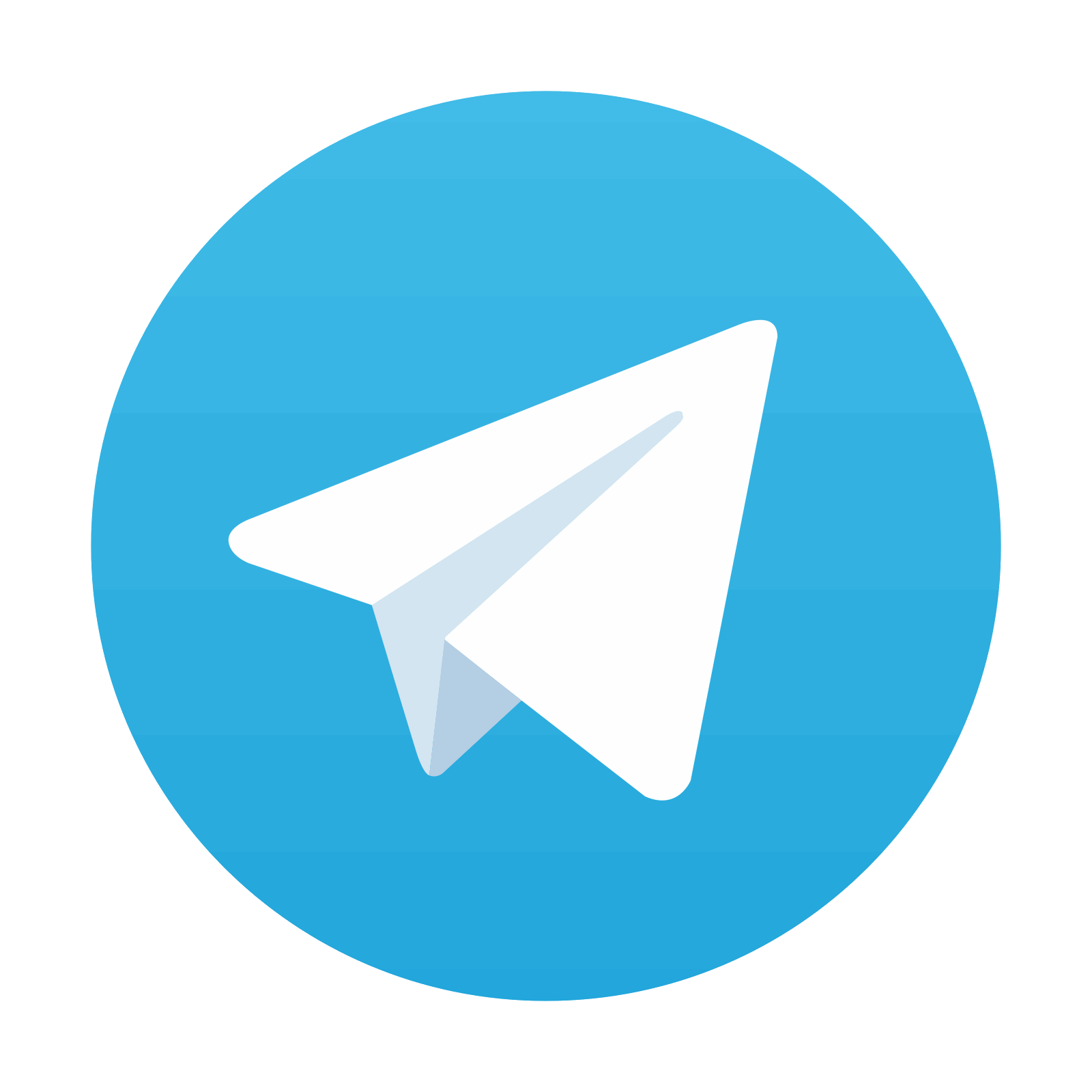
Stay updated, free articles. Join our Telegram channel

Full access? Get Clinical Tree
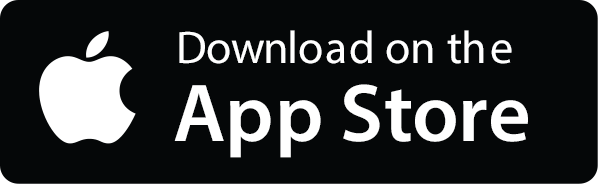
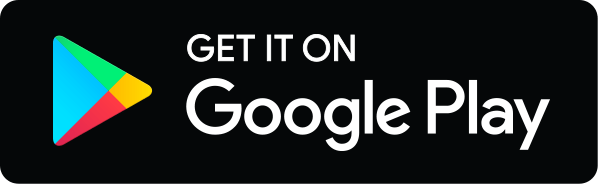