Background
Global longitudinal strain (GLS) derived from two-dimensional speckle-tracking is an emerging technology, but lack of industry standards limits its application. Prior studies support using this tool to identify subclinical disease through serial changes, but the variability introduced by a change in vendor or reader is not well defined.
Methods
Fifty study subjects were prospectively identified to include four subgroups to ensure a broad range of GLS: normal ( n = 20), left ventricular hypertrophy ( n = 10), ST-segment elevation myocardial infarction ( n = 10), and systolic heart failure ( n = 10). Raw data were obtained using equipment from two vendors during the same session, and GLS was analyzed using an offline workstation. Intraobserver and interobserver variation was measured using correlation coefficients, intraclass correlation coefficients, and Bland-Altman plots.
Results
GLS measurements were highly reproducible by the same reader or a different reader using vendor 1 and vendor 2 or comparing vendors (correlation coefficients and intraclass correlation coefficients ≥ 0.95). However, the Bland-Altman plots suggested that the variation in repeat GLS measurements may range from ± 2% to ± 5% on the basis of a change in vendor, reader, or both.
Conclusions
The expected variation in GLS measurements associated with a change in vendor, reader, or both should be considered when making conclusions about significant changes in serial measurements.
Echocardiography has had the potential to provide a quantitative measurement of left ventricular (LV) contractility for more than a decade, but clinical applications of noninvasively derived strain have been limited because of a lack of high reproducibility. In the early 2000s, there was great promise for strain derived by tissue Doppler. As more investigators used this tool, the associated weaknesses of angle dependency and high signal-to-noise ratio in abnormal ventricles became more apparent. In the late 2000s, advances in imaging technology and the advent of two-dimensional (2D) speckle-tracking echocardiography (STE) allowed strain measurements to be derived without the limitations of angle dependency with greater signal fidelity. This field is now on the cusp of becoming a clinically useful tool in chemotherapy-induced cardiomyopathy, LV dyssynchrony, subclinical valve disease, and ischemic heart disease, but questions remain about reproducibility and establishing measurement standards across different vendors.
Concerns about the reproducibility of 2D strain measurements culminated in 2010 at the EuroEcho meeting in Copenhagen, with the creation of the Industry Strain Standardization Task Force, which was a joint initiative of the European Association of Cardiovascular Imaging and the American Society of Echocardiography. In 2011 and 2012, these organizations generated an expert consensus document on quantitative evaluation of cardiac mechanics and emphasized that for 2D STE to become a more widely adopted clinical tool, significant improvements in variation across different vendors are needed. Since that time, multiple studies have evaluated 2D STE using multiple combinations of different vendors’ systems and software analysis packages, and overall improvements are being seen. Although there are different measures of strain within the left ventricle (longitudinal, circumferential, and radial), technical studies have demonstrated longitudinal strain as the parameter with the lowest variability, and therefore, many clinical studies have focused on global longitudinal strain (GLS) as a target to track for subclinical changes over time. However, as this new technology gains traction for clinical applications, it is important to define the magnitude of variation that might be anticipated in a real-world setting.
We hypothesized that by studying a series of patients with a wide range of GLS values, we would be able to quantify the variability introduced by a change in the imaging equipment, a change in the reader, or both in order to help define the variability one might expect with serial measurements in clinical practice. By defining the magnitude of this variation, one might be able to help distinguish normal variation from a clinically significant change.
Methods
A total of 50 participants were prospectively enrolled in this study, with four subgroups that were preselected to ensure that a broad spectrum of GLS was represented. These groups were as follows: normal volunteers ( n = 20), patients with LV hypertrophy ( n = 10), patients with ST-segment elevation myocardial infarctions ( n = 10), and patients with congestive heart failure (CHF) due to systolic dysfunction ( n = 10). The normal volunteers were all screened with a health questionnaire demonstrating no history of heart disease, no significant risk factors, and normal results on echocardiography. Other patients were recruited via the clinical echocardiography laboratory. LV hypertrophy was determined as wall thickness ≥ 1.2 cm. Patients with ST-segment elevation myocardial infarctions had echocardiographic images obtained within 48 hours of admission (four anterior, four inferior, and two lateral). Patients in the CHF group had to have CHF as a clinical indication for the study and an LV ejection fraction (LVEF) < 45%. Additional patient characteristics were obtained through review of the electronic medical record. Patients were excluded from the study if they had significant arrhythmia or poor acoustic windows. This study was approved by the institutional review board, and consent was obtained for participation.
Echocardiography
Two transthoracic studies were obtained for each patient. An initial transthoracic echocardiogram was obtained using either vendor 1 (Vivid E9; GE Healthcare, Milwaukee, WI) or vendor 2 (iE33; Philips Medical Systems, Andover, MA) in random order on the basis of machine availability. Immediately after completion of the first examination, a repeat set of images for 2D STE were obtained using the alternative vendor. The same sonographer obtained the images for both examinations on the same individual patient. The time between the two studies was estimated at <10 min. Images obtained for 2D speckle-tracking echocardiographic analysis included apical two-chamber, three-chamber, and four-chamber views with three consecutive cycles and a frame rate between 40 and 80 Hz. Continuous-wave Doppler across the aortic valve was obtained to determine the timing of aortic valve opening and closure. A quantitative LVEF was estimated from both studies using the biplane Simpson’s method for all study subjects.
Image Analysis for Vendor 1
The images obtained on the GE system were stored and transferred to a computer workstation for offline analysis using a commercially available vendor-specific software program (EchoPAC PC version BTO 11; GE Healthcare). Continuous-wave Doppler was used for event timing of aortic valve opening and closure. Using the Automated Function Imaging software (GE Healthcare), the reader identifies the LV basal segments and the apex. A region of interest covering the left ventricle is automatically generated and segmented by the software. The reader visually inspects the speckle-tracking of the LV segments and either approves the strain measurements or manipulates the region of interest to attempt to improve the tracking for measurement. The Automated Function Imaging software generates a bull’s-eye diagram that displays the peak systolic global and segmental longitudinal strain values. This process was performed by two separate readers, reader 1 (T.A.B.) and reader 2 (P.V.), and later repeated by reader 1 to establish intraobserver variability. There was a gap of ≥2 weeks between reading sessions.
Image Analysis for Vendor 2
The images obtained on the Philips system were stored and transferred to a computer workstation for offline analysis using a commercially available vendor-specific software program (QLAB version 9.0; Philips Medical Systems). Continuous-wave Doppler was used for event timing of aortic valve opening and closure. Using the QLAB software package, the reader identifies the LV basal segments and apex and visually inspects the tracking of the myocardium once all segments have been verified. Peak systolic GLS was also recorded from the bull’s-eye diagram. This process was performed by two separate readers, reader 1 (P.V.) and reader 2 (J.L.R.), and later repeated by reader 1 to establish intraobserver variability. There was a gap of ≥2 weeks between reading sessions.
Statistical Analysis
Intraclass correlation coefficients (ICCs), Pearson’s correlation coefficients, and Bland-Altman statistics were used to assess the intraobserver, interobserver, and intervendor variation. Results are displayed in tabular format, as correlation plots, and as Bland-Altman plots with bias (mean difference) and 95% limits of agreement (LOA). Student’s t tests were used to compare means when appropriate. Two-sided P values < .05 were considered statistically significant. Statistical analyses were performed using MedCalc for Windows version 12.4.0.0 (MedCalc Software, Mariakerke, Belgium).
Results
Patient and Echocardiographic Characteristics
A total of 50 study subjects were prospectively enrolled in this study, with four predefined subgroups to represent a spectrum of GLS. The baseline characteristics are shown in Table 1 . The overall average age was 59 ± 17 years, and 48% of participants were women. The average heart rate during image acquisition was 66 ± 12 beats/min for vendor 1 and 67 ± 12 beats/min for vendor 2 ( P = NS). The average frame rate during 2D STE for vendor 1 was slightly higher compared with that for vendor 2 (50.6 ± 5.2 vs 48.1 ± 7.2 frames/sec, P < .01). The average LVEF was 53 ± 16% (range, 12%–75%). The highest average LVEF was in the normal group (64 ± 5%), and the lowest was in the CHF group (30 ± 10%). The average and range of GLS measurements are described in the following sections.
Variable | MI | LVH | CHF | Normal | Total |
---|---|---|---|---|---|
Age (y) | 65 ± 15 | 73 ± 12 | 68 ± 10 | 45 ± 12 | 59 ± 17 |
Women | 6 (60%) | 4 (40%) | 7 (70%) | 7 (35%) | 24 (48%) |
Systolic BP (mm Hg) | 106 ± 14 | 137 ± 17 | 111 ± 18 | 121 ± 14 | 119 ± 18 |
Diastolic BP (mm Hg) | 63 ± 10 | 73 ± 15 | 66 ± 11 | 68 ± 10 | 67 ± 11 |
Vendor 1 heart rate (beats/min) | 70 ± 16 | 68 ± 8 | 63 ± 15 | 65 ± 11 | 66 ± 12 |
Vendor 2 heart rate (beats/min) | 69 ± 16 | 68 ± 8 | 68 ± 16 | 65 ± 11 | 67 ± 12 |
Coronary artery disease | 100 | 40 | 50 | 0 | 38 |
Hypertension | 50 | 100 | 50 | 0 | 40 |
Diabetes mellitus | 10 | 30 | 10 | 0 | 10 |
Tobacco | 70 | 20 | 20 | 0 | 22 |
Vendor 1 LVEF (%) | 49 ± 13 | 56 ± 11 | 30 ± 10 | 64 ± 5 | 53 ± 16 |
Vendor 2 LVEF (%) | 49 ± 12 | 57 ± 11 | 30 ± 10 | 66 ± 5 | 53 ± 16 |
Vendor 1: Intraobserver and Interobserver Analysis and ICC
There were high correlations for GLS for both intraobserver and interobserver variation (correlation coefficient = 0.99 and 0.98, respectively, P < .05). The ICCs were also high for intraobserver and interobserver variation (0.99 and 0.98, respectively). Bland-Altman plots demonstrated the following levels of agreement across a broad range of GLS: the bias for intraobserver measurements of GLS using vendor 1 was 0.2%, with a range in strain values of −1.5% to +1.9% (95% LOA), and the bias for interobserver measurements of GLS using vendor 1 was 0.1%, with a range in strain values of −2.4% to +2.7% (95% LOA) ( Table 2 ).
Correlation coefficient ( r ) | P | ICC | Bland-Altman bias (95% LOA) | |
---|---|---|---|---|
Vendor 1 | ||||
Intraobserver | 0.99 | <.0001 | 0.99 | 0.2 (−1.5 to 1.9) |
Interobserver | 0.98 | <.0001 | 0.98 | 0.1 (−2.4 to 2.7) |
Vendor 2 | ||||
Intraobserver | 0.97 | <.0001 | 0.97 | 0.1 (−3.1 to 3.3) |
Interobserver | 0.95 | <.0001 | 0.97 | −0.9 (−4.8 to 3.0) |
Vendor 1 vs vendor 2 | ||||
Same reader | 0.96 | <.0001 | 0.96 | −0.6 (−3.9 to 2.7) |
Different readers | 0.95 | <.0001 | 0.95 | −0.7 (−4.7 to 3.4) |
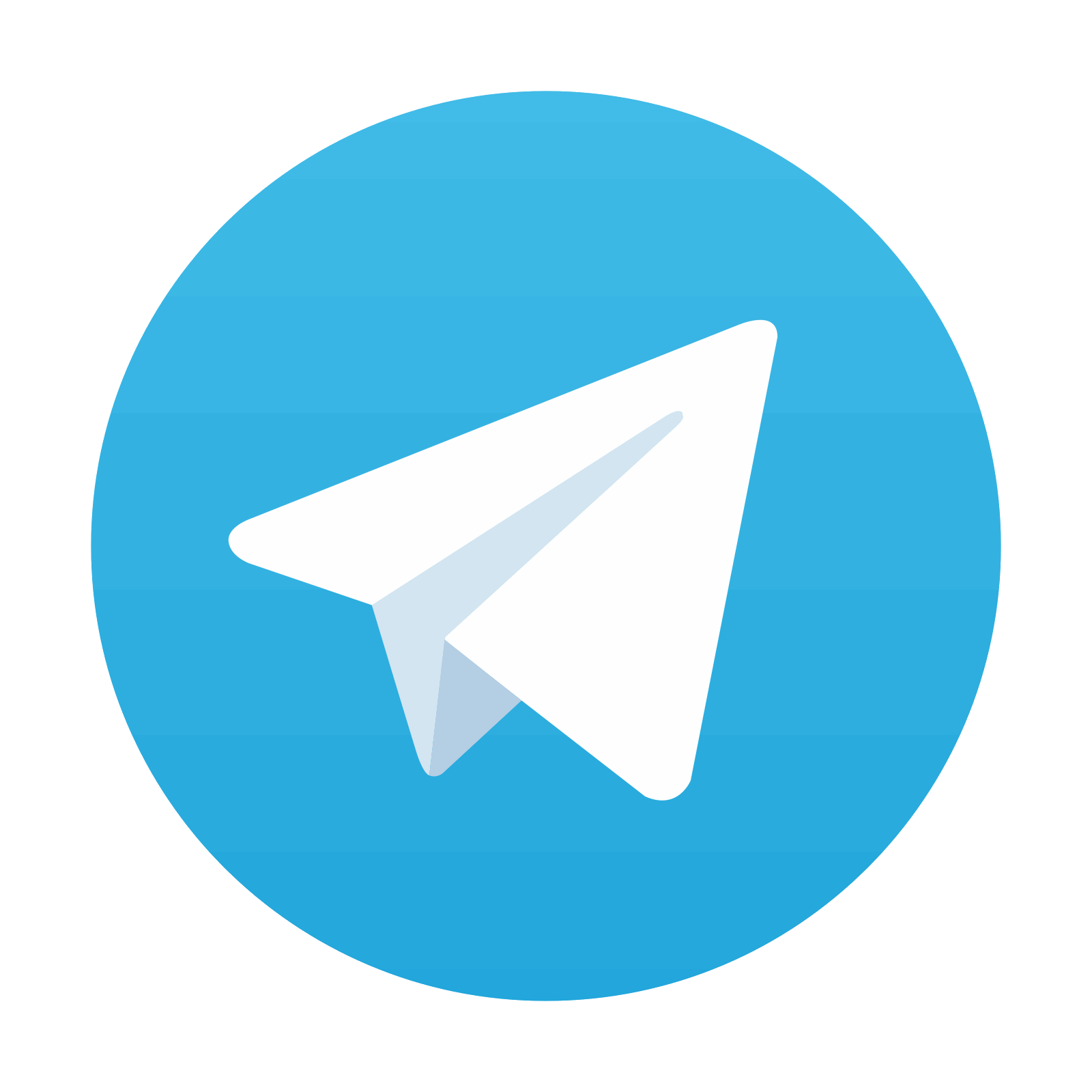
Stay updated, free articles. Join our Telegram channel

Full access? Get Clinical Tree
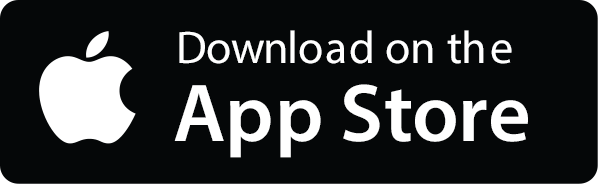
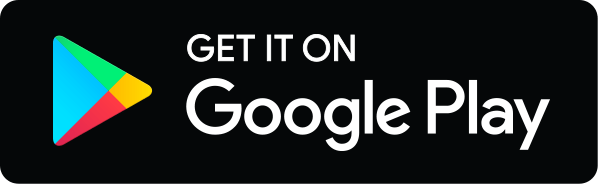
