Year of first publication
Device
Methods
Measurement site
Regional stiffness
1984a
Complior®
Mechanotransducer
Aorta, cf PWVb
1990a
Sphygmocor®
Tonometer
Aorta, cf PWVb
1991
WallTrack®
Echo-tracking
Aorta, cf PWVb
1994
QKD
ECG+
Aorta, cf PWVb
1997a
Cardiovascular Eng. Inc®
Tonometer
Aorta, cf PWVb
2002
Artlab®
Echo-tracking
Aorta, cf PWVb
2002
Ultrasound systems
Doppler probes
Aorta, cf PWVb
2002
Omron VP-1000®
Pressure cuffs
Aorta, ba PWVb
2007
CAVI-Vasera®
ECG + pressure cuffs
Aorta, ca PWVb
2008
Arteriograph®
Arm pressure cuff
Aorta, aa PWVb
2009
MRI, ArtFun®
MRI
Aorta, aa PWVb
2009
Vicorder®
Cuffs
Aorta, cf PWVb
2010
Mobil-O-Graph®
Arm pressure cuff
Aorta, cf PWVb
Local stiffness
1991
WallTrack®
Echo-tracking
CCAc, CFA, BA
1992
NIUS®
Echo-tracking
RA
2002
Artlab®
Echo-tracking
CCAc, CFA, BA
Various ultrasound system
Echography
CCAc, CFA, BA
2009
MRI, ArtFun®
Cine-MRI
AA, DA
Systemic stiffness
1989
Area method
Diastolic decay
1995
HDI PW CR-2000®
Modif. Windkessel
1997a
Cardiovascular Eng. Inc®
Tonometer/Doppler/echo
6.2.1.1 Regional Measurements of Arterial Stiffness
The measurement of pulse wave velocity (PWV) is generally accepted as the most simple, non-invasive, robust and reproducible method with which to determine arterial stiffness [3]. It is recommended by the 2013 ESH-ESC Guidelines for the Management of Hypertension [4]. Carotid-femoral PWV is a direct measurement, and it corresponds to the widely accepted propagative model of the arterial system. Measured along the aortic and aortoiliac pathway, it is the most clinically relevant, since the aorta and its first branches are what the left ventricle ‘sees’ and are thus responsible for most of the pathophysiological effects of arterial stiffness. Carotid-femoral PWV has been used in epidemiological studies demonstrating the predictive value of aortic stiffness for CV events [1, 2].
PWV is usually measured using the foot-to-foot velocity method from various waveforms. These are usually obtained transcutaneously at the right common carotid artery and the right femoral artery (i.e. ‘carotid-femoral’ PWV), and the time delay (Δt, or transit time) is measured between the feet of the two waveforms. The ‘foot’ of the wave is defined at the end of diastole, when the steep rise of the wavefront begins. The transit time is the time of travel of the ‘foot’ of the wave over a known distance. A variety of different waveforms can be used including pressure, distension and Doppler [3, 5]. The distance (D) covered by the waves is usually assimilated to the surface distance between the two recording sites, i.e. the common carotid artery (CCA) and the common femoral artery (CFA). The direct distance DD is (CFA to CCA). PWV is calculated as PWV = D (metres)/Δt (seconds). However, since the descending thoracic aorta is reached by the pressure wave at the time another pressure wave, originating from the same cardiac contraction, arrives at the carotid site, it has been recommended to calculate the distance between the suprasternal notch (SSN) and the common femoral artery (CFA) and to subtract from this distance the small length between carotid transducer and SSN. The so-called subtracted distance is (SSN to CFA) – (SSN to CCA). A recent consensus paper [6] stated that investigator should either use the subtracted distance or, best, measure the direct distance and apply a 0.8 coefficient, to take into account the different pathways of the pressure wave described above.
6.2.1.2 Local Determination of Arterial Stiffness
Local arterial stiffness of superficial arteries can be determined using ultrasound devices. Carotid stiffness may be of particular interest, since in that artery, atherosclerosis is frequent. All types of classical, bi-dimensional vascular ultrasound systems can be used in determining diameter at diastole and stroke changes in diameter, but most of them are limited in the precision of measurements because they generally use a video-image analysis. Echo-tracking devices were developed to measure diameter in end-diastole and stroke change in diameter with a very high precision. The two first devices were the Wall Track System® and the NIUS 02® (Table 6.1). These apparatus use the radio-frequency (RF) signal to obtain a precision six to ten times higher than with video-image systems, which are limited by the spatial resolution of pixel analysis.
At present, some researchers also measure local arterial stiffness of deep arteries like the aorta using cine magnetic resonance imaging (MRI). However, most of pathophysiological and pharmacological studies have used echo-tracking techniques. Most of these parameters required measurement of blood pressure. This should be local pressure, which is usually obtained by applanation tonometry of the vessel in question and calibration of the waveform to brachial mean and diastolic pressures obtained by integration of the brachial or radial waveform or automatic calculation using transfer function processing. All the superficial arteries are suitable for the geometrical investigation and particularly the common carotid, common femoral and brachial arteries.
6.2.1.3 Systemic Arterial Stiffness
Methods used for the non-invasive determination of systemic arterial stiffness are based on analogies with electrical models combining capacitance and resistance in series (Table 6.1). As such they rely on numerous theoretical approximations following direct measurement of one peripheral, and often distal, parameter. Their theoretical, technical and practical limitations that impact on their widespread application in the clinical setting have been discussed and compared with methods used for the non-invasive determination of regional stiffness [3]. Until now, they did not provide evidence, in a longitudinal study, that systemic arterial stiffness or systemic arterial compliance has independent predictive value for CV events.
6.2.2 How to Assess Central Blood Pressure
A large number of reviews have made recommendations for adequate measurements of central BP [3, 7–9]. Arterial pressure waveform should be analysed at the central level, i.e. the ascending aorta, since it represents the true load imposed to the heart, the brain and the kidney and more generally to central large artery walls. Table 6.2 details the various methods currently used for determining central BP [8]. The pressure waveform can be recorded non-invasively with a pencil-type probe incorporating a high-fidelity Millar strain gauge transducer (SPT-301, Millar Instruments). The most widely used approach is to perform radial artery tonometry and then apply a transfer function (Sphygmocor, AtCor, Sydney Australia) to calculate the aortic pressure waveform from the radial waveform (Table 6.2). Indeed, the radial artery is well supported by bony tissue, making optimal applanation easier to achieve. The algorithm used to apply the transfer function revealed to be robust for accurately determining central BP even during haemodynamic perturbations, including exercise, Valsalva manoeuvre, nitroglycerin, beta-adrenergic stimulation, angiotensin II and noradrenaline [9]. However, this procedure may underestimate central systolic BP at higher heart rates (e.g. above 100 bpm), and major error can be created by using inaccurate upper arm cuff BP values to calibrate the pressure waveforms. Careful attention to correctly measuring brachial cuff BP can help alleviate, but not entirely deal with this problem, particularly as there may be additional systolic BP amplification from the brachial to radial arterial beds [8, 9]. Despite these limitations, radial tonometry is popular since it is simple to perform and well tolerated.
Table 6.2
Device and methods used for determining central blood pressure and wave reflections
Year of first publication | Device | Method | Parameter | Measurement site |
---|---|---|---|---|
Central pressure waveform | ||||
1984 | Millar strain gauge®a | Tonometer, direct | cSBP, cPP, cAIx | Radial, carotid |
1990 | Sphygmocor®a | Tonometer, transfer function | cSBP, cPP, cAIx | Radial, carotid |
1997 | Cardiovascular Eng. Inc®a | Tonometer, cardiac echo, impedance | cSBP, cPP, cAIx, Zc, fW, bW | Radial, carotid |
2004 | Pulse Pen® | Tonometer, direct | cSBP, cPP, cAIx | Carotid |
Central SBP | ||||
2008 | Arteriograph® | Oscillometric | cSBP, cPP, cAIx | Brachial |
2009 | Omron HEM-9001A I® | Tonometer | cSBP, rAIx | Radial |
2010 | Mobil-O-Graph® | Oscillometric | cSBP, cPP, cAIx | Brachial |
A part from methods determining the pressure waveform at the central site, novel methods have been developed, which aim at determining the discrete value of central SBP instead of the whole pressure waveform at central site [3, 8] (Table 6.2). Estimation of central BP without a transfer function is possible from the second systolic peak (SBP2) on the radial or brachial pressure waveform. The radial method relies on accurate calibration using brachial cuff BP.
6.3 Prevalence
A prerequisite to a wide implementation of pulse wave velocity and central blood pressure measurements into clinical practice is the availability of normal and reference values based on a large population, established through standardisation of methodology for measurement.
6.3.1 Normal and Reference Values of Pulse Wave Velocity
These values have been established by The Reference Values for Arterial Measurements Collaboration in 16,867 subjects and patients originating from 13 different centres across eight European countries [10]. Of these, 11,092 individuals were free from overt CV disease, nondiabetic, and untreated by either antihypertensive or lipid-lowering drugs and constituted the reference value population, of which the subset with optimal/normal blood pressures (n = 1,455) is the normal value population. Subjects were categorised by age decade and further subdivided according to BP categories. Pulse wave velocity increases with age and BP category, the increase with age being more pronounced for higher BP categories and the increase with BP being more important for older subjects (Tables 6.3 and 6.4).
Table 6.3
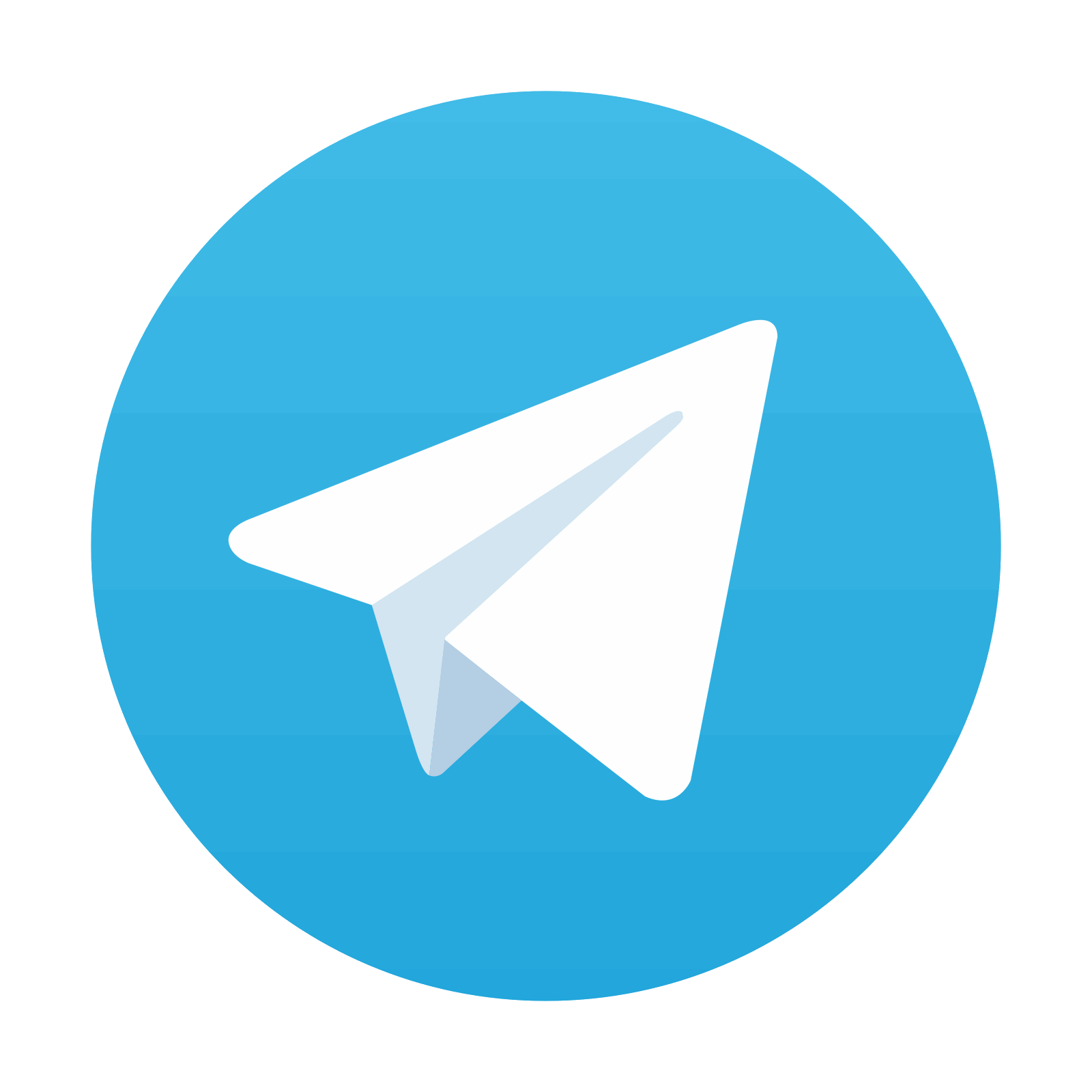
Distribution of pulse wave velocity (m/s) according to the age category in the normal values population (1,455 subjects)
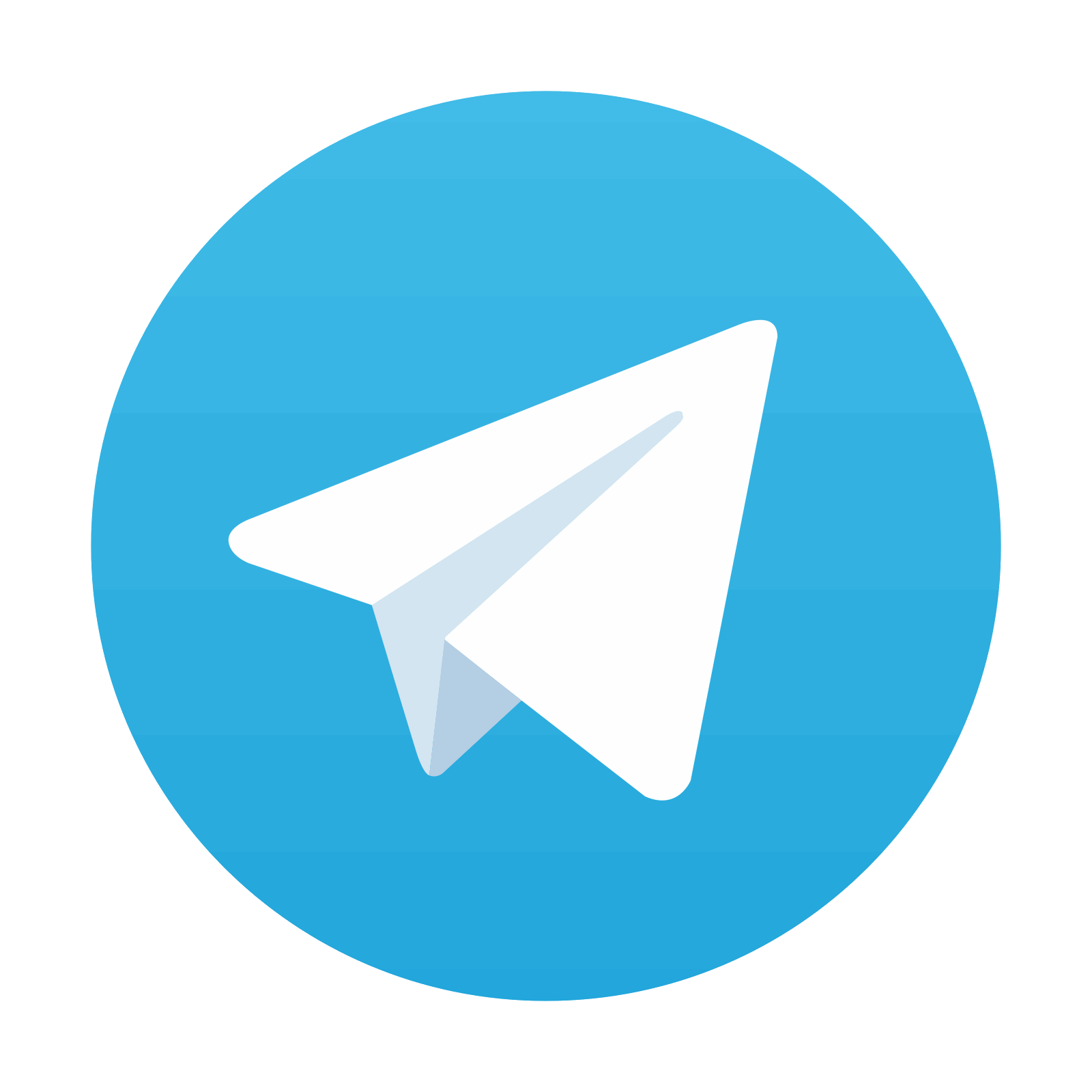
Stay updated, free articles. Join our Telegram channel

Full access? Get Clinical Tree
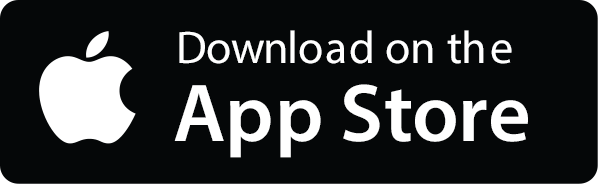
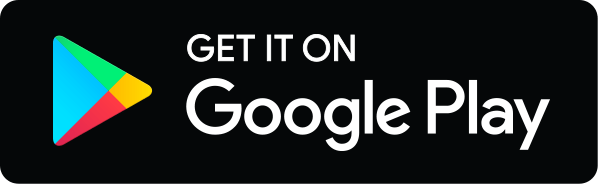
