(1)
Department of Cardiovascular, Neural and Metabolic Sciences, Istituto Auxologico Italiano, Milan, Italy
2.1 Arterial Stiffness and Cardiovascular Risk
Cardiovascular diseases are currently the main cause of death in developed countries. One of the main goals of Healthcare systems is, therefore, the prevention of cardiovascular diseases and the reduction in major cardiovascular events such as myocardial infarction and cerebral stroke.
Throughout the 20th century, the vascular disease was considered synonymous with atherosclerosis. Consequently, only the factors able to favor or inhibit the process of atherosclerosis were taken into account in cardiovascular prevention and the assessment of cardiovascular risk last century. Actually, the arterial system has always been considered to be made of inert “tubes”, which connect the left ventricle to peripheral tissues. These “tubes” assume clinical significance only when they tend to narrow (infarction) or break (bleeding).
Arteriosclerosis, namely the stiffening of large elastic arteries, was, therefore, considered insignificant in the assessment of cardiovascular risk. It was only at the beginning of the new millennium that several epidemiological studies started to show the predictive value of aortic stiffening (aortosclerosis, i.e., arteriosclerosis of the aorta) for cardiovascular diseases, over and above other traditional major risk factors. Below you will find some of the most important outcomes from the main epidemiological and clinical studies where aortic stiffening was assessed by measuring aortic pulse wave velocity (PWV), the velocity by which pulse wave travels along the aorta.
A higher relative risk of cardiovascular mortality was shown in hypertensive patients characterized by high aortic PWV values [1]. In this population, an increase in PWV by 5 m/s increases the relative risk of cardiovascular event as much as 10 years of aging.
Aortic stiffness has been shown to be an independent predictor of primary coronary events in patients with essential hypertension [2]. The risk of developing a coronary heart disease event rose with each tertile of PWV and remained significant after adjustment for Framingham risk score or all cardiovascular risk factors (Fig. 2.1). The increase in risk of coronary heart disease events with tertiles of PWV was particularly steep for patients traditionally considered at low risk.
Fig. 2.1
The observed incidence of coronary heart disease events parallels the incidence of coronary heart disease, which has been predicted from the Framingham risk score (FRS), except for the highest values of FRS. Pulse wave velocity (PWV) significantly (p = 0.002) predicted all cardiovascular events in patients considered at low risk, i.e., belonging to the first and second tertiles of the FRS, even after full adjustment for all cardiovascular risk factors [2]
A 1 m/s increase in aortic PWV was associated with a 7 % higher risk of a cardiovascular event for a 60-year-old man without other known cardiovascular risk factors [3].
Aortic stiffness was significantly associated with the risk of stroke death in patients with essential hypertension. In these patients, arterial stiffness, measured through PWV, predicted the occurrence of fatal stroke beyond the prediction provided by classic risk factors. PWV was significantly associated with a 72 % increase in stroke risk for each 4 m/s increase in PWV [4].
Aortic PWV was a significant predictor of cardiovascular complications, above and beyond 24-h mean arterial pressure and traditional risk factors, including sex, age, body mass index, current smoking and alcohol intake (Figs. 2.2 and 2.3) [5].
Fig. 2.2
Absolute risk associated with aortic pulse wave velocity (PWV) in women and men at different levels of mean arterial pressure in the office controlling for age, body mass index, current smoking, and alcohol intake. At any level of mean arterial pressure, the absolute risk of a composite cardiovascular outcome increased in relation to aortic PWV, more in women than in men [5]
Fig. 2.3
Relative hazard ratios for the composite cardiovascular end point by quintiles of the distribution of aortic pulse wave velocity (PWV) and office and 24-h pulse pressures adjusted for sex and age. The hazard ratios express the risk in each quintile vs. the average risk in the whole population [5]
More recently, data from the Framingham Heart Study [6] clearly showed that higher aortic stiffness assessed by PWV is associated with higher risk for a first cardiovascular event. Aortic PWV improves risk prediction when added to standard risk factors and may represent a valuable biomarker of cardiovascular disease risk in the community (Fig. 2.4).
Fig. 2.4
Kaplan-Meier plot of cumulative probability of a first major cardiovascular event. Participants were grouped according to quartiles of carotid-femoral (aortic) pulse wave velocity (PWV). When individuals in the highest (≥11.8 m/s) aortic PWV group were compared with those in the lowest (<7.8 m/s) aortic PWV group after adjustment for age, sex, and standard risk factors, individuals in the highest quartile had an adjusted Hazard Ratio of 3.4 (p = 0.008) Data from Framingham Heart Study [6]
Thus, at present, an increase in aortic PWV, expression of aortic stiffness (aortosclerosis), is considered to be an independent predictor of cardiovascular mortality. This implies that not only the phenomena of atherosclerosis but also the factors associated with arteriosclerosis, namely with the reduction in the viscoelastic properties of large arteries, must be taken into account in the assessment of cardiovascular risk.
2.2 Aortic Pulse Wave Velocity
Measurement of pulse wave velocity (PWV) represents the simplest way to measure the stiffness of a specific arterial segment, as it is non-invasive reproducible, and supported by considerable scientific literature.
The pulse wave is transmitted through the arterial vessels, and its speed is inversely related to the viscoelastic properties of the wall itself; the higher the velocity, the less elastic the wall.
The concept of pulse wave velocity is not always clear (it is not clear to many “experts” either). Therefore, let us clear up the most common misunderstanding:
pulse wave velocity is not blood flow velocity.
Blood flow velocity changes in the cardiac cycle and is on the order of cm/s (a mean blood velocity of 22 cm/s corresponds to <0.8 km/h, i.e., <0.5 mph) whereas pulse wave velocity is on the order of m/s, PWV values ranging from 4 to over 30 m/s, i.e., from 14 km/h (=9 mph) to over 108 km/h (=67 mph).
However, the term “velocity” evokes the image of something which moves and travels. This is the reason why, when speaking about velocity in the arterial system, red blood cells tend to be considered as a lot of red Ferrari racing cars whizzing down the aorta at high speed.
But … this is not pulse wave velocity.
To provide better understanding of pulse wave velocity, let us change the term to “pulse wave transmission”, which evokes something which is propagated from segment to segment, rather than something which moves and travels, just like the velocity at which the vibrations of violin or guitar strings are transmitted.
We must conceive pulse wave velocity as a shock wave.
To see what “shock wave propagation” means, let us imagine arterial circulation as a series of “units”, where each unit represents the stroke volume, that is, the amount of blood ejected from the heart at each systole. Each of these “unit” can be considered similar to the carriage of a train. Each systole represents a railway engine that arrives at the station and crashes into the carriages that are there, thereby generating a shock wave that is transmitted along the track (arterial system).
Another example which can help us to understand the meaning of the shock wave characterizing pulse wave velocity is the Newton’s cradle. This is a toy consisting of a number of steel pinball-sized balls hanging in a row from a rigid bar (Fig. 2.5).


Fig. 2.5
Newton’s cradle: example of shock wave velocity. If we take the first ball (“A”), raise it, and then let it fall again to hit the next ball (“B”), a shock wave is generated. As a result, the last ball (“Q”) almost immediately breaks away from the row. The time elapsed between the impact of the first ball (“A”) with the second one (“B”) and the time when the last ball (“Q”) breaks away from the row is a thousandth of a second and is unrelated to the speed at which the first ball hits the second one
Even in this case, we can consider our vascular system as a series of “units” (our balls), where each “unit” is correlated with the stroke volume, i.e., the amount of blood ejected from the heart at each systole. Each systole represents a “unit” (a ball) which is thrown against the others, generating a shock wave which is transmitted along the arterial system.
If we do not merely let the first ball fall against the second one, but rather push it with all our strength or let it fall from a higher position, we can see that the corresponding greater impact is not accompanied by a change in the latency period between the impact of the first ball with the second one and the time that the last ball takes to break away from the row. Shock wave velocity, therefore, is unrelated to the strength (pressure) exerted. Similarly, the relationship between pulse wave and blood pressure is secondary to the organic alterations caused by blood pressure on the structure of the arterial wall, that is, the elastin and collagen fibers ratio, but depends only partially on blood pressure values recorded at the time the test is carried out. However, some conditions characterized by high blood pressure values cause a state of strain and distensibility of the vascular wall, which affects its elastic capabilities and can influence pulse wave transmission. If we replace our metal balls with cotton or styrofoam balls, we can easily see that, in this case, with respect to the series of steel balls, the latency time between the impact of the first ball with the second one and the time the last ball breaks away from the series increases. This leads us to the concepts of vascular “stiffness” and “elasticity”.
2.2.1 Methodological Aspects
Technically, it is possible to assess pulse wave velocity by simultaneously recording the pressure waveform at two different sites in the arterial tree: a proximal site and a distal, peripheral one. This enables the time delay between pressure waveforms recorded in the distal segment with respect to the proximal one to be calculated.
We know that velocity is equal to displacement/time. As a consequence, pulse wave velocity is calculated using the following formula:
where ΔT represents the time delay of the distal pulse pressure waveform with respect to the proximal one.

The possibility of establishing a correlation between PWV and artery distensibility is based on the calculation of the speed of transmission of transverse elastic waves. According to the law for transmission of transverse waves, as applied for the first time by Moens (1878) and later modified by Bramwell and Hill [7], this concept has been formalized in a mathematical model that connects the elasticity of the arterial wall with the inverse of the square of PWV.
where distensibility is defined as the percentage variation in diameter for each increase in blood pressure of 1 mmHg. Therefore, according to this formula, for each increase in blood pressure of 1 mmHg:

PWV of 4 m/s corresponds to distensibility of 0.80 %
PWV of 6 m/s corresponds to distensibility of 0.35 %
PWV of 8 m/s corresponds to distensibility of 0.20 %
PWV of 10 m/s corresponds to distensibility of 0.13 %
PWV of 12 m/s corresponds to distensibility of 0.09 %
PWV of 14 m/s corresponds to distensibility of 0.06 %
PWV of 16 m/s corresponds to distensibility of 0.05 %
Carotid–femoral pulse wave velocity (aortic PWV) is considered the gold-standard method for non-invasive assessing of arterial stiffness [8].
The proximal transducer is placed on the carotid artery and the distal transducer on the femoral artery. In this way, the PWV along the aorta is measured. Therefore, the carotid–femoral PWV reflects the viscoelastic properties of the aorta.
Carotid–femoral PWV measurement can be easily carried out and two different methods are possible:
- 1.
At one time (Figs. 2.6 and 2.7), two transducers are simultaneously used (tonometers, ultrasound probes, oscillometers, mechanotransducers):
Fig. 2.6
Carotid-femoral pulse wave velocity assessed all at once: carotid and femoral pressure waveforms are recorded simultaneously
Fig. 2.7
When carotid-femoral pulse wave velocity is assessed all at once, carotid and femoral may be recorded simultaneously both by one expert operator (upper panels) or by two operators (lower panels). Tests performed using a wireless PulsePen® device are shown in the images on the right
The first transducer records the proximal pulse wave curve (the proximal transducer is placed on the common carotid artery, considered as central detection site).
The second transducer records the pulse wave curve simultaneously in the peripheral artery (femoral, brachial, radial, posterior tibial arteries, etc.).
This method enables the calculation of the time delay between the distal pulse wave curve and the proximal pulse wave curve and it is being used by several devices on the market:
The Complior Analyse® (Alam Medical, Vincennes, France) uses two piezoelectric sensors, which record pressure waveforms
The PulsePen® ETT (DiaTecne srl, Milan, Italy), uses two tonometers. This model also allows the recording of central aortic blood pressure and pulse wave analysis
The PulsePenLab® (DiaTecne srl, Milan, Italy), a model derived from the PulsePen® and targeted at the study of small animals and newborns
The SphygmoCor® XCEL PWV Measurement System (AtCor Medical Pty Ltd, Sydney, Australia) measures carotid pulse pressure with a tonometer, while the femoral pulse is measured through pulsations in a cuff placed around the thigh
- 2.
At two times (Figs. 2.8 and 2.9), just one transducer combined with electrocardiographic tracing is used. In this way, PWV can be assessed at two times, separated by a short interval, taking the R wave of the QRS complex of the electrocardiogram (ECG) as a reference point. ΔT will be given by the difference between the time delay of the distal (femoral, brachial, radial, posterior tibial arteries, etc.) pulse wave with relation to the R wave belonging to the QRS complex of the ECG and the time delay of the proximal pulse wave in relation to the R wave of the QRS complex of the ECG.
Fig. 2.8
Carotid-femoral pulse wave velocity assessed at two times. (I) Upper panel: carotid pressure waveform recording and assessment of the time delay of the waveform with respect to the R wave of the QRS complex of the ECG recording. (II) Lower panel: pressure waveform recording in the femoral artery and assessment of the time delay of the waveform with respect to the R wave of the QRS complex of the ECG
Fig. 2.9
When carotid-femoral pulse wave velocity is assessed at two times, firstly pulse pressure wave is recorded in carotid artery simultaneously with ECG (upper panels); secondly, pulse pressure wave is simultaneously recorded with ECG in femoral artery (lower panels). Tests performed using a PulsePen® device are shown in the images on the right. It is worth noting that, thanks to the high sensitivity of the PulsePen® tonometer, the femoral pressure waveform can be recorded by placing the probe on the patients’ clothes, so that they are not required to undress
This second method provides outcomes that are exactly superimposable on the first method. However, being carried out at two times, significant variation in heart rate or systolic blood pressure between the recording of the proximal and distal pulse waveform curves must not occur. This is an important reason why blood pressure and heart rate must be recorded by means of a traditional validated sphygmomanometer at the same time of both proximal and distal pulse wave acquisition. Measurements of PWV where the difference between the mean values of the heart period (defined by the R–R interval of the ECG) recorded in carotid and peripheral arteries is over 10 % are not considered reliable.
This method is used by two devices; they both use a tonometer to record the pulse wave:
The PulsePen® ET (DiaTecne srl, Milan)
The SphygmoCor® CPV System (AtCor Medical Pty. Ltd., Sydney)
2.2.1.1 How to Measure the Distance
Pulse wave velocity is given by the ratio between the distance between two artery points and the delay in the peripheral artery pressure wave with respect to the proximal pressure wave. However, how can we measure this distance?
To measure distances, it is possible to use a rigid bar, which must be graduated, equipped with diastimeters at the ends, similar to the one used to measure a newborn’s height. Such an instrument can be useful especially when measuring the distance in overweight or pregnant subjects. Actually, in these cases, if we used a classic flexible tape measure, we would run the risk of overestimating the distance; it is very important to take the tape measure distance in a straight line.
As for carotid–femoral PWV, direct measurement of the distance between the carotid and femoral artery pressure wave has been used for years (Fig. 2.10a).


Fig. 2.10
Main methods to evaluate carotid-femoral distance in assessing PWV. (a) “Direct” measurement of carotid-femoral distance. (b) Measurement of carotid-femoral distance with the “subtractive” method. Firstly, the suprasternal notch is taken as a reference point. Secondly, the distance between the suprasternal notch and the femoral artery is measured. Thirdly, from this measure, the distance between the suprasternal notch and the carotid sampling site is subtracted
Distance can also be measured with the so-called subtractive method (Figs. 2.10b and 2.11), which is more accurate. This method seems to be better correlated with the real anatomical distance between the two analyzed arterial sites.


Fig. 2.11
The “subtractive method” is based on the knowledge that, while the pressure wave reaches the carotid pressure landmark (distance a → c in the figure), it would cover the same distance in the aorta (distance a → x). This path coincides with segment “sx”, which corresponds to segment “cs”, i.e., the distance between the suprasternal notch and the carotid sampling site
Distance is a basic parameter for the assessment of PWV, and its importance should not be underestimated; for example, let us consider a subject with a delay of 100 ms in the femoral artery pressure wave in relation to the carotid pressure wave, with carotid-to-femoral distance of 650 mm, suprasternal notch-to-carotid distance of 100 mm, and suprasternal notch-to-femoral distance of 560 mm. If PWV is, calculated using the “direct” method, it is 6.5 m/s [PWV = 650/100]. On the contrary, if PWV is calculated using the “subtractive” method, it is 4.6 m/s [PWV = (560 − 100)/100]. In this case, PWV is 32 % greater using the “direct” method than the “subtractive” method.
A newly published expert consensus document on the measurement of aortic stiffness [9] provides advice on the use of 80 % of the direct carotid-to-femoral tape measure distance as new standard for daily practice:


A more accurate, simple and acceptable modification of this formula, taking into account age, was proposed, too. This tested formula adjusts this distance for age by adding 1 mm/year for an age above 50 and subtracting 1 mm/year for an age below 50, respectively:


I always suggest considering all the three measures: carotid-to-femoral, suprasternal notch-to-carotid, and suprasternal-to-femoral. In this way, you can always compare your own findings with those reported in literature, regardless of the method used by the authors.
When you read scientific papers or articles concerning PWV, you should always pay attention to the method used to define distance in the calculation of PWV. This is the reason why you are totally bewildered when scientific publications and official guidelines express cutoff values for PWV without specifying the method used to obtain them.
2.2.1.2 How to Measure the Transit Time Delay
The foot of the pulse pressure wave represents the landmark for the calculation of the time delay of the pulse wave, which is the time delay between two waves on a simultaneous recording (Fig. 2.12a) or the time delay with respect to the R wave of the ECG recorded at two intervals (Fig. 2.12b). This is the method used by the PulsePen® (intersecting interpolation algorithm), the Complior Analyse® and the SphygmoCor® (intersecting tangent algorithm), providing PWV values that are superimposable between them. On the contrary, the old model Complior SP® used an algorithm based on a derivative of the change in arterial wall motion, secondary to pulse pressure. The Complior SP® supplies PWV values inferior to the PulsePen® and the SphygmoCor®.


Fig. 2.12
(a) Pulse waves foot-to-foot transit time assessment using the intersecting interpolation algorithm. Measurement of the time delay of the peripheral pressure waveform (bottom) in relation to the central pressure waveform (top). The foot of the pressure waveform curve is estimated by the intersection of the horizontal line tangent to the lowest point of the pressure waveform (line “z”) with the prolongation of the straight line that minimizes the standard deviation of the points building up the initial protosystolic rapid ascending phase of the pressure waveform curve (line “y”). (b) Measurement of the time delay of the pressure waveform (top) in relation to the R wave of the ECG complex (bottom). The latter occurs when pulse wave velocity is recorded at two times
Several formulas for converting one measurement system into another have been suggested. The most commonly used method suggests conversion of the carotid–femoral pulse transit time (TT), according to the following formula:


2.2.1.3 Pulse Wave Velocity in Subjects with Arrhythmias
Is the PWV value recorded in a subject with arrhythmias or with atrial fibrillation reliable? What are the rules to follow? This is one of the most frequent questions that operators using this method in clinical practice ask themselves.
Arrhythmias and atrial fibrillation, in particular, are probably the only clinical conditions where central and peripheral pressure waveforms are strongly recommended to be recorded simultaneously to define PWV.
Yet, PWV, recorded at two times in subjects with atrial fibrillation, may be considered reliable under the following conditions:
In subjects where fibrillation is rather regular and with no significant changes in the R-R interval on ECG. Measurements where the standard deviation of the R-R intervals is less than 20 % of the mean value can be accepted.
If PWV is to be recorded at two times, it is always important to verify that the difference between the mean value of the cardiac period of the R′–R′ interval recorded in the carotid artery and that recorded in the peripheral artery is less than 10 %.
If the previous conditions are fulfilled, it is advisable to carry out at least two measurements in subjects with atrial fibrillation and to use the mean of the recorded measurements.
Assessment of PWV is easier in subjects with premature atrial or ventricular complexes.
In fact, it is strongly recommended that the analysis of the foot of the pulse pressure waveform corresponding to the extrasystole and to the next one should be excluded when assessing PWV.
On the contrary, as for pulse wave analysis, it is advisable to exclude not only the pressure curve generated by the extrasystole but also the previous and the following ones.
2.2.1.4 Sampling Rate
Sampling rate is a very important parameter for a device that aims to accurately calculate PWV and accurately define pulse wave analysis. Figures 2.13 and 2.14 illustrate what the pressure waveform and the ECG would look like if there were no interpolation process and the signal was recorded point by point at different sample rates. The low sensitivity of the recordings with a low sampling rate is evident. However, the processes of interpolation ensure a phenomenon of cosmetics of the recordings, and the waves acquired at low sampling rate often appear cleaner and more linear than waves acquired point-to-point, without the aid of an interpolation process. At low sampling rates, many details of the pulse waveform are lost. Moreover, the definition of the peak of the R wave in the QRS complex is obviously inaccurate, as well as the definition of the PWV.



Fig. 2.13
Importance of sampling rate in defining the pulse pressure waveform and the parameters required for calculating the pulse wave velocity (heart rate: 60 beats/min). (a) Upper panel: pulse wave and ECG signal are acquired with 1 kHz sampling rate (each signal every 1 ms). This sampling rate is used by the PulsePen®, the PulsePenLab®, and the Complior Analyse® devices. (b) Medium panel: pulse wave and ECG signal are acquired with 256 Hz sampling rate (each signal every 3.9 ms). This sampling rate is used by the SphygmoCor® XCEL device. (c) Lower panel: pulse wave and ECG signal are acquired with 128 Hz sampling rate (each signal every 7.8 ms). This sampling rate is used by the SphygmoCor® device

Fig. 2.14
(a) ECG tracing simultaneously recorded by SphygmoCor®, characterized by a 128 Hz sampling rate and (b) by PulsePen®, characterized by a 1 kHz sampling rate. Only single signals acquired during recording are shown, without interpolation
The ARTERY Society Guidelines for a standard methodology assessing PWV, suggested a sampling rate of 1 kHz (=1000 Hz), with a minimum recording time of 10 cardiac cycles. At present, this recommended sampling rate is used by both the Complior Analyse® system and the PulsePen® system. On the contrary, the SphygmoCor® system acquires signals with a sampling rate of 128 Hz or 256 Hz (XCEL system).
2.2.1.5 Aortic Distensibility and PWV Evaluated by MRI
Aortic PWV can also be measured by Magnetic Resonance Imaging (MRI). This procedure allows the user to select two regions of interest along the aorta, recording a flow-time curve (Fig. 2.15). As a matter of fact, PWV can be given by the ratio of the distance between two arterial points and the time delay between the two aortic flow curves (Fig. 2.16) [10]. MRI can also measure PWV at a single aortic location (ascending aorta, aortic arch, or descending aorta), providing useful hemodynamic information in different vascular diseases. However, the evaluation of the aortic blood flows by MRI appears to be very general and, consequently, the acquisition of PWV inaccurate (Figs. 2.17 and 2.18). This is the reason why MRI cannot be considered as a standard reference method, but it is a reliable procedure for the segmental evaluation of arterial compliance as it permits an accurate measurement of the variation of the vascular diameter during the cardiac cycle.





Fig. 2.15
Assessment of pulse wave velocity (PWV) by magnetic resonance imaging (MRI). In this case, three regions of interest (ROIs) have been considered. Sagittal scout MRI showing the aortic arch and the descending aorta. Two sections separated by a fixed distance of 15 cm are positioned perpendicularly to the thoracic aorta determining the three ROIs. MRI Proximal-PWV is determined with distance d 1, and the MRI Distal-PWV is determined with distance d 2; MRI Thoracic-PWV is determined by using the total arterial length (d 1 + d 2)

Fig. 2.16
Example of two normalized blood velocity-time curves generated from two intra-aortic ROIs (ROI 1 and 3). The curves are scaled to represent the same peak velocity value. Time-delay calculation is based on superimposition and minimization of the square deviation between waveforms

Fig. 2.17
Large spread of values in the data set acquired using magnetic resonance imaging (MRI) in the recording of aortic blood velocity-time curves. The figure highlights the curves corresponding to the mean (central thick curve), to the standard deviation (SD), and to 2SD of the data acquired

Fig. 2.18
Aortic blood velocity-time curves recorded by magnetic resonance imaging (MRI). (a) Curve of the blood flows as it is shown by the test. The curve is the result of the interpolation of the mean of the single points acquired. (b) Mean values of the single points acquired without interpolation. (c) A detail of the proto-meso-systolic phase of the picture shown above
2.2.2 Factors Affecting Pulse Wave Velocity
PWV depends on structural alterations and transient functional changes in the arterial wall. (Fig. 2.19 and Fig. 2.20).



Fig. 2.19
Structural and functional factors affecting pulse wave velocity in the aorta and large elastic arteries. The thickness of the arrow shows how much every single factor affects pulse wave velocity

Fig. 2.20
Structural and functional factors affecting pulse wave velocity in muscular arteries. The thickness of the arrow shows how much every single factor affects pulse wave velocity
2.2.2.1 Structural Factors
Structural alterations are stable and due to the change in the elastin and collagen fiber ratio in the arterial wall.
An increase in PWV with age is well established. Aging is characterized by generalized increase in arterial wall stiffness related to structural alterations. The aging process causes histological alterations in the arterial wall, and the degeneration of elastin fibers is accompanied by a boost in collagen fibers. Increased elastase activity and reduced elastin synthesis cause thinning and breakage of elastin fibers, and the result is a decrease in the elastin and collagen ratio. It was calculated (Faber and Moller-Hou 1954) that in the age range of 20–80 years, the percentage, in dry weight, of elastin fibers in the thoracic aorta decreases from 32 to 20 % whereas the percentage of collagen fibers increases from 21 to 32 %. However, the relationship between age and PWV is not linear. PWV values change insignificantly in the first decades of life then they tend to increase as age advances, by an average of 0.07 m/s per year from 45 to 65 years and of 0.2 m/s per year after 65 years.
As a matter of fact, it is well known that age-relating changes in the arterial structure reflect general aging on the whole body. In order to totally understand the process leading to the loss of elasticity of large elastic arteries, just think of the physiological loss of elasticity of the skin in the elderly. Try and pinch the skin on a forearm of elderly people: the skin will tend to remain in the pinched position and it will take a long time to return to normal. On the contrary, what a different reaction in the skin of children or young adults! The elasticity of these cutaneous tissues allows the skin to go back to its normal position quickly. As the skin loses its original elasticity in the elderly, so the aorta and large arteries tend to lose their elasticity and become stiffer with aging (arteriosclerosis process).
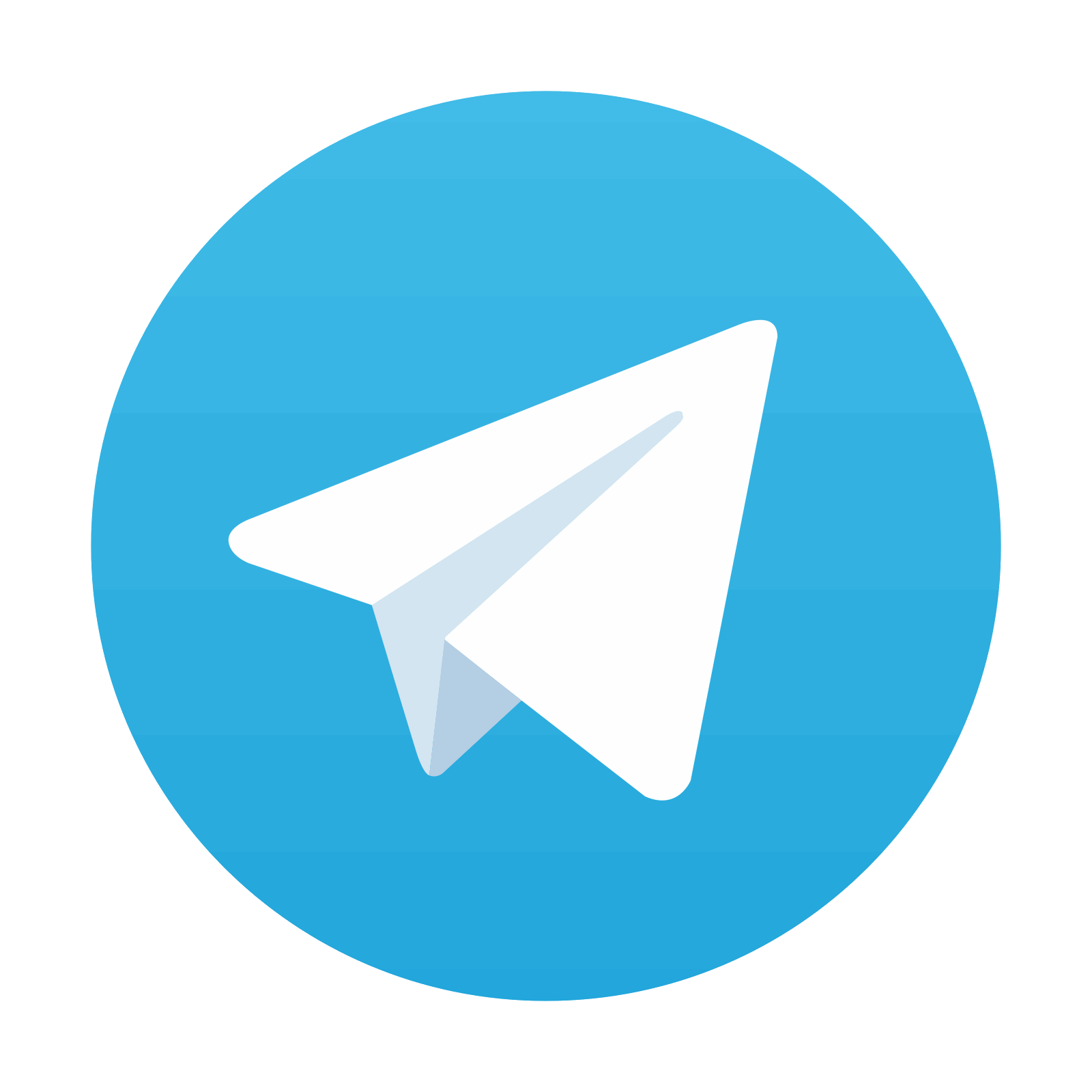
Stay updated, free articles. Join our Telegram channel

Full access? Get Clinical Tree
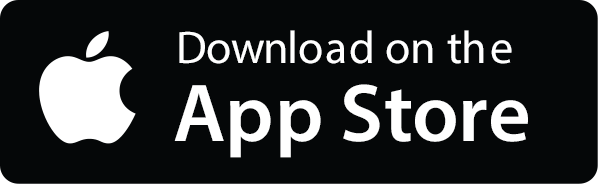
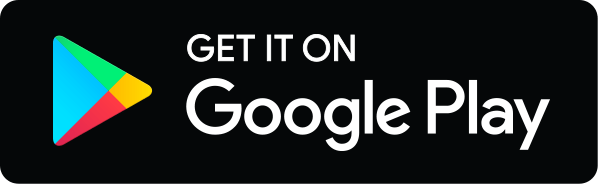
