Chapter 6 Pulmonary ventilation



Breathing consists of rhythmic changes in lung volume brought about by the medullary respiratory neurones described in Chapter 5. Several muscle groups are involved in effecting the change in lung volume. First, muscles of the pharynx and larynx control upper airway resistance; secondly the diaphragm, ribcage, spine and neck muscles bring about inspiration; and finally, muscles of the abdominal wall, ribcage and spine are used when active expiration is required. Many of these muscle groups have common origins and attachments such that their activity is complex and dependent both on each other and many non-respiratory factors including posture, locomotion and voluntary activity.
Upper Airway Muscles
During inspiration through the nose, the pressure in the pharynx must fall below atmospheric by an amount equal to the product of inspiratory gas flow rate and the flow resistance afforded by the nose (see Figure 4.1). This development of only a few kilopascals of sub-atmospheric pressure in the pharynx tends to cause the pharynx to collapse.
Pharyngeal obstruction in response to these pres-sure changes during inspiration is opposed by reflex contraction of pharyngeal dilator muscles during inspiration.1 The afferent side of the reflex arises from mechanoreceptors in the pharynx and larynx. These pressure receptors respond in a graded manner to subatmospheric pressure, and have myelinated afferent fibres to facilitate a rapid response.2,3 Based on the observation that the pharyngeal dilator reflex is less active during sleep (page 270) the reflex pathway is believed to involve higher centres of the brain.4 Nevertheless, the reflex is extremely rapid with both genioglossus and tensor palati electromyographic (EMG) activity increasing less than 50 ms after a negative pressure is applied to the pharynx. This compares with a reaction time for voluntary tongue movements of 190 ms.2 The efferent side of the reflex involves most of the pharyngeal dilator muscles, which display tonic contraction and/or phasic inspiratory activity. Airway diameters are well maintained down to pressures of 1.5 kPa (15 cmH2O) below atmospheric, during active but not passive breathing manoeuvres.5 Pulmonary slowly adapting stretch receptors (page 67) may also be involved in the reflex as the activity of all pharyngeal dilator muscles is inhibited by lung inflation.6
There is no significant narrowing of the airway when changing from the erect to the supine posture in the normal subject.7 Genioglossus EMG activity is increased by 34% in the supine position, presumably to counteract the effect of gravity on the tongue.8 Anatomical considerations suggest that patency of the nasopharynx in the supine position is maintained by tensor palati, palatoglossus and palatopharyngeus, and tonic but not phasic respiratory activity has been detected in levator palati.9 The soft palate tends to fall back against the posterior pharyngeal wall in the supine position without contraction of these muscles.
Failure of the various mechanisms that preserve pharyngeal airway patency may occur in sleep, hypoxia or anaesthesia; their occurrence and prevention are discussed in Chapters 16 and 22.
Laryngeal control of airway resistance. During quiet breathing, movement of the vocal folds is used as a choke for fine control of airway resistance. On inspiration, phasic activity of the posterior cricoarytenoid muscles, acting by rotating the arytenoid cartilages, abducts the vocal cords to minimise resistance.10 A greater effect occurs during expiration, when phasic electrical activity in thyroarytenoid muscles indicates adduction of the vocal cords,13 and therefore an increase in resistance. This may help to prevent collapse of the lower airways (page 49).
Respiratory Muscles of the Trunk
The Diaphragm
The diaphragm is a membranous muscle separating the abdominal cavity and chest, and in adults has a total surface area11 of approximately 900 cm2. It is the most important inspiratory muscle, with motor innervation solely from the phrenic nerves (C3, 4, 5). In comparison with other skeletal muscles, the diaphragm is extremely active. Muscle fibres within the diaphragm can reduce their length by up to 40% between residual volume and total lung capacity,11 and spend 45% of each day contracting, compared with only 14% for the soleus muscle.12 The diaphragm has considerable reserve of function, and unilateral phrenic block causes little decrement of overall ventilatory capacity. Despite the importance of the diaphragm to respiration, bilateral phrenic interruption is still compatible with good ventilatory function.
Mechanics of diaphragmatic function. The origins of the crural part of the diaphragm are the lumbar vertebrae and the arcuate ligaments, whilst the costal parts arise from the lower ribs and xiphisternum. Both parts are inserted into the central tendon. Recent studies of human subjects using MRI scans, illustrated in Figure 6.1, have enabled the in vivo actions of the diaphragm to be better defined.11,13,14 Under normal circumstances, a zone of apposition exists around the outside of the diaphragm where it is in direct contact with the internal aspect of the ribcage, with no lung in between, but the parietal pleura still allowing free movement of the diaphragm. At upright FRC in humans, approximately 55% of the diaphragm surface area is in the zone of apposition.11
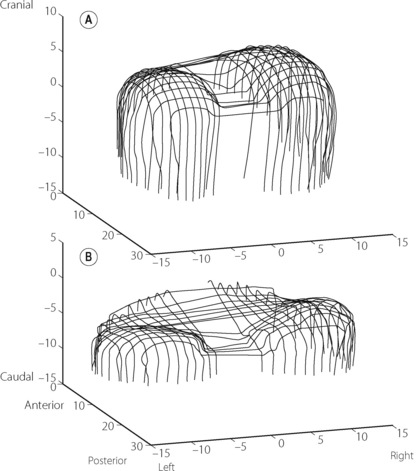
Fig. 6.1 Three-dimensional reconstructions of the human diaphragm at functional residual capacity using fast computed tomography scanning (dimensions in cm). (A) Normal subject showing extensive zone of apposition and normal curvature of the diaphragm domes. (B) Patient with hyperinflated chest as a result of chronic obstructive pulmonary disease (page 411). Note the reduced zone of apposition and the flattened diaphragm domes.
(After reference 14 by permission of the authors and the publishers of American Journal of Respiratory and Critical Care Medicine.)
There are many ways by which diaphragm contraction may bring about an increase in lung volume,15 and these are illustrated schematically in Figure 6.2. These may be considered using a ‘piston in a cylinder’ analogy, the trunk representing the cylinder and the diaphragm the piston (Figure 6.2A). Figure 6.2B illustrates the first possible mechanism, involving downward movement of the diaphragm simply by shortening the zone of apposition around the whole cylinder and leaving the dome shape unchanged. This is a pure ‘piston-like’ action and has the advantage of very efficient conversion of diaphragm muscle fibre shortening into changes in lung volume. Figure 6.2C illustrates ‘non-piston-like’ behaviour in which the zone of apposition remains unchanged but an increase in the tension of the diaphragm dome reduces the curvature, so expanding the lung. This is likely to be less efficient than piston-like behaviour because much of the muscle tension developed simply opposes the opposite side of the diaphragm rather than moving the diaphragm downwards, such that in theory, when the diaphragm becomes flat, further contraction will have no effect on lung volume. Finally, Figure 6.2D incorporates both types of behaviour already described but also now includes expansion of the lower ribcage (known as ‘piston in an expanding cylinder’) that occurs with diaphragmatic contraction, particularly in the supine position, and so represents a simple description of the in vivo situation.
In the supine position, diaphragm action is a combination of all the above mechanisms as well as a change in shape involving a tilting and flattening of the diaphragm in the antero-posterior direction.11
Ribcage Muscles16
As already described, the ribcage may be regarded as a cylinder with length governed primarily by the diaphragm and secondarily by flexion and extension of the spine. The cross-sectional area of the cylinder is governed by movement of the ribs. This movement involves mainly rotation of the neck of the rib about the axis of the costovertebral joints, and their shape is such that elevation of the ribs in this way increases both the lateral and anteroposterior diameter of the ribcage. Elevation of the ribs by the intercostal muscles tends to result in a ‘bucket handle’ action, whilst elevation of the anterior ribcage by, for example, the sternomastoid muscle elevating the sternum results in a ‘pump-handle’ type of movement. These two actions tend to occur together, and depend also on other requirements such as posture and upper limb movements. Upper ribs are inserted into the sternum and do not necessarily behave in quite the same way as the lower ‘floating’ ribs, which are inserted into the more flexible costal cartilage.
The intercostal muscles are divided into the external group, fibres of which run in a caudal–ventral direction from their upper rib and are deficient anteriorly, and the less powerful internal group which have fibres running caudal–dorsal from their upper rib and are deficient posteriorly. Internal intercostal muscles of the upper ribcage become thicker anteriorly where they are known as the parasternal intercostal muscles. In 1749, mechanical considerations led Hamberger to suggest that the external intercostals were primarily inspiratory, and the internal intercostals primarily expiratory.17 Though an oversimplification,16 this has generally been confirmed by electromyography. The parasternal portion of the internal intercostals are inspiratory in both humans and animals, and the inspiratory activity of external intercostals, though minimal during quiet breathing, becomes increasingly important during stimulated breathing. Posture plays an important role in intercostal activity in humans. For example, during the rather extreme postural challenge of rotating the trunk, which changes the mechanical properties of the ribs, the respiratory activity of internal and external intercostals is reversed with internal intercostals becoming expiratory and vice versa.18
Scalene muscles are active in inspiration during quiet breathing in humans19 particularly when upright. Their role is to elevate the ribcage and this counteracts the tendency of the diaphragm to cause inward displacement of the upper ribs. Innervation is from C1 to C5.
Integration of Respiratory Muscle Activity
Breathing
Figure 6.3 shows the radiographic appearance of the ribcage at residual volume, the normal expiratory level and at maximal inspiration, and illustrates the enormous range of movement within the semi-rigid ribcage. Expiration normally proceeds passively to the functional residual capacity (FRC), which may be considered as the equilibrium position governed by the balance of elastic forces, unless modified by residual end-expiratory tone in certain muscle groups. Inspiration is the active phase, entering the inspiratory capacity but normally leaving a substantial volume unused (the inspiratory reserve volume). Similarly, there is a substantial volume (the expiratory reserve volume) between FRC and the residual volume (see Figure 3.9). By voluntary effort it is possible to effect a satisfactory tidal exchange anywhere within the vital capacity, but the work of breathing is minimal at FRC.
Although we tend to think of the respiratory muscles individually, it is important to remember that they act together in an extraordinarily complex interaction that is influenced by factors including posture, minute volume, respiratory load, disease and anaesthesia. Figure 6.4 illustrates some features of the interaction.18,20
Inspiration. In Figure 6.4 it can be seen that the ribcage inspiratory muscles (external intercostals and scalenes) and diaphragm act in parallel to inflate the lungs, with posture affecting which muscle group is dominant (see below). In either position, diaphragm activity alone results in a widening of the lower ribcage and an indrawing of the upper ribcage (often seen with spontaneous respiration during general anaesthesia), which must be countered by the intercostal and neck muscles contracting simultaneously.
Separation of Volume Contribution of Ribcage and Abdomen
Konno & Mead originally proposed that the separate contributions to tidal volume of changes in ribcage (RC) and abdominal (AB) compartments could be measured.22 Essentially similar results may be obtained by measuring either anteroposterior distance (magnetometers), circumference (strain gauge) or cross-sectional area (respiratory inductance plethysmography, RIP23
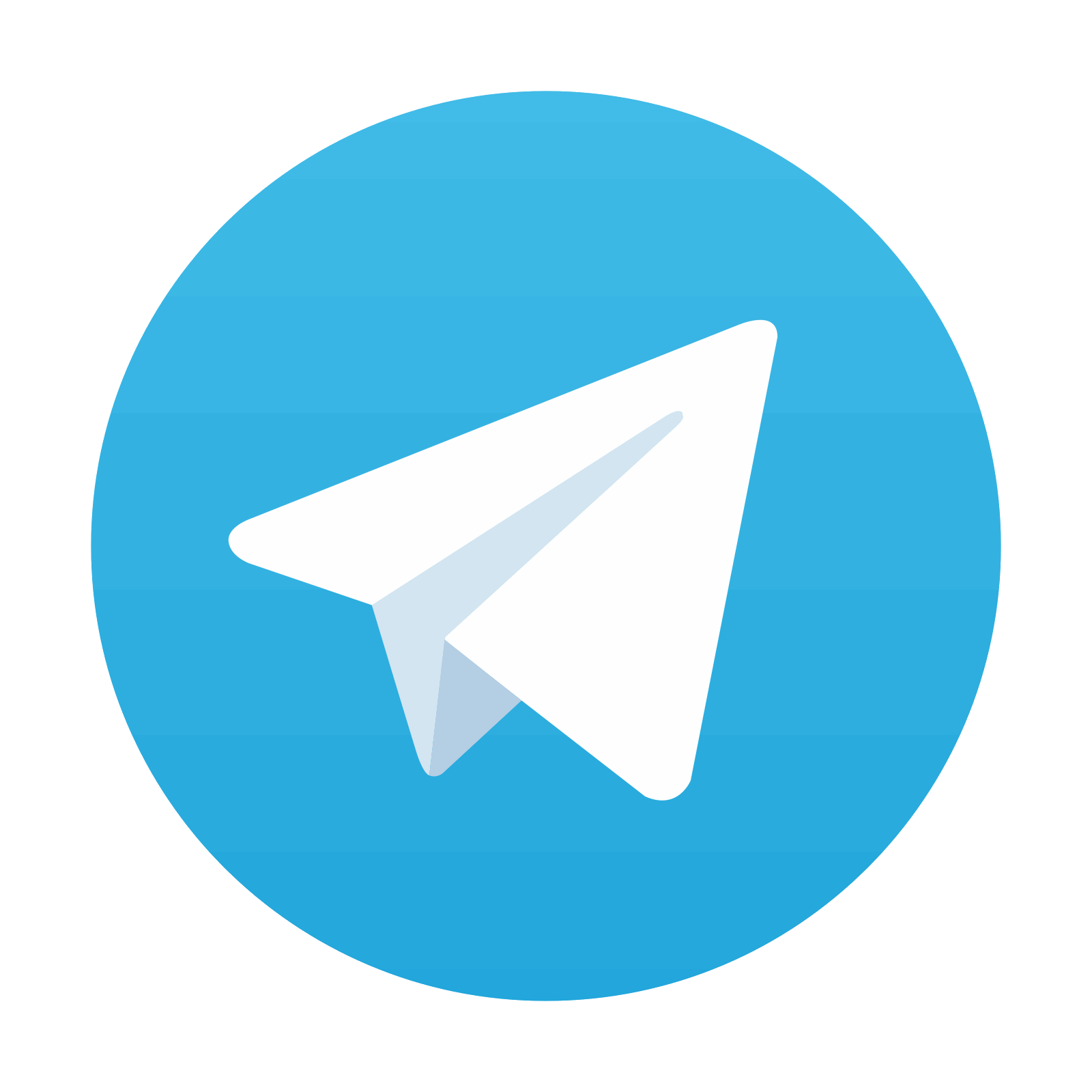
Stay updated, free articles. Join our Telegram channel

Full access? Get Clinical Tree
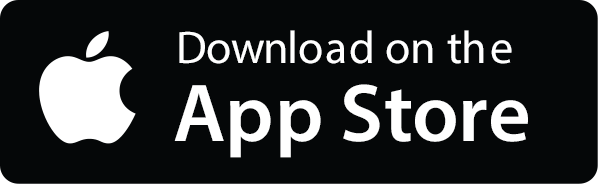
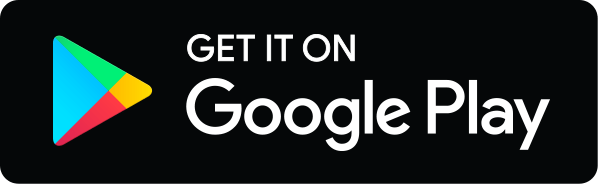