Diseases of the pulmonary venous system have generally been poorly characterized and less frequently investigated compared with diseases of the bronchial tree and pulmonary arterial system. Generally speaking, disorders of the venous system follow a more indolent course. They are progressive in nature and generally do not have a dramatic presentation, with the exception of some congenital disorders that may present dramatically in infancy. Individually, these disorders have a low incidence, which impedes their study. Collectively, however, they affect a significant number of patients and are a challenge for physicians to diagnose and treat. The diagnosis of most of the diseases described in this section are only considered after more common causes of respiratory symptoms have been ruled out, specifically infection. Although delay in diagnosis is frustrating for physicians, there does not appear to be an effect on prognosis in most cases. This is most likely because of the slow progression of these diseases and the lack of effective therapies. This chapter reviews pulmonary vein embryology and describes diseases of pulmonary veins. It also discusses diseases of the pulmonary lymphatic system and briefly mentions disorders that are extremely rare and described only in case reports.
The tissue that gives rise to the pulmonary vasculature is hypothesized to have two distinct origins. The proximal vessels, including the pulmonary veins, are thought to develop through angiogenesis, a process by which existing vessels give rise to new ones by branching, beginning at the pulmonary truncus, an outgrowth of the primitive left atrium. The peripheral vessels, on the other hand, are thought to develop in situ from progenitor cells of lung mesenchyme. This process is a bit more complex, involving formation of a primitive primary plexus, which undergoes remodeling, resulting in a mature network of vessels varying in size. This process is termed vasculogenesis.1 The two separately formed groups of vessels subsequently fuse, communicating the peripheral lung to the heart.2 Morphogenesis and cell differentiation are controlled by numerous gene products, including transcription factors, peptide growth factors, intracellular adhesion molecules, and cell adhesion receptors. Ongoing research continues to provide insight into the genetic pathways that control pulmonary vasculature. Specific genes and pathways that have been identified as essential for normal lung vasculature development include vascular endothelial growth factor A (VEGF-A), endothelial monocyte activating polypeptide II (EMAP II), transforming growth factor β family (TGF-β), insulin like growth factors I and II (IGF-I, IGF-II), forkhead box transcription factors, and the Wnt signaling pathway. The absence of appropriate expression of these genes and pathways results in disrupted pulmonary vasculature development and early embryonic demise.3,5,6,7,8,9,10,11,12,13,14 Although the molecular and morphologic mechanisms that give rise to the pulmonary vasculature have not been clearly delineated, the chronologic events that take place have been well established for a considerable length of time.
Much of what we know about the chronologic development of pulmonary veins can be attributed to the studies and writings of Dr. Catherine A. Neill.4 In 1956, she published a landmark paper in Pediatrics in which she focused on the development of the pulmonary veins in embryos from 2 to 16 mm in size. Before publication of Dr. Neill’s paper, Streeter had broken down the development of the human embryo into “horizons” characterized by embryo size. This system has proved to be useful because it uses both embryo length and approximate embryo chronologic age. This allows for “cross-referencing” of events (Figure 20-1).
FIGURE 20-1.
Displays Dr. Streeter’s system of characterizing embryo growth and shows various milestones in different stages or horizons.
(Copied with permission from: NEILL, C. A. (1956). Development of the pulmonary veins; with reference to the embryology of anomalies of pulmonary venous return. Pediatrics, 18(6), 880–887.)

The development of the four heart chambers and the development of the vasculature that connects the heart to the lungs occurs between horizons XII and XVI when the embryo is approximately 3 and 11 mm, respectively. At the age of 25 days (horizon XII), the human embryo is approximately 3 mm in length, the heart is S shaped, and the sinus venosus communicates only with what will become the right atrium. At this stage, although the lung buds are present, there is no evidence of pulmonary circulation, and there are no well-defined vessels in the area. Horizon XIII occurs at age 27 to 29 days, when the embryo is approximately 4 to 5 mm in length. At this stage, the earliest stages of cardiopulmonary vasculature development can be seen with a collection of mesenchymal progenitor cells, angioblasts surrounding both lung buds, and developing bronchi. An endothelial extension of the left atrium can be seen growing toward the lung bud, which will ultimately become the common pulmonary vein. These events are reflected in Figure 20-2, which is a drawing taken from Dr. Neill’s publication. By the time the embryo reaches days 28 to 30, it is 6 to 7 mm in length, and the bronchi are well developed. At this stage, the pulmonary vein is a well-defined vessel connecting the left atrium to the pulmonary venous plexus. By horizon XV, days 30 to 32, the embryo is 7 to 8 mm in length. Blood is now contained within the pulmonary vein, which is functional and draining the pulmonary plexus into left atrium. The location of the common pulmonary vein in relation to the surrounding structures at this stage is important, particularly the right and left horns of the sinus venosus. The slightest shift in location of the pulmonary vein during this stage may result in abnormal connection to either of these structures. In stages XVI to XVIII, the common pulmonary vein resorbs into the wall of the left atrium. The resorption of the common pulmonary vein into the wall of the left atrium is thought to be an asymmetric process resulting in the formation of four pulmonary veins.4,14
FIGURE 20-2.
The figure illustrates the developing heart and the initial stages of pulmonary vein development.
(Copied with permission from: NEILL, C. A. (1956). Development of the pulmonary veins; with reference to the embryology of anomalies of pulmonary venous return. Pediatrics, 18(6), 880–887.)

The lymphatic system develops much later compared with the venous system. It is thought to begin during the sixth week of life as an outgrowth of the venous system or from differentiation within the mesenchymal tissues. Migration of primordial differentiated lymphatic tissue results in the formation of lymphatic channels and sacs. The largest sacs are paired—juguloaxillary, inguinal, retroperitoneal, and a single cisterna chyli. Endothelial buds originate from these structures and form the peripheral lymphatic plexus. Early in the development of the lung parenchyma, lymphatic tissue makes up a significant portion of the lung volume. As growth of the fetus progresses, the alveolar tissue grows, and around the twentieth week of growth, the lymphatic tissue begins to regress.15 Most of later development is characterized by growth. Morphogenesis continues to take place, however, and normal pulmonary vein development continues to be influenced by the development of surrounding structures and normal blood and lymph flow.
Total anomalous pulmonary venous return (TAPVR), or total anomalous pulmonary vein connection (TAPVC), is a rare congenital malformation in which all four pulmonary veins do not connect normally to the left atrium but instead drain abnormally to the right atrium by way of an abnormal (anomalous) connection. Although genetic and environmental factors have been implicated, the cause of this malformation has not been established.22 The overall incidence of TAPVR has not been reported; however, in a large study examining 2659 pediatric cases with cardiovascular abnormalities, TAPVR was found to occur in 41, or in 1.5% of all patients with cardiovascular malformations. The regional prevalence was reported as 6.8 per 100,000 live births.22 TAPVR is classified into different types based on the location of the abnormal pulmonary vein connection. In the case of supracardiac TAPVR, the pulmonary veins drain via superior vena cava directly into the right atrium. The pulmonary veins come together behind the heart and then drain upward to an abnormal vertical vein. This vein joins the innominate vein which connects to the right superior vena cava and drains into the right atrium. In cardiac TAPVR, the pulmonary veins come together behind the heart and then drain directly to the right atrium via the coronary sinus. The coronary sinus is the vein that normally returns blood from the heart muscle itself back to the right atrium after its oxygen has been depleted. The coronary sinus drains directly into the right atrium. A third type of TAPVR is infracardiac TAPVR. In this case, the pulmonary veins drain to the right atrium via the hepatic veins and inferior vena cava. In this type, the pulmonary veins join together behind the heart and then typically drain downward, connecting to the liver’s portal vein system. They then drain through the vascular bed of the liver and enter the right atrium from the hepatic veins. Obstructed pulmonary veins most commonly occur in the infracardiac type of TAPVR. Figure 20-3 shows the various ways in which the pulmonary vein could abnormally drain into the heart.16,17,18,19,30
Despite anatomical differences, all of these malformations result in similar clinical consequences. Common to all types of TAVR is an atrial septal defect (ASD). Because none of the pulmonary veins connect normally to the left side of the heart, blood is shunted from the right atrium across the ASD. Absence of an ASD in TAPVR is not compatible with survival. Diminished oxygen content of the arterial blood and an increased volume load on the right ventricle characterize the physiology of TAPVR. The abnormal pulmonary vein connection results in mixing together of the oxygenated blood returning from the lungs (in pulmonary veins) and the unoxygenated blood returning from the body (in the vena cava). The left atrium and left ventricle are filled only by blood shunting across an ASD, from the right atrium to the left atrium. The mixture of oxygenated and unoxygenated blood leads to an overall decrease in oxygen content in the blood ejected by the left ventricle to the aorta, resulting in low arterial oxygen saturations. The ASD results in the increased volume load on the right ventricle, which is the characteristic of TAPVR. When the ASD is small or restrictive to blood flow from the right atrium, the volume of blood filling the left atrium and left ventricle may be diminished. This may lead to low cardiac output and shock.
The clinical presentation of patients with TAPVR largely depends on whether pulmonary vein obstruction is present. Patients with preserved pulmonary venous flow present with symptoms more similar to a large ASD. Presenting symptoms include recurrent respiratory infections, failure to thrive, and greater respiratory effort than normal with exertion. Findings on cardiac examination may include a wide split-second sound, an increase in right ventricular impulse, and a pulmonary outflow murmur with or without a tricuspid diastolic murmur. Cyanosis infrequently occurs in the first year of life. If restriction develops in the foramen ovale, some degree of pulmonary hypertension is more likely, with earlier onset of tachypnea, louder pulmonary closure sound, more prominent right ventricular impulse, and a greater likelihood of systemic and pulmonary venous congestion. Cardiomegaly is often seen on chest radiography.
Patients with obstructed pulmonary veins present more dramatically, and findings on physical examination include severe cyanosis with significant respiratory distress. Findings on cardiac examination include a prominent P2 and an anteriorly displaced point of maximum impulse (PMI). A gallop may be present as well as a variable occurrence of a systolic murmur over the pulmonary area or a tricuspid insufficiency murmur at the mid and lower left sternal border. Peripheral pulses are usually normal after birth but may decrease as heart failure progresses. Hepatomegaly is commonly seen, especially in TAPVC with infracardiac venous drainage. In some cases of TAPVR, the route of blood from the pulmonary veins back to the heart may have areas of narrowing or obstruction. This obstruction may prevent adequate blood return from the pulmonary veins and may increase the pressure in the veins, leading to pulmonary edema and pulmonary hypertension. Patients with obstructed TAPVR are usually critically ill with severe cyanosis and often have marked hemodynamic instability. Emergent surgical intervention may be necessary in these cases. Pulmonary venous obstruction occurs in virtually all patients with infracardiac drainage and in approximately 50% of patients with supracardiac drainage. Patients with obstruction develop symptoms early, usually at age 24 to 36 hours, including tachypnea, tachycardia, and cyanosis. Signs of pulmonary hypertension progress with decreasing pulmonary blood flow and worsening cyanosis. The natural history is that of progressive clinical deterioration and early death in the first week or month of life, depending on the degree of pulmonary venous obstruction.25,26
The diagnosis of TAPVR is made by echocardiography. This study will display abnormal drainage of the pulmonary veins in a supracardiac, cardiac, or infracardiac pattern. Additional findings on echocardiography that support the diagnosis of TAPVR include enlargement of the right atrium and right ventricle. Doppler studies can assess the size of and flow across the ASD. Cardiac catheterization may be required to make a definite diagnosis of TAPVR. Cardiac catheterization allows for direct visualization of the pulmonary veins and will define the abnormal connection. Cardiac catheterization is frequently required in cases of mixed TAPVR in which pulmonary venous return demonstrates both supracardiac and infracardiac patterns. Cardiac catheterization can also identify pulmonary veins obstruction and help define the ASD.27,28,29
The mainstay of therapy for TAPVR is surgery. Surgical correction consists of three parts: connecting the common pulmonary vein to the left atrium, dividing the vertical pulmonary vein, and closing intraarterial shunts, if present. Surgical treatment is emergent in patients with pulmonary vein obstruction. The technical details of surgical correction are beyond the scope of this chapter and therefore are only described briefly. In the case of supracardiac TAPVR, the surgeon ligates the left-sided vertical vein at its junction with the binominate vein. The surgeon then opens the left atrium and incises the posterior wall. The surgeon then incises the anterior wall with the venous confluence. He or she creates an anastomosis of the pulmonary venous confluence to the left atrium and closes the ASD or patent foramen ovale. When the connection is to the azygous vein, the surgeon ligates and performs a direct anastomosis of the venous confluence to the posterior aspect of the left atrium. In the case of infracardiac TAPVR, the surgeon ligates the descending vertical vein at the level where it enters the diaphragmatic hiatus after establishing cardiopulmonary bypass. The surgeon then opens the left atrium and incises the posterior wall. The surgeon also incises the anterior wall of the venous confluence, which he or she anastomoses to the left atrium, and then closes the patent ductus with a patch.20,21,24,31,32 Complications of surgical repair have not been well characterized; however, a hepatic necrosis was recently reported after surgical repair of TAPVR.23
Pulmonary veno-occlusive disease (PVOD) has been identified as a rare cause of pulmonary hypertension. The first well-established case of PVOD was documented by Dr. Julius Hora at the University of Munich in 1934. Despite more than 7 decades of investigation, the underlying pathophysiology continues to be poorly understood, and clinical outcomes continue to be disappointing. The epidemiology of PVOD is not well known because many cases of the disease are misdiagnosed as primary pulmonary hypertension (PPH). It has been suggested that up to 25% of cases diagnosed as PPH may meet criteria for PVOD. In a pooled review of seven series characterizing pulmonary hypertension, PVOD accounted for 10% of cases. Using these data as an accurate representation of pulmonary hypertension patients, the incidence of PVOD would be estimated at 0.1 to 0.2 cases per million.47 The quest to find a single etiologic agent responsible for the onset and progression of PVOD has been disappointing. The currently favored theory is that PVOD has no single etiological origin but instead can result from many different insults. No risk factors for the disease have been well founded; however, many possible culprits have been suggested. The association of PVOD with certain viral and bacterial infections suggests a possible postinfectious cause with specific pathogens including toxoplasma, rubeola, cytomegalovirus, Epstein-Barr virus, and HIV.36 PVOD has been seen in patients after treatment for a variety of malignancies and has been suggested to result from exposure to chemotherapeutic agents; however, the supporting evidence is limited to case reports. Specific chemotherapy agents that have been identified in case reports are mitomycin C, bleomycin, and the combination of mitomycin and cisplatin. The chemotherapeutic agents, mitomycin and bleomycin have been identified in cases of autopsy-proven PVOD. One report involved the treatment of two patients with mitomycin C after surgery for non–small cell lung cancer. A second report involved the treatment of a patient with metastatic cervical carcinoma with bleomycin, mitomycin C, and cis-platinum, and a third report described PVOD in two patients with lymphocytic lymphoma after treatment with bleomycin.39,42,54 PVOD has also been diagnosed at autopsy in patients who had received bone transplantation for treatment of acute lymphocytic leukemia, so this has also been considered as a possible cause. In this circumstance, PVOD was suggested as an end consequence of an autoimmune reaction similar to a transfusion reaction that had occurred in the lungs.58 Interestingly, no cases of PVOD have been reported associated with transfusion-related acute lung injury. PVOD has not been reported in cancer patients who have not received chemotherapy, suggesting that PVOD is not a consequence of the malignancy itself. PVOD has also been diagnosed in patients with a variety of known autoimmune diseases, including systemic lupus erythematosus, scleroderma, mixed connective tissue disease, and Hashimoto’s thyroiditis.41,43,44,59 Such cases are in the minority, and there has been little success in elucidating the underlying autoimmune process or identifying serologic markers associated with PVOD.
It is not surprising that the possibility of a genetic predisposition for PVOD has been considered. This hypothesis is supported by the fact that at least two sets of siblings have been diagnosed with PVOD. In these cases, no other environmental cause could be identified; however, given the shared environment, an environmental cause could not be conclusively excluded.35,57 In 2003, a collaborative evaluation of a mother–son set (son with proven PVOD and mother with suspected PVOD) attempted to identify the gene responsible for transmission of PVOD. The researchers suggested that PVOD may be caused by an inherited mutation in bone morphogenic protein receptor II (BMPR-II). Genetic analysis of the son with biopsy-proven PVOD and extended family members was successful in showing that the son had likely inherited the BMPR-II mutation from his mother. Details of the mother’s death, however, were limited to reported complications of right heart failure as a result of elevated pulmonary artery pressures illustrated on right heart catheterization. The absence of a postmortem evaluation made confirming the diagnosis impossible.55 A recent analysis of 24 cases of PVOD reported a BMPR–II mutation in two of those cases; interestingly, the mutation was at a different location than reported in the mother–son case.48
Although the underlying mechanism(s) remains poorly understood, the pathology and natural history of PVOD are well characterized. The pathologic hallmark of PVOD is rapidly progressive fibrosis, cellular proliferation, and muscularization of pulmonary venules, resulting in eventual occlusion of these vessels. The process involves the intimal, medial, and adventitial layer of the venules. Intimal and adventitial thickening is seen as well as muscularization and arterialization of the media. Additionally, the process has an external (intravascular) component with deposition of fibrous and cellular material contributing to the occlusion of the vessel. The material that occludes the vessels varies from dense fibrous material to a looser, edematous material, possibly representing different stages of disease. The occlusion of veins has been described as both an eccentric and concentric process; the intimal thickening and medial muscularization is generally concentric, and the deposition of fibrous and cellular material is generally eccentric. The disease preferentially affects the venules and small veins of the lobular septa and much less frequently the larger veins (Figure 20-4).34,47,51
FIGURE 20-4.
Cross-section of a small pulmonary vein showing complete luminal obliteration by pink collagen (between arrows).
(Copied with permission from Mandel, J., Mark, E. J., & Hales, C. A. (2000). Pulmonary veno-occlusive disease. American Journal of Respiratory and Critical Care Medicine, 162(5), 1964–1973.)

The vein or venule may be recanalized in the later stages of the disease. Arterial changes have been described, but they are not consistently present and not nearly as profound as the changes seen in the venules. It is unclear whether these changes have clinical consequences or contribute to the progression of the disease. Arterial changes consist primarily of medial hypertrophy, although arteritis and adventitial thickening are also described. Pathologic changes are not limited to the blood vessels; they may also be present in the lung parenchyma. Parenchymal abnormalities include interstitial edema and deposition of collagen fibers, which are best seen in the lobular septa but can also be seen in the alveolar wall. Alveolar hemorrhage can be seen as well as dilated lymphatics, but this may be a consequence of focal congestion resulting from venule occlusion rather than part of the disease process.34,47,51
The clinical presentation of PVOD is nonspecific with the most common initial complaints being exercise intolerance, lethargy, and progressively worsening dyspnea. Less frequent initial symptoms include chronic cough and a presumed respiratory tract infection that fails to resolve with antibiotic therapy. The more severe and dramatic initial presentations of the disease, including hemoptysis and sudden death, are fortunately rarely seen. The clinical picture of PVOD is very similar to that of PPH. Syncope, clubbing, and hemoptysis were previously thought to be more common in PVOD than PPH, but the most recent analysis of 24 cases of PVOD did not find these features helpful in differentiating between the two diseases clinically.47,48,53 PVOD may have a more rapid progression and may be associated with worse outcomes than PPH. Many patients succumb to PVOD within 2 years of onset of symptoms, and the diagnosis is unfortunately often made at autopsy.56
The definitive diagnosis of PVOD can only be made histologically. However, certain radiographic, hemodynamic, laboratory, and pulmonary function features of the disease may raise the index of suspicion for PVOD and may help distinguish it from PPH and other pulmonary diseases. Common findings on chest radiographs include scattered patchy opacities; central vascular congestion; and Kerley B lines. All three of these findings are thought to be a consequence of chronic capillary hypertension.33 Pleural effusions are more likely to be present in PVOD than PPH and may help distinguish between the two disorders.47 The radiographic hallmark on computed tomography (CT) is smooth septal thickening, which is seen in virtually all PVOD patients. Other common yet nonspecific findings on CT include ground-glass opacities, multiple small nodules, pleural effusions, areas of alveolar consolidation, and lymph node enlargement. Of these findings, septal lines, lymph node enlargement, and centrilobular ground-glass opacities are more frequently found in PVOD than PPH. The presence of multiple abnormalities on CT seems to be associated with PVOD and helps distinguish it from PPH; however, the absence of radiographic findings is not sufficient to rule out PVOD (Figure 20-5).38,47,48,52
FIGURE 20-5.
A. CT of chest showing septal thickening, marked ground-glass opacities in centrilobular pattern and nodular opacities. B. Lymph node enlargement.
(Copied with permission from Montani, D., Achouh, L., Dorfmuller, P., Le Pavec, J., Sztrymf, B., Tcherakian, C., et al. (2008). Pulmonary veno-occlusive disease: Clinical, functional, radiologic, and hemodynamic characteristics and outcome of 24 cases confirmed by histology. Medicine, 87(4), 220–233.)

Although routine right heart catheterization has not been suggested in the evaluation of persons suspected to have PVOD, it may be helpful in diagnosing and measuring the severity of the disease. Hemodynamic measurements are similar to those in PPH. The most striking finding during right heart catheterization is an elevated pulmonary artery pressure; however, right atrial pressure is also elevated. Pulmonary artery wedge pressure is usually normal. One notable finding during right-sided cardiac catheterization of these patients is the inability to obtain a pulmonary artery wedge pressure tracing. When the wedged catheter is flushed, the pressure increases rapidly and disproportionately and is slow to return to baseline. A proposed explanation for this is the saline becomes trapped between the balloon and the narrowed pulmonary veins.47 Hemodynamic parameters may be helpful in differentiating PVOD from PPH because PVOD has been reported to have relatively less elevated right atrial pressure and lower mean systolic arterial pressure (systemic) than PPH.48
Pulmonary function testing is an additional tool that may be helpful in diagnosing and differentiating PVOD from PPH. Pulmonary function testing results in PVOD are nonspecific, usually revealing a restrictive pattern with diminished diffusion lung capacity for carbon monoxide (DLCO).40 DLCO is frequently around 50% of predicted, which is a significantly lower value than that seen in PPH. Hypoxemia is usually present and is also worse than in PPH. In the study previously mentioned comparing 24 cases of PVOD with 24 cases of PPH, the mean SaO2 in the PVOD was 61.3 compared with 75.4 in the PPH group.47,48
The presence of pulmonary arterial hypertension, radiographic evidence of pulmonary edema, and a normal pulmonary artery occlusion pressure has been previously thought to be suffice to diagnose PVOD. However, many patients do not present with all three findings, and these findings cannot always differentiate between PVOD and PHH. Definitive diagnosis can only be made with surgical lung biopsy. Given the rapid progression of the disease, patients often present with significantly compromised respiratory function, and the decision proceed to open lung biopsy is not one that is taken lightly. One of the arguments against obtaining a lung biopsy is the lack of effective treatment. Currently, the only treatment strategy that has proven effective in extending life has been lung transplantation.40,47,49 However, most clinicians believe that lung biopsy is warranted in cases of suspected PVOD. Beneficial aspects of obtaining a definitive diagnosis include avoiding treatment of presumed PPH with ineffective and potentially harmful treatment and proceeding with a more timely evaluation for lung transplant.
Currently, there is no effective treatment that has been convincingly shown to slow the progression of PVOD or improve outcomes. Pulmonary vasodilators and immunosuppressive therapy, although used commonly to treat PVOD, lack convincing data to support their use. Available data are limited to case reports and small case series. Vasodilator therapies include calcium channel blockers (CCBs) and prostacyclin (epoprostenol). The evidence for epoprostenol efficacy appears to be slightly better than that for CCBs but is still limited to case reports and therefore inconclusive. However, epoprostenol continues to be the standard treatment for proven PVOD.45,50
Treatment of patients with PVOD is not without risk of complications. Treating patients with PVOD with CCBs and immunotherapy has been a reported to be associated with significant side effects. An increased risk of pulmonary edema has been reported in patients with PVOD who received vasodilator therapy, specifically CCBs.48 The underlying mechanism for this pulmonary edema may be the preferential dilatation of pulmonary arterioles with persistent occlusion of the venules, resulting in alveolar congestion. Sildenafil therapy has also been considered, but again, evidence to support its use is limited to case reports.45,46,49 Response to immunotherapy is limited to case reports. In cases of favorable response to immunotherapy, patients had evidence of coexisting autoimmune disease and elevated serologic markers.47 PVOD patients are anticoagulated with warfarin unless there is a contraindication to do so because of early observational trials showing improved outcomes.37 Current experimental treatments included a variety of antithrombotic agents such as heparin and tissue plasminogen activator; however, the natural history of PVOD does not suggest that thrombosis plays a major role, and these therapies are not likely to have an impact on survival.47 Oxygen therapy is recommended in the treatment of patients with PVOD. As previously mentioned, lung transplantation is felt to be the only therapy to improve outcomes in PVOD.
Pulmonary vein stenosis (PVS) has historically been described primarily in the pediatric population. The initially described form of PVS in children is known as primary or congenital PVS. The other forms of PVS are secondary to either a surgical procedure or an underlying disease process in the vicinity of the pulmonary veins.61,67,68 Because of the rarity of this disorder, no prevalence has been reported. Approximately two to three cases per year are severe enough to require treatment. The condition is even rarer in adults and is usually associated with a mediastinal process such as fibrosing mediastinitis or malignancy. More recently, another population of PVS patients has been identified in children and adults who have undergone invasive procedures involving the pulmonary veins. These procedures include radiofrequency ablation procedures for atrial fibrillation in adults and surgery for TAPVR in children. PVS has also been described in adults without a surgical history or identified underlying mediastinal process, but this group of patients is limited to case reports.74,85 Based on distinct causes, this condition can be divided into different categories.
The pathophysiology of the primary form of PVS seen in children is distinctly different than the other forms. Primary (sometimes called congenital) PVS is defined as PVS with normal venous connections and no previous surgery. This form of PVS was previously thought to be primarily congenital malformation present at birth, but it is now believed that a majority of these cases represent an acquired defect that occurs in conjunction with cardiac defects. A recent review of 26 cases of primary PVS found that echocardiograms at birth or shortly thereafter were without abnormalities of the pulmonary veins. Nearly all of the patients had congenital heart defects, particularly those that result in a left-to-right shunt (i.e., patent ductus arteriosus, ASD, ventricular septal defect, and atrioventricular septal defect). It has been suggested that the increased pulmonary vein flow may be in part responsible for the stenosis; however, the underlying mechanism has yet to be elucidated. Prematurity, a known risk factor for congenital heart defects, was also found to be a risk factor for primary PVS.79 Bronchopulmonary dysplasia (BPD) was seen in approximately one-third of these subjects. However, it is unclear whether the findings of venous congestion, interstitial edema, and elevated pulmonary artery pressures are caused by PVS or BPD.65 The underlying mechanism leading to stenosis of the pulmonary vein(s) is poorly understood but is thought to be related to turbulent flow created by concurrent heart defects.82 The presence of PVS in the absence of cardiac defects has been described, which would lead to the assumption that other factors may contribute to the development of PVS as well. A postmortem evaluation of the pulmonary veins in a small group of autopsy-proven PVS cases characterized the stenotic tissue as made up of myofibroblast-like cells. This was based on the histologic appearance, immunochemical staining, and a variety of surface markers found on lesional cells. The expression of various receptor tyrosine kinases, including vascular endothelial growth factor receptor, platelet-derived growth factor receptor, and fibroblast growth factor receptor, have been demonstrated, suggesting that intercellular signaling may play a role in the development of primary PVS.78 Conceivably, the turbulent flow within the pulmonary vein may lead to a change in cell surface marker and receptor profile, which allows the disease process to progress. However, much remains to be understood in the pathogenesis and mechanisms involved in PVS. Regardless of the underlying mechanisms, the resulting condition is a rapidly progressive stenosis of the pulmonary vein(s) with eventual occlusion of one or more vessels. The histopathology seen in PVS is nicely displayed in Figure 20-6, in which panel A shows the obliteration of the pulmonary vein.
FIGURE 20-6.
H&E staining displaying the occlusion of the pulmonary vein by the stenotic lesion and myofibroblas features of the lesional cells.
(Copied with permission from T. Riedlinger, W. F., Juraszek, A. L., Jenkins, K. J., Nugent, A. W., Balasubramanian, S., Calicchio, M. L., et al. (2006). Pulmonary vein stenosis: Expression of receptor tyrosine kinases by lesional cells. Cardiovascular Pathology: The Official Journal of the Society for Cardiovascular Pathology, 15(2), 91–99.)

The course of PVS varies considerably depending on its cause. Patients with primary PVS typically present within 1 to 2 years of life, although many present within the first months of life. A small number of patients present significantly later. Patients typically experience recurrent pulmonary infections and persistent tachypnea. The severity of pulmonary symptoms is related to the number of pulmonary veins involved and the degree of stenosis. Signs of pulmonary hypertension become evident as the disease progresses. PVS may involve a single pulmonary vein or all four. When the condition is unilateral, perfusion favors the unaffected lung, and symptoms may take longer to appear. In bilateral disease, patients display symptoms more rapidly, and pulmonary edema is frequently seen. Hemoptysis is rare in young children but can be seen in older children and adults. The disease is often rapidly progressive with progressive worsening of symptoms and decline in respiratory status. The overall prognosis for primary PVS varies significantly among studies but is consistently poor. Death often occurs within months, and the overall mortality rate is around 50%.65,66 An earlier age of diagnosis and higher initial pulmonary artery pressures are associated with worse outcomes.66 The development of corrective surgical procedures for TAPVR has led to a new group of PVS in children. The incidence of PVS in children undergoing surgery for TAPVR varies significantly in different series but has been estimated as high as 50%. PVS in this population is usually diagnosed within 6 months of the TAPVR repair.63
The disease process and underlying mechanisms are presumably the same in adults; however, PVS has been less well studied in this patient population. The presentation in adults is usually preceded by an identifiable mediastinal process such as sarcoidosis or fibrosing mediastinitis. Patients present with progressively worsening shortness of breath and may have radiographic findings resembling an infectious process on chest radiographs. Symptoms persist without improvement with antibiotics. Patents tend to present late in the course of the etiologic disease process.62,73
Adult patients who have undergone catheter radiofrequency ablation for atrial fibrillation make up the fastest growing population of PVS patients. PVS was recognized as a complication of this procedure early in its development. Modifications to this procedure have significantly reduced the incidence of PVS; however, 2% to 5% of patients who undergo this procedure experience PVS.71,76,80,81 Direct injury to previously anatomically normal pulmonary veins differentiates the pathophysiology of PVS in this group. Thermal injury to the pulmonary veins and the subsequent healing process is thought to play a major role in the development of PVS in this group.86 These patients present at various lengths of time after the procedure. Diagnosis is often delayed by extensive evaluation or treatment for an interstitial or infectious process. PVS is not usually considered until a negative workup or failure to respond to therapy is encountered.80 Patients are typically asymptomatic unless there is greater than 70% stenosis. Respiratory symptoms are associated with more severe PVS or multiple vein involvement. PVS in this group also appears to be a less aggressive disease process with better reported outcomes. Pulmonary vein angioplasty has had significantly better outcomes in this population compared with other types of PVS. There persists a significant risk of restenosis; however, restenosis does not necessarily translate to return of respiratory symptoms. After pulmonary vein angioplasty, approximately 90% of patients remain symptom free despite restenosis.84 The long-term sequelae of this restenosis remains to be seen.77 Given the increasing use of catheter ablation to treat atrial fibrillation, pulmonologists and electrophysiologists need to have a heightened awareness of the potential for this complication in this specific patient population.
The diagnosis of PVS can be made by noninvasive means. Doppler echocardiogram has proven to be one of the most useful studies in detecting PVS and is generally thought to be sufficient in the appropriate clinical setting. The pathognomonic finding indicating pulmonary vein obstruction is a pulmonary vein flow velocities of more than 1.6 m/sec. In addition to providing information about the pulmonary veins, echocardiography allows for the evaluation of other cardiac abnormalities.83,85 Figure 20-7 shows the accelerated blood flow seen on Doppler echocardiogram in PVS.
Other noninvasive studies have proved helpful in supporting the diagnosis. Perfusion/ventilation scan demonstrates decreased ventilation of the affected lung. The gold standard for diagnosis of PVS is angiography, which offers the most selective view of the pulmonary veins and clearly displays stenosis when present. Given the invasiveness of the procedure and the multiple noninvasive studies available, angiography is not usually required to make the diagnosis (Figure 20-8).68
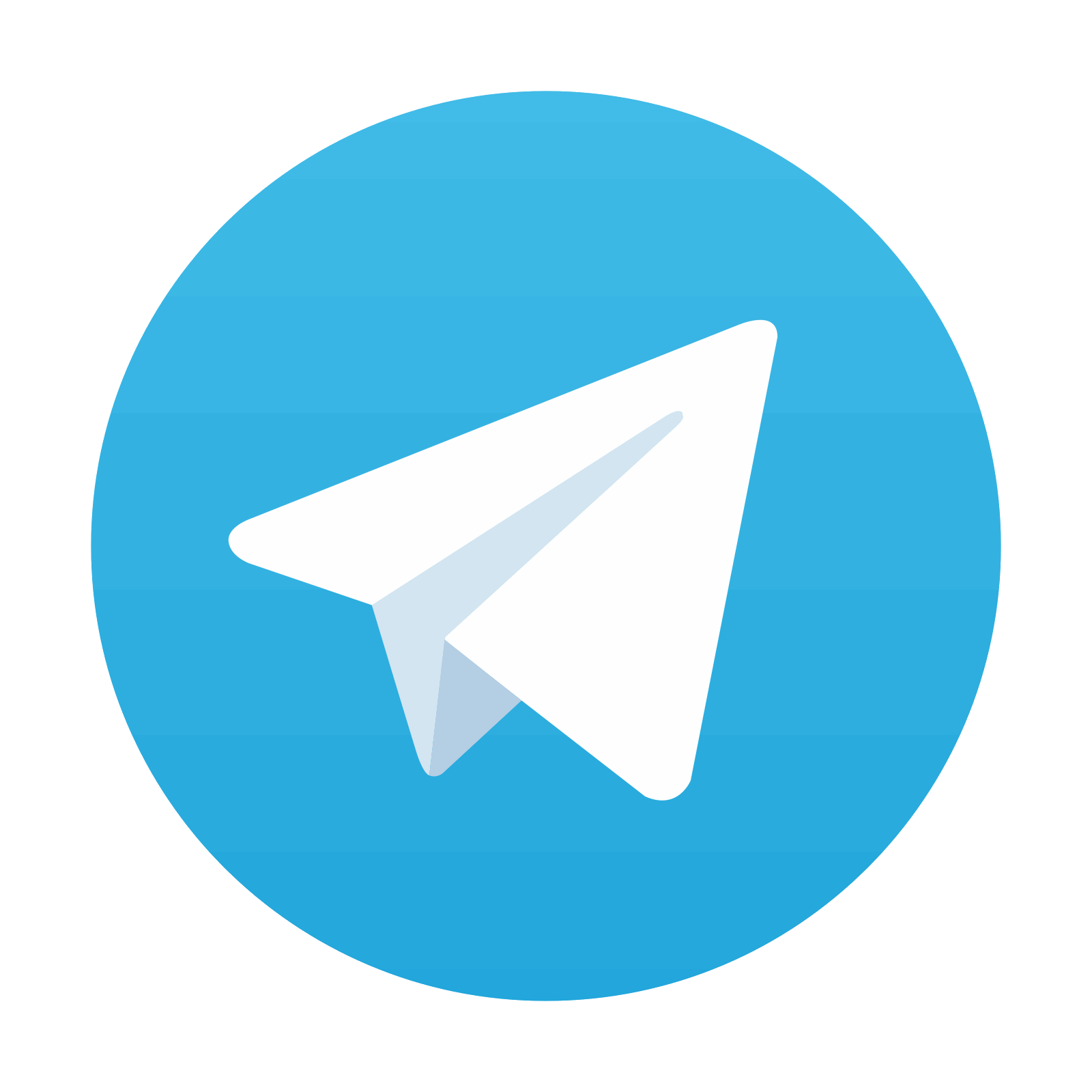
Stay updated, free articles. Join our Telegram channel

Full access? Get Clinical Tree
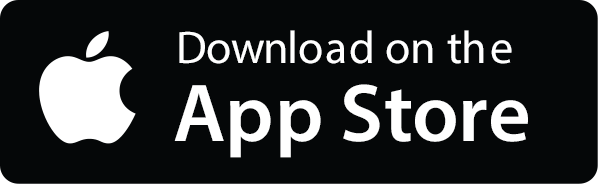
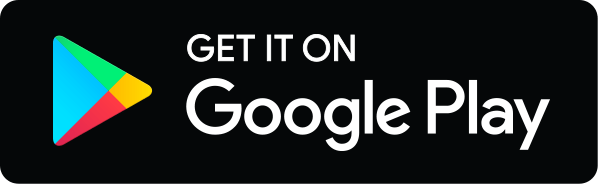