Pulmonary hypertension describes a number of different diseases involving the pulmonary vasculature, which have haemodynamic, histological, and therapeutic features in common. The hallmark of these diseases is an abnormally high pulmonary arterial pressure, which—except for large intracardiac shunts permitting transfer of pressure—is due to an increase in the resistance of the pulmonary vasculature, either arterial or venous, to the flow of blood. The histological changes are relatively uniform, and show only vaguely specific dependence on the cause, be it idiopathic, haemodynamic, inflammatory, toxic, or other. The haemodynamic and clinical consequences depend not only on the extent and speed of these changes, but also on the state of the subpulmonary ventricle, which is most usually the morphologically right ventricle.
The different diseases resulting in increased pulmonary vascular resistance show a spectrum of responses to the currently available specific pulmonary antihypertensive substances, with disease-specific profiles of response beginning to emerge. With regard to the individual patient, nonetheless, a correct understanding of the precise pathophysiological situation in which pulmonary vascular resistance is increased and causes pulmonary hypertension is of crucial importance for the correct commencement, and evaluation of efficacy, of treatment.
CLASSIFICATION OF PULMONARY HYPERTENSION
Already in 1891, Ernst von Romberg had described changes in the pulmonary vasculature,
which he called sclerosis of the pulmonary arteries. 1 Subsequently, the term primary pulmonary hypertension was coined in 1951. 2 The original classification of chronic cor pulmonale was established by the World Health Organization in 1973, 3 and basically covered three aetiologic groups:
- •
Diseases primarily affecting the airways of the lung and the alveoluses, such as chronic bronchitis, bronchial asthma, emphysema, pulmonary granulomata, pulmonary resection, congenital cystic disease of the lungs, and high-altitude hypoxia.
- •
Diseases primarily affecting the movement of the thoracic cage, such as kyphoscoliosis, thoracoplasty, pleural fibrosis, chronic neuromuscular weakness, obesity with alveolar hypoventilation, and idiopathic alveolar hypoventilation.
- •
Diseases primarily affecting the pulmonary vasculature, such as affections of the arterial wall, thrombotic disorders, embolism, pressure on pulmonary arteries and veins by mediastinal tumours, and so on.
- •
Over the past decades, the classification has been modified, bringing together clinical as well
as aetiologic features with the biological expression of the disease. In 1998, during the Second World Symposium on Pulmonary Hypertension, held in Evian, France, the main focus was to establish categories with similar pathophysiological conditions and therapeutic options. 4 From this meeting emerged the following classification:
- •
Pulmonary arterial hypertension
- •
Pulmonary venous hypertension
- •
Pulmonary hypoxaemic hypertension associated with disorders of the respiratory system
- •
Pulmonary hypertension caused by thrombotic or embolic diseases
- •
Pulmonary hypertension caused by diseases affecting the pulmonary vasculature itself
- •
The Third World Symposium on Pulmonary Arterial Hypertension, held in Venice, Italy, in 2003, allowed an assessment of the usefulness of the classification produced in Evian and its minor modifications ( Table 56-1 ). 5 The clinically functional classification established by the New York Heart Association for cardiac disease ( Table 56-2 ) is used in an adapted form to assess the clinical severity and progression of the pulmonary vascular disease. 5 Currently, pulmonary arterial hypertension is defined as a mean pulmonary arterial pressure of greater than 25 mm Hg at rest, or greater than 30 mm Hg while exercising, with a normal pulmonary arterial wedge pressure of less than 15 mm Hg, and an increased pulmonary vascular resistance index of greater than 3 Wood units times m 2 body surface area. The Dana Point symposium (2008) introduced the term borderline pulmonary hypertension for those disease states in which mean pulmonary artery pressure exceeds 20 mm Hg (personal communication).
|
Class I | Patients with pulmonary hypertension, but without resulting limitation of physical activity. Ordinary physical activity does not cause undue dyspnoea or fatigue, chest pain, or near syncope. |
Class II | Patients with pulmonary hypertension resulting in slight limitation of physical activity. They are comfortable at rest. Ordinary physical activity causes undue dyspnoea or fatigue, chest pain, or near syncope. |
Class III | Patients with pulmonary hypertension resulting in marked limitation of physical activity. They are comfortable at rest. Less than ordinary activity causes undue dyspnoea or fatigue, chest pain, or near syncope. |
Class IV | Patients with pulmonary hypertension with inability to carry out any physical activity without symptoms. These patients manifest signs of right heart failure. Dyspnoea and/or fatigue may even be present at rest. Discomfort is increased by any physical activity. |
PULMONARY HYPERTENSIVE DISEASES
Idiopathic Pulmonary Arterial Hypertension
Idiopathic pulmonary arterial hypertension is characterised by progressive and sustained elevation of pulmonary arterial pressure in the absence of any distinct cause. The diagnosis is made by exclusion of other aetiologies for pulmonary arterial hypertension. There is also some thought that the disease may be a neoplastic and angioproliferative disorder, rather than a vasomotor model centred on vasoconstriction or impaired vasodilation. 6
Pulmonary Veno-occlusive Disease
This is a very rare condition which affects mainly, but not exclusively, children and young adults. Multiple possible factors for its development have been discussed, including infections, genetic aspects, toxic exposure, and autoimmune disorders. 7–13 The clinical presentation is very similar to idiopathic pulmonary arterial hypertension, with shortness of breath, chest pain, syncope, hypoxaemia, and later signs of right-sided cardiac failure. Histological findings ( Fig. 56-1 ) include intimal fibrosis of the pulmonary veins, leading to narrowing and occlusion of affected vessels. 14,15 From the haemodynamic aspect, there is elevated pulmonary arterial pressure, with a normal pulmonary capillary wedge pressure. The chest radiograph (see Fig. 56-3A ) typically shows enlargement of the pulmonary trunk, pulmonary oedema with prominent Kerley B lines, and pleural effusions. On computed tomography (see Fig. 56-3B and C ), the disease manifests with thickened interlobular septums, enlarged lymph nodes, and ground-glass opacities. 16 Definite diagnosis requires lung biopsy, a high-risk procedure where the benefit for the patient needs to be taken into account. Cardiac catheterisation, with testing for vasodilation, may be indicated, but acute pulmonary oedema has been described. 17 Therapy includes supplemental oxygen and diuresis, as well as anticoagulation. 18 There are also reports on the use of immunosuppressive therapy with corticosteroids 19 and azathioprine, 20 but this approach should be limited to patients with suspected or proven collagen vascular disease. Lung transplantation is most likely the only option for treatment, but the long waiting time for organs may limit the final outcome.

Alveolar Capillary Dysplasia
This is a relatively rare cause of persistent pulmonary hypertension of the newborn, but its incidence is most likely underestimated. A retrospective study on neonates treated with extracorporeal membrane oxygenation in the United Kingdom between 1997 and 2000 identified a total of nine patients with such irreversible lung dysplasia. 21 The aetiology is still uncertain, with familial cases suggesting a possible genetic mutation. 22,23 The histological findings include muscularisation of the pulmonary arterioles, a deficiency in the number of alveolar units and pulmonary capillary vessels with thickening of the interalveolar septums, anomalous pulmonary veins accompanying the pulmonary arteries and bronchuses, and dilated lymphatics. 24–26 The newborn usually presents with severe hypoxaemia, without response to treatment with oxygen or pulmonary vasodilators. Open lung biopsy has been recommended if a neonate with persistent pulmonary hypertension shows no clinical improvement after 72 hours despite maximal medical therapy. 25,26 Cardiac catheterisation may be helpful to complete the diagnostic work-up. 27 Currently the disease is incurable, with a maximum reported survival time of about 3 months, albeit sometimes with a phase of mild improvement with the administration of vasodilating agents. 28,29
Congenital Diaphragmatic Hernia
This entity is characterised by pulmonary hypoplasia, with structural as well as functional anomalies, leading to a high pulmonary vascular resistance and pulmonary hypertension. A combination of compression of the lungs during fetal life, limited flow of blood to the lungs, and intrinsic pulmonary developmental arrest are essentially responsible for the parenchymal pulmonary hypoplasia. 30–33 In addition, a dysregulation of the expression of the receptors for endothelin-1 has been documented. 34 This leads to failure of postnatal remodelling, resulting in increased right ventricular pressure and perfusion bias to the unaffected side. The clinical management includes surgical repair in the early neonatal phase, followed by care over the long term. With newer options for treatment like high-frequency oscillatory ventilation, intravenous infusion of prostaglandin, inhaled nitric oxide and extracorporeal membrane oxygenation, better neonatal survival is achieved, 35–37 but the number of patients with chronic lung disease, or recurrent or residual pulmonary hypertension, is also increasing. There are no controlled clinical trials on the use of vasodilators in long-term survivors with elevated pulmonary arterial pressures. Maximal exercise capacity was shown to be mildly decreased when a cohort of patients with this problem was compared to normal controls. 38
Pulmonary Hypertension Associated with Bronchopulmonary Dysplasia or Chronic Lung Disease
Persistently elevated pulmonary arterial pressure related to bronchopulmonary dysplasia is a common finding in ex-preterm infants born with low weight, 39,40 despite preventive therapy, 41 but the pressures tend to fall with increasing age during the first year of life. Improved survival of very immature infants has led to increased numbers of infants with chronic lung disease. Histological findings suggest an arrested alveolar development, or loss of alveoluses, and it was hypothesised that disruption of angiogenesis impairs alveolarisation. In contrast, preservation of vascular growth and endothelial survival may promote growth, and may sustain the architecture of the distal air space. 42 Currently, supplemental oxygen is provided to children with chronic lung disease to prevent hypoxaemia. Other vasodilators, such as oral sildenafil and intravenous or aerosolised prostacyclin, may be considered after careful evaluation and exclusion of fixed pulmonary vascular resistance.
Pulmonary Hypertension Associated with Pulmonary Venous Obstruction
Pulmonary venous obstruction induces both pulmonary arterial and pulmonary venous hypertension. The condition maybe related to anatomical abnormalities of the pulmonary veins, such as partially or totally anomalous pulmonary venous connection or scimitar syndrome, a structural variation such as divided left atrium, or varying occlusion of one or more pulmonary veins, be the occlusion congenital or acquired. In the majority of cases, a surgical or interventional approach is warranted, 43–46 followed by individual assessment for residual pulmonary hypertension and tailored therapy. Whereas the long-term prognosis in the presence of divided left atrium is usually benign, the intrinsic pathology of the pulmonary veins is often progressive.
Pulmonary Hypertension Associated with Congenitally Malformed Hearts: General Considerations
Epidemiology
Pulmonary hypertension in the context of the congenitally malformed heart has a rather small place in the overall classification of pulmonary hypertension (see Table 56-1 ). Such classification is nowadays seen as insufficient, 47 and is currently under revision. There are a multitude of haemodynamic situations in which pulmonary vascular resistance is increased and plays an important part in the state and prognosis of the patient. Furthermore, almost one in every hundred newborns is born with a congenitally malformed heart, and more than 90% of these now survive well into adulthood, albeit 10% to 18% of these have some form of pulmonary hypertension. 48 These are adults with congenitally malformed hearts and pulmonary hypertension, a combination which is quite complex. The number of these patients is already several-fold higher than the number with idiopathic pulmonary arterial hypertension. The patients with congenitally malformed hearts require their own very specialised cardiopulmonary management.
Pathophysiological Considerations
The pulmonary vasculature is located haemodynamically between the right and left ventricles, with the two ventricles sharing the ventricular septum. This truism results in complex interactions. Damage to the arterial side of the pulmonary endothelium may be the result of shear stress due to pulmonary over-circulation in the setting of congenital interatrial communications, ventricular septal defects, or shunts at arterial level, or to the venous side in the setting of malformations that oppose the blood leaving the lungs, such as stenoses of the pulmonary veins, of the mitral and aortic valve and of the aortic isthmus. This last category includes hypoplasia and systolic and diastolic malfunction of the left heart. The pulmonary vasculature may also be congenitally hypoplastic, as in tetralogy with pulmonary atresia or pulmonary hypoplasia due to diaphragmatic hernia and so on, thus producing an intrinsically increased vascular resistance. In these pathophysiological situations, the pulmonary vascular resistance becomes a major factor influencing morbidity and mortality. 49 To estimate the risk of the development and progression of increased pulmonary vascular resistance in these circumstances, it is necessary to factor in the time, pressure, and volume with which the respective lesion is exerting its damaging stimulus to the pulmonary vasculature. Table 56-3 provides a classification of pulmonary hypertension in the setting of congenital heart disease.
Condition | Fontan Circulation | Pre-operative/Transplantation | Post-operative | Left Heart Disease | Eisenmenger Physiology | Pulmonary Atresia |
---|---|---|---|---|---|---|
Resulting clinical problem of pulmonary vascular resistance elevation | Signs of right heart failure, low cardiac output | Post-operative or post-transplant right heart failure, excluding operation/heart transplantation alone | Increased right ventricular afterload ultimately with circulatory collapse and death | Reduced LV preload, septum pushed to the left ventricle; low cardiac output | Reduced transpulmonary flow of oxygenated blood, increased cyanosis | Less transpulmonary blood flow and pulmonary venous return and systemic oxygenation, increased cyanosis |
Treatment aim | Reduced symptoms of right venticular failure | Decreased likelihood of post-operative failure | Decreased RV afterload | Improved LV function and septum position | Increased peripheral saturation | Increased peripheral saturation |
Indication | mPAP > 15 mm Hg, low CO | > 3–4 WU × m 2 BSA | RP > 2/3 R S low RV function | Low cardiac output | PVRI > SVRI saturation <82%–85% | Saturation < 82%–85% |
End point | mPAP or CO | WU × m 2 BSA | mPAP or PVRI | CO, R/LVEDP | Saturation | Saturation |
Fontan Physiology
In those with the Fontan circulation, a small change in pulmonary vascular resistance will have an over-proportionate effect. This means that, while being too small to be measured, with the measurement in itself being a challenge, therapeutic reduction may result in palpable clinical benefits. The increase in pulmonary vascular resistance may be due to pulmonary factors or due to ventricular factors, such as disease of the left heart, that may be intricately entangled.
Pulmonary Vascular Resistance in the Child Prior to Operation or Transplantation
This should be low in the child submitted for a timely procedure, but should be measured later, particularly if it cannot be measured accurately in the setting of large shunts, transposition, common arterial trunk, or atrioventricular septal defect. It correlates with the likelihood, as with transplantation, of post-operative pulmonary hypertensive crises or right ventricular failure.
Chronic Postoperative Pulmonary Hypertension
Successful surgery will reconstitute the normal haemodynamic sequence of flow into and out of the two ventricles. In this situation, it is reasonable to transfer most considerations regarding idiopathic pulmonary arterial hypertension. The congenital cardiac malformation itself, nonetheless, coupled with the length of time the pulmonary vasculature was stressed pre-operatively, along with the surgical interventions, increase the vulnerability to abnormal loading conditions and the likelihood of cardiac failure.
Left Heart Disease
This may cause pulmonary venous hypertension, which results in an over-reactive increase of pulmonary vascular resistance. The consequences of the ensuing right ventricular stress, with right ventricular dilation and hypertrophy, result in an important shift of the septum into the left ventricle, which again inhibits left ventricular function. Thus, although the original cause of pulmonary vascular resistance increase is left-sided, an acute test using vasodilators is justified to differentiate the amount of independent overshooting of pulmonary vascular resistance, which may justify pharmacological treatment to increase the overall ventricular performance and cardiac output.
Eisenmenger Physiology
In this setting, changes in pulmonary vascular resistance will not change right ventricular afterload. This is fixed and, by definition, at systemic level due to the large unrestricted shunt. Changes in resistance, nonetheless, will determine the ratio of systemic to pulmonary flows, and thereby the resulting saturation.
Tetralogy with Pulmonary Atresia
If the flow of blood to the lungs is multifocal, through major aortopulmonary collaterals that are mostly mechanically obstructed, as can occur in very cyanosed patients, any amount of successful reduction of pulmonary vascular resistance due to acute vasodilation or chronic vascular remodeling will clinically justify pharmacological treatment.
Measurement of Pulmonary Vascular Resistance in Congenital Cardiac Disease
Due to the now established early timing for surgical correction of many congenital cardiac lesions, the likelihood of increased pulmonary vascular resistance in the absence of other risk factors at this early time point is low, and can reasonably be neglected when considering surgery. Late presentation, or presentation without a history of pulmonary over-circulation and cardiac failure despite an unrestrictive shunt, would characterise the typical candidate in whom invasive evaluation of pulmonary vascular resistance is indicated.
The pulmonary vascular resistance is very important, but is not the only criterion on which depend the decisions for the type of cardiac surgery or transplantation, pharmacological treatment, or combinations thereof. The gold standard for obtaining such data remains cardiac catheterisation. This invasive method, however, is increasingly challenged by an increased perception of its risk, especially when compared to the non-invasive measurements and increasingly better and more sophisticated approximations that are provided by magnetic resonance imaging. 50,51 Indeed, the measurement of pulmonary vascular resistance in the setting of congenital cardiac disease presents special challenges regarding the indication for catheterisation, the risk and protocol for anaesthesia, and the techniques of measurement and calculations for pulmonary vascular resistance at baseline and under maximum pharmacological pulmonary vasodilation.
While older patients and children may be catheterised under local anaesthesia, general anaesthesia is usually seen as the safer approach for neonates and infants. Its risk 52 is increasingly perceived as prohibitive, and not justifying the value of the obtained data for clinical decision-making, especially in children in whom the dynamics of the process of disease tend to be more aggressive. As in all patients with increased pulmonary vascular resistance, the risk of general anaesthesia occurs specifically during the phase of induction, and during extubation. The risk is exacerbated by a weak clinical state, especially loss of weight, by specific episodes in the past, and by reduced right ventricular function. A steady state during the procedure, aided by continuous administration of agents to avoid haemodynamic variations, especially towards the end of the examination, is crucial. This should provide stable levels of carbon dioxide, and saturations near those achieved during spontaneous ventilation.
The calculation of pulmonary vascular resistance in the presence of shunts requires meticulous attention to achievement of a steady state, and the site of sampling. Multiple catheters may be used. Correct calculations require the inclusion of the dissolved oxygen into the calculation of the content of a blood sample, and measurement rather than estimation of oxygen consumption, this being especially important for cyanotic patients and candidates for Glenn or Fontan operations. 53,54 The widely used solution of cancelling out the consumption of oxygen from the corresponding equations leads to the fact that flow of blood to the lungs and pulmonary vascular resistance are put in relation to their systemic counterparts, which makes pulmonary vascular resistance a function of systemic vascular resistance. In this way, not only the individual measurements, but also comparisons between multiple catheterisations with the aim of comparing the effects of specific and pulmonary selective treatments, become unreliable.
The difference of mere pulmonary endothelial dysfunction, as opposed to fixed structural decrease and constriction of pulmonary vascular diameter, may be tested with inhalable or intravenous pulmonary vasodilators, providing maximum pulmonary vasodilation. Although, during the last 15 years, a multitude of substances and protocols have been developed for such evaluations and still exist, most institutions use a combination of oxygen and inhaled nitric oxide, nebulised iloprost, or combinations thereof. 55 If normal values are measured during baseline investigations or pulmonary vasodilation, then the surgical and peri-operative risk is assumed to be acceptably low. If, in the presence of increased values for pulmonary vascular resistance, the decrease of either pulmonary vascular resistance, mean pulmonary arterial pressure, or the ratio of pulmonary to systemic vascular resistance ratio exceeds 20%, the patient is considered a responder to pulmonary vasodilation, with the implication that the pulmonary vascular system still possesses endogenous vasodilatory capacity without perivascular fibrosis. This is associated with longer survival, and is an indication for treatment with calcium channel antagonists as an inexpensive therapeutic option. 56,57
Evaluation of Pulmonary Vascular Resistance Prior to Operative Intervention or Transplantation
In general, pulmonary congestion with cardiac failure in those with shunting defects within the first 3 months of life is taken by most as virtual absence of increased pulmonary vascular resistance. Absence of such an episode, or an age older than 12 months, indicates potentially increased pulmonary vascular resistance needing evaluation. The precise calculation of pulmonary vascular resistance is mandatory for patients considered for operations crucially relying on lowest possible pulmonary vascular resistance, such as creation of a Glenn shunt or conversion to the Fontan circulation. 58 As the results of operation and long-term follow-up depend so much on institutional skill and experience, clear data does not exist as a cutoff for when operation is possible as opposed to being contraindicated. It is customary to assume that values of less than 4 to 6 Wood units times m 2 body surface area, and ratios for pulmonary to systemic resistances from 0.25 to 0.3, indicate operability without the need for further testing using pulmonary vasodilators. Values of up to 7 to 10 Wood units times m 2 body surface area and ratios of resistances in the range of 0.4 to 0.5, are acceptable only if the testing with vasodilators achieves values unequivocally in the lower ranges given above. For those considered for Glenn or Fontan operations, still lower values are required, with less than 3 Wood units times m 2 body surface area and ratios of resistances of 0.2.
Treatment of Pulmonary Hypertension Associated with Congenital Cardiac Disease
Aside from the haemodynamic stressors, other factors such as arterial hypoxaemia in cyanosis, and hypercarbia in hypoventilation, as well as certain syndromes such as Down, Noonan’s, and Edwards’, are known to enhance or predispose for increased pulmonary vascular resistance. Whatever the precise pathogenetic stimulus, the pulmonary vasculature probably has a limited arsenal of different patterns to react to it. Hence, the result is a uniform change of cellular and histological appearance of the pulmonary vasculature, as delineated by Heath and Edwards 59 or Wagenvoort, 60 beginning with pulmonary endothelial dysfunction, leading to intimal and smooth muscle cell hypertrophy in a complex vascular-adventitial interaction, and eventually ending in complete obstruction of the pulmonary vessel due to the formation of plexiform lesions. The speed of the increase of pulmonary vascular resistance, together with volume loading of the pulmonary circulation, then provides for the macroscopic effects on pulmonary arterial diameter, right ventricular dilation and hypertrophy, and eventually reduction of myocardial contractility. This relative paucity of patterns of reaction is probably the reason that most forms of pulmonary hypertension do react to the current specific options for treatment, which merely replace pulmonary endothelial factors and favour general vasodilation. No drugs yet in routine clinical use provide inhibition or reversal of cell growth. Treatment of the pulmonary vascular resistance is indicated in all those situations where increased flow to the lungs would result in an improvement in delivery of oxygen, or where reduction of the afterload to the right ventricle would increase cardiac output and decrease right-sided cardiac failure. There are also clear contraindications. These include defects permitting large shunts without an increased, and hence protective, pulmonary vascular resistance, and therefore without reduced pulmonary perfusion. In this setting, further pulmonary vasodilation would increase the pulmonary over-circulation and lead to cardiac failure. Pulmonary venous obstructive disease is another contraindication. Because the pulmonary venules in this setting are lined with obstructing non-dilating endothelial cells not reactive to pulmonary vasodilators, pulmonary vasodilation would only inhibit the protective pulmonary arterial capillary vasoconstriction, and thus lead to pulmonary oedema. Hypoplasia or reduced diastolic or systolic function of the left ventricle constitutes a further contraindication, because pulmonary vasodilation would overcome the protective increase in pulmonary vascular resistance and again lead to pulmonary oedema.
Neonatal Pulmonary Hypertension Due to Congenital Cardiac Disease
In some neonates, the high fetal pulmonary vascular resistance does not rapidly fall as is usually the case at birth. This may be due to endothelial and smooth muscle injury as a result of co-existent cardiac anomalies and increased flow of blood to the lungs. The resulting endothelial shear stress may cause alterations of the signaling pathways involving nitric oxide and endothelin, which precede anatomical changes and pulmonary vascular remodeling. In addition, changes in mediators such as transforming growth factor–beta, vascular endothelial growth factor, and vascular potassium channels, as well as upregulation of collagens, have been noted in both animal models and children with congenital cardiac disease with increased flow of blood to the lungs.
Pulmonary hypertension in the presence of large unrestricted shunting in the setting of lesions such as the persistently patent arterial duct, transposition, and common arterial trunk is simply a consequence of communicating tubes, and will be corrected only by surgical treatment. There are a few lesions such as pulmonary hypertension and cyanosis in transposition or Ebstein’s malformation, where the use of inhaled nitric oxide may be indicated pre-operatively to enhance the flow to the lungs, enhance oxygenation, and normalise future post-operative right ventricular afterload. Surgical creation of an aortopulmonary shunt may be more effective if the increased pulmonary vascular resistance is due to general pulmonary vascular hypoplasia. 61 In general, the earlier the indicated surgery is performed, the less persistent will be the damage to the pulmonary endothelium and vascular tree. Besides timing, surgical and neonatal intensive care experience will decide on the need for a corrective as opposed to a palliative approach. In doubtful cases, leaving or creating an atrial shunt to bridge the peri-operative period may be useful, the shunt being closed interventionally at a later date.
Acute Post-operative Pulmonary Hypertension
This transient clinical and pathophysiological condition can result from cardiopulmonary bypass surgery. The activation of inflammatory pathways occurs in all organs, with specific problems in each. In the lung, it leads to, and augments, any pre-existing pulmonary endothelial dysfunction. Especially in neonates and infants, but also in larger children, important bronchomechanical interactions exist so that the fluctuations in pulmonary vascular resistance post-operatively have a direct impact on lung compliance and gas exchange. In the high-risk patient, these make the meticulous monitoring of both haemodynamic and pulmonary mechanical parameters mandatory so as to achieve optimal clinical management.
Following cardiopulmonary bypass operations, the pulmonary vascular system experiences for a few days or longer an increase in resistance and sensitivity to vasoconstrictive stimuli. The resistance can be lowered by supplementation of elements from the l -arginine–nitric oxide pathway, and by blockade of the endothelin receptors, suggesting a combined pathophysiology of different pathways as a cause. This pathophysiology persists for several days, making the lung very vulnerable to different stimuli such as handling, suctioning, movement, and hypoventilation. Such stimuli are answered within seconds with an exponential, and very dangerous, life-threatening pulmonary vasospasm. This is the so-called pulmonary hypertensive crisis, which results in a sharp increase in pulmonary vascular resistance and pulmonary arterial pressure, acute right ventricular decompensation with raised central venous pressure, decreased pulmonary blood flow with cyanosis, and left atrial and systemic hypotension ( Fig. 56-2A ). In infants and young patients, this phase is directly associated with a decrease in lung compliance (see Fig. 56-2B ), hypoventilation, hypercarbia, and further pulmonary vascular restriction, producing a vicious circle which can only be interrupted by manual forced and energetic hyperventilation, and which may require other immediate measures aimed at resuscitation. This bronchopulmonary interaction has also been described elsewhere, such as in the variation of both pulmonary compliance and flow of blood to the lungs, and asthma in pulmonary hypertension.

The incidence of life-threatening pulmonary hypertensive crises has markedly decreased, from around 20% of cases in the 1980s to only 1% to 2% 25 years later. This reflects better techniques for surgical repair, cardiopulmonary bypass, post-operative handling, mechanical ventilation, and most importantly, timely operation before the sixth month of life. The pathophysiology nevertheless remains relevant for complex patients, particularly for those with the Fontan circulation. While the basic strategies for avoidance include alkalotic hyperventilation, sedation and paralysis, and sometimes also hypothermia, advanced strategies such as extracorporeal membrane oxygenation are only indicated for bridging a situation that may be expected to recover. Such situations are the postoperative inflammation-related increase of pulmonary vascular resistance, and depressed left or right ventricular function. Other strategies include negative pressure ventilation, ventilation with nitric oxide, and use of specific pulmonary antihypertensive drugs, such as the intravenous or inhaled use of the nucleotide adenosine, the phosphodiesterase 5 inhibitor sildenafil, the calcium channel sensitiser levosimendan, and the endothelin receptor antagonist tezosentan.
Chronic Post-operative Pulmonary Hypertension
The patient in this setting will have a surgically corrected, normal circulation without significant residual shunting defects or obstructions. Pulmonary vascular resistance is increased most likely due to the persisting pre-operative pulmonary vascular damage, as well as the effects of cardiopulmonary bypass surgery. Any left-sided component, such as pulmonary venous stenosis, mitral valvar obstruction or regurgitation, or left ventricular restriction, should clearly be recognised as such, and may warrant cardiac catheterisation to reveal the attributable increase in pulmonary vascular resistance, thus differentiating the increase from the independent overshooting reaction of the arterial pulmonary vascular tree, with the latter potentially accessible to pharmacological treatment.
It is important to make sure that patients and physicians understand that this form of pulmonary hypertension is not idiopathic. It may be stable, regress over time, or progress gradually and then require careful monitoring of cardiac function, which may be more vulnerable because of the surgical repair. The strategies for such treatment are similar to those for idiopathic pulmonary hypertension. This includes the use of anticoagulation, calcium channel blockers, balloon atrioseptostomy, and all specific drugs including phosphodiesterase 5 inhibitors, endothelin receptor antagonists, and intravenous epoprostenol. The reaction to these drugs is probably similar.
Pulmonary Hypertension Due to Left-Sided Cardiac Anomalies
Left-sided obstructions to flow of blood from the lungs includes pulmonary venous stenosis, divided left atrium, mitral valvar stenosis or regurgitation, and left heart systolic or diastolic dysfunction, either without or due to aortic stenosis or coarctation. The increased pressure upstream from the obstruction causes dilation of the involved structures, in other words, the pulmonary veins. Increases in pulmonary venous pressure directly translate into increases of pulmonary arterial pressure, while the transpulmonary gradient, and thus the calculated pulmonary vascular resistance, remains low. Invasive measurement of pulmonary venous pressure or left ventricular end-diastolic pressure, respectively, is necessary, and then calculation of the transpulmonary gradient and pulmonary arterial vascular resistance.
If the lesion is longstanding, the arterial side of the pulmonary vascular tree will react to this increased pressure and develop increased pulmonary vascular resistance via the cellular mechanisms described for idiopathic pulmonary hypertension. This may overshoot and increase out of proportion. The continuing increase of pulmonary vascular resistance will cause an increase of right ventricular diameter, with septal shift into the left ventricle, and reduced cardiac output due to right ventricular failure as described for idiopathic pulmonary arterial hypertension.
Treatment of this form of pulmonary hypertension is only warranted if the primary condition is optimised as far as possible, and invasive demonstration has shown, first, that cardiac output is reduced due to increased pulmonary vascular resistance and, second, that reduction of pulmonary vascular resistance results in improved cardiac output without any increase in pulmonary venous pressure. If the left-sided obstruction is relieved temporarily or chronically by pharmacologic treatment, corrective surgery, or assist devices, the pulmonary vascular resistance may normalise instantly or over a longer time span. In patients with end-stage left-sided cardiac failure, the long-term administration of inotropic agents, or the implantation of ventricular assist devices, to off-load the left ventricle is an accepted and often successful option to permit normalisation of the increased pulmonary vascular resistance and allow for transplantation of the heart in isolation. Unfortunately, the pulmonary hypertension due to pulmonary venous stenosis or mitral valvar disease may persist tenaciously due to the impossibility of completely relieving the lesion surgically or interventionally, this being a far from rare situation.
Fontan Circulation
The serial Fontan circulation amplifies the interdependence of all its circulatory elements. The flow of blood into the thorax and pulmonary vasculature is augmented during inspiration by negative intrathoracic pressure, while the flow of blood out of the thorax is supported by the diastolic function of the systemic ventricle. For both of these mechanisms to function, it is vital to retain as low a pulmonary vascular resistance as possible. Even a mild increase in resistance has an appreciable influence on the Fontan circulation, and leads to its failure. Several factors, with some of them being part of the Fontan state itself, may lead to an increase of pulmonary vascular resistance and reduction on the flow of blood to the lungs.
Anatomical and Mechanical Factors
These include small pulmonary arteries, the presence of stenoses, discontinuous pulmonary arteries, and distal abnormalities of arborisation, all of which increase the pulmonary vascular resistance. The detrimental effect of paralysis of the diaphragm on the dynamics of flow in the Fontan circulation has been clearly demonstrated.
Pathophysiological Factors
Too little flow, or too much flow, can result in either a hypoplastic pulmonary arterial tree or, as a reaction to the additional left-to-right flow, an increased medial thickness of the pulmonary arterioles. Both factors elevate pulmonary vascular resistance, and both have an unknown chronic time course.
Pulmonary Arterio-venous Malformations
These channels occur in around one-sixth of patients, bypassing the exchange of gases by connecting the arterial side directly to the venous side of the pulmonary vasculature. These vessels may be large and allow a right-to-left shunt at low resistance as cyanotic admixture to the systemic blood in patients with increased pulmonary vascular resistance, or in those with previous Glenn operations, where the flow of venous blood from the lower body, including the hepatic contribution, remains connected to the heart, thus bypassing the lung.
Pulsatility of Pulmonary Flow and Pulmonary Endothelial Dysfunction
Pulsatility is greatest in those patients having an atriopulmonary connection. In contrast, the pulsatility is almost lacking in patients with a lateral tunnel or total cavopulmonary connection, causing persistent pulmonary endothelial dysfunction, which can be shown by inhalation of nitric oxide to provoke a fall in pulmonary vascular resistance. 62 Even in those with pulsatile flow, the mean pulmonary arterial pulse pressure is lower than normal.
Reduced Growth of the Pulmonary Arteries
There is insufficient growth of the pulmonary arteries in relation to growth of body surface area over an observation period of 3 to 5 years. This may be due to early reduction of volume from staged cavopulmonary anastomosis to prevent ventricular volume overload and preserve systemic ventricular function. The reduction of pulmonary flow, and early introduction of non-pulsatile flow in the pulmonary circulation, may also affect the growth of the pulmonary arteries.
Micro- and Macro-thrombuses in the Pulmonary Circulation
For several reasons, the Fontan patients are at increased risk for the formation of silent or clinically apparent macro- and micro-thrombus, namely, the low, non-pulsatile systemic venous flow, the thrombogenic material of the conduit itself, and altered hepatic function due to the Fontan state. 63 The threat of pulmonary thromboembolism to a pulmonary circulation with already decreased overall diameter is clear from the experience of increased mortality in such patients with idiopathic pulmonary hypertension, and justifies effective anticoagulation.
Reduced Ventricular Function after the Fontan Operation
The acute reduction in preload resulting from the Fontan operation leads to an increase in the ratio of ventricular mass to volume ratio, with concomitant impaired ventricular relaxation and patterns of filling. 64 Although this hypertrophy has been shown to regress 1 to 3 years after surgery, the abnormal ventricular relaxation and reduced ventricular compliance persist over the long term. A progressive increase in ventricular stiffness, which naturally occurs with age, could also potentially contribute to a steady rise in pulmonary venous pressure, with ensuing increase of pulmonary vascular resistance.
In the attempt to modulate therapeutically pulmonary vascular resistance in patients with the Fontan circulation, both inhaled nitric oxide and the phosphodiesterase inhibitor sildenafil have been used successfully in the acute post-operative setting. Sildenafil was also used for chronic problems like protein-losing enteropathy, 65 improving diastolic mesenteric blood flow, exercise tolerance, pulmonary arterial pressure and pulmonary vascular resistance, and plastic bronchitis, with similar effects as the use of fenestrated stents. 66 Improvement was also reported after treatment with bosentan, an antagonist of endothelin receptors. Although the occurrence of these complications over the long term is correlated in some but not all patients with an increase in pulmonary vascular resistance and central venous and pulmonary arterial pressures, the clinical success of targeted pharmacological modulation of the pulmonary circulation indicates that there may be a role for such treatment on a broader basis, if not even in an anticipatory prophylactic way.
Eisenmenger Syndrome and Its Pathophysiology
Anatomy
The strict definition of Eisenmenger’s syndrome as severe systemic cyanosis due to reversal of the shunting at atrial, ventricular or great arterial level theoretically excludes the settings of common atrium and anomalous pulmonary venous drainages, functionally single ventricle, and common arterial trunk, as the cyanosis in these lesions is not a result of shunt reversal, but of obligate intracardiac mixing of desaturated and oxygenised blood. Such considerations are important for proper management. Practically however, the working definition does include the latter diagnoses, and implies inoperability due to suprasystemic pulmonary vascular resistance. 67,68
The natural history of Eisenmenger’s syndrome is quite variable, but eventually leads to severe hypoxaemia, cyanosis, and cardiac failure. In one study, half of a cohort of patients with unrepaired ventricular septal defect was alive 20 years after the diagnosis was made. 69 Other symptoms include progressive shortness of breath, polycythaemia, headache, and haemoptysis. Therapeutic options are limited, and include supplemental oxygen therapy, anticoagulation, vasodilator therapy, and endothelin receptor antagonists. 70–76 There is some controversy about the impact of the drugs. Lung and heart-lung transplantation may be offered with an acceptable risk, and a favourable long-term outcome. 77 Oral contraceptives should be prescribed for females, as pregnancy in such patients has a high incidence of maternal and perinatal death. 78
While the process is usually interrupted with early surgical correction of the shunting lesion, it may progress in those undergoing late correction. In such cases, a haemodynamic situation similar to idiopathic pulmonary arterial hypertension will result. In cases of significant residual defects, right-to-left shunting can occur, with establishment of the syndrome in a patient who earlier underwent successful surgical correction in the absence of pulmonary vascular disease.
Pathophysiology
The effect of the shunt depends on its exact location and size. This can cause different loading conditions, both to the pulmonary vascular tree and the subpulmonary ventricle, with specific morbidity and mortality.
Atrial Level
The defects with shunting at atrial level deliver large volumes of blood without pressure, causing shear stress to the pulmonary endothelium. In the case of abnormally draining pulmonary veins, the shunt is fixed by the abnormal connection, and only indirectly reflects increasing pulmonary vascular resistance by a reduction of pulmonary flow. In the case of interatrial communications, the left-to-right shunt correlates with the strength of right ventricular systolic opening pull, and right atrial and ventricular diastolic compliance. Due to the continuous venous flow, the shunted volume can be huge, and causes right ventricular dilation. The increase of the pulmonary vascular resistance in the third and fourth decades stretches still further the right ventricular myocytes so that they do not cope well with the increasing afterload, and tend to fail early. The Eisenmenger pathophysiology with cardiac cyanosis is then a result of the atrial right-to-left shunting due to right ventricular failure, and only indirectly due to increased pulmonary vascular resistance.
Ventricular Level
These defects deliver volume and, depending on their restrictive or unrestrictive size, also left ventricular pressure. In systole, and only then, the direction and volume of the shunt is directly ejected into the great arteries, and depends on the ratio of pulmonary to systemic resistance and the ejecting power of the ventricles, ending with closure of the arterial valves. In early and late diastole, there may be different directions and volumes of the shunt depending on ventricular compliance, thus explaining the angiographic and haemodynamic findings of left-to-right shunting during systole and right-to-left shunting in diastole in the presence of a hypertrophied and stiff right ventricle. In an atrioventricular septal defect, the addition of atrial shunting contributes also a volume load, causing ventricular dilation.
In the case of a large defect, the high pressure postnatally maintains a high, near-systemic pulmonary vascular resistance, avoiding severe cyanosis or loud heart sounds, but also avoiding a period of cardiac failure which would stimulate a diagnosis. The cyanosis may not be marked, and may be discovered only later, when pulmonary vascular resistance becomes suprasystemic. Both right and left ventricles deliver blood to both great arteries and thus see the same afterload and work as one unit. 79,80 The peripheral saturation of oxygen is a function of the ratio of pulmonary to systemic flows. Pharmacologically induced pulmonary vasodilation to augment pulmonary venous return may counteract severe cyanosis, but may be hazardous to the oft-compromised myocardial function.
Arterial Level
In the setting of transposition physiology, cyanosis is due to interatrial and intraventricular streaming and morphology, which impairs mixing so that desaturated systemic venous blood enters the subaortic ventricle or the aorta, and pulmonary venous blood returns to the subpulmonary ventricle or the pulmonary artery while pulmonary vascular resistance is low. Saturation can then only be increased anatomically by either separating the atriums in the case of common atrium and anomalously connected pulmonary veins, or in the case of transposition, by creating a left-to-right shunt at atrial level.
All lesions with arterial shunting deliver the shunt flow at systemic pressures to the pulmonary vascular tree continuously during both systole and diastole. They are associated, therefore, with an early and aggressive rise in the pulmonary vascular resistance. Below a certain size, the exact diameter of the defect does matter, and the Eisenmenger syndrome will not be manifest. That threshold appears to be from 2 to 3 cm at atrial level, limiting the ratio of pulmonary to systemic flows to four- to sixfold, and from 1 to 1.5 cm at ventricular level, limiting the ratio to three- or fourfold. The size at arterial level is from 0.5 to 0.7 cm. If the septum is almost entirely missing, as in common atrium, and in those with functionally single ventricles or common arterial trunk, there is complete mixing of the venous and arterialised blood. The resulting saturation is a function of the ratio of pulmonary to systemic flows, and is equal in both great arteries, with univentricular cardiac function. Uncorrected defects of medium size, or residual defects which are neither restrictive nor univentricular, produce a classical Eisenmenger syndrome, where the degree of cyanosis depends on pulmonary vascular resistance.
Differences between Idiopathic and Congenital, Cardiac-Disease–associated Pulmonary Arterial Hypertension
Known Trigger
In contrast to those with idiopathic pulmonary arterial hypertension, the cause for the development of increased pulmonary vascular resistance in patients with congenitally malformed hearts is well known and monitored. In some groups, however, the increase in pulmonary vascular resistance seems to be out of proportion to the stimulus, such as in children with small, persistently patent arterial ducts or insignificant interatrial communications, and in those who have undergone timely correction of transposition. 81–83 It is as yet unclear whether these patients have a latent form of idiopathic pulmonary arterial hypertension, which was triggered by these defects.
Different Dynamics
Those with idiopathic pulmonary arterial hypertension have a broad range of age, with the problem occurring as late as the seventh decade, or as early as the first months of life. The paediatric form shows an aggressive course, which only recently has been modified by available pharmacological treatment. 84–86 In contrast, the development of increased pulmonary vascular resistance in those with congenitally malformed hearts is more predictable, being avoidable by surgical correction at less than 6 months of life, and showing stability over decades in those with the Eisenmenger syndrome.
Right Ventricular Function
When given time, the morphologically right ventricle can adapt to a large extent to increased afterload, and will maintain cardiac output until contractility decreases, at which time the ejection period, stroke volume, and peak systolic pressure correlate with contractility. Morbidity and increased mortality 87–89 are seen when the ventricle is congenitally malformed or surgically altered, with direct effects on the pattern of contraction.
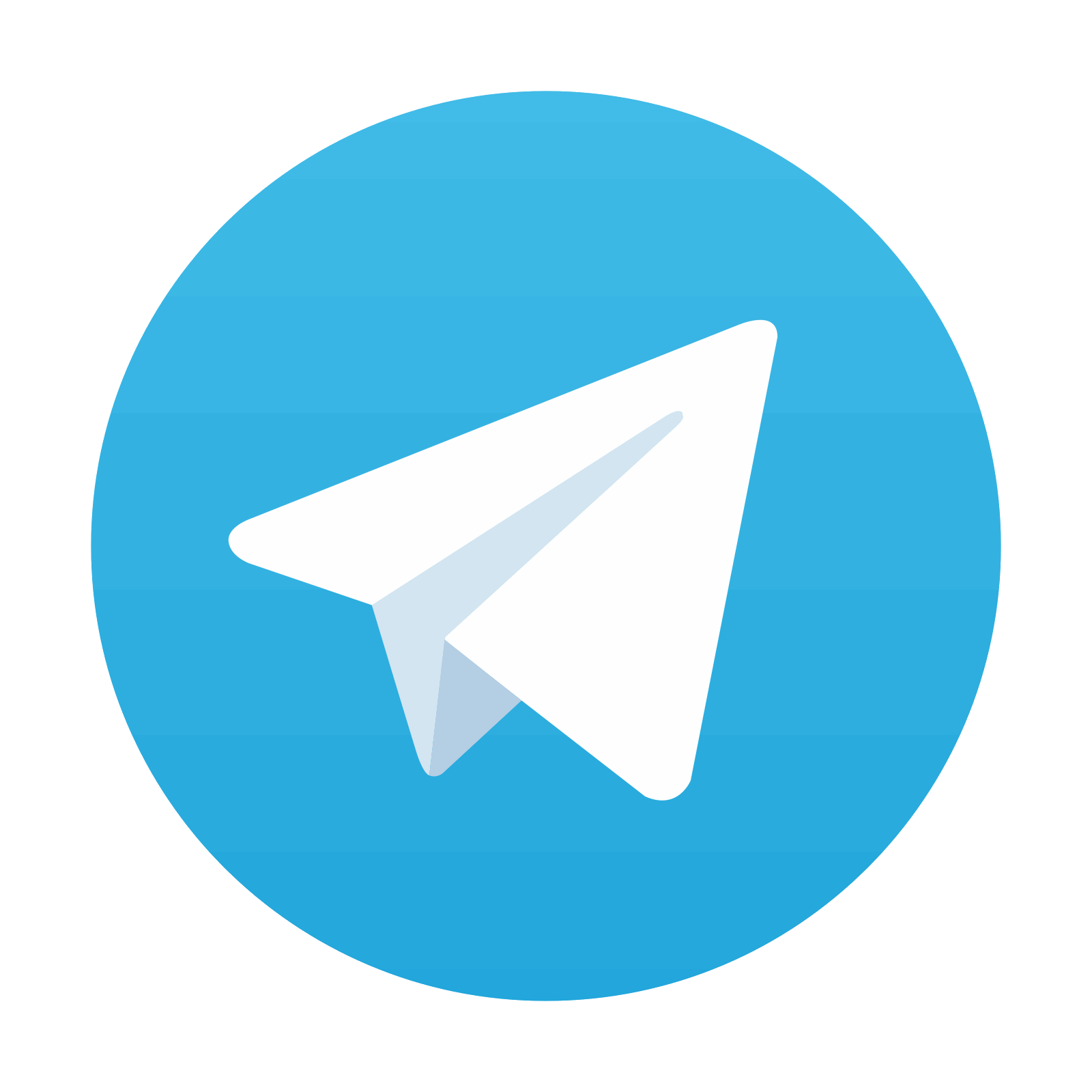
Stay updated, free articles. Join our Telegram channel

Full access? Get Clinical Tree
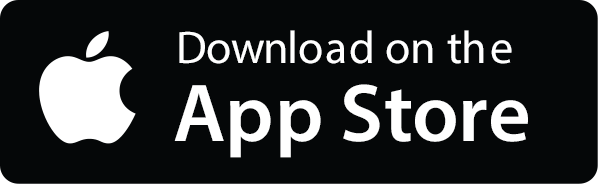
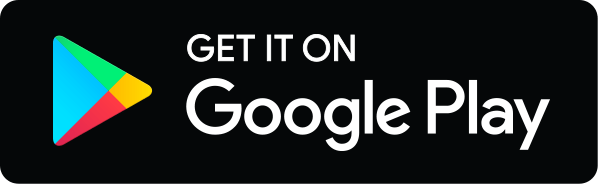