Computed tomography image of a patient with pediatric acute respiratory distress syndrome, demonstrating heterogeneous lung disease with dependent atelectasis
Placing adults with acute hypoxemic respiratory failure in the prone position has been reported to improve oxygenation as early as 1976 [2]. Since then, many studies have confirmed improved oxygenation, but meaningful outcomes such as improved survival and ventilator-free days have been inconsistently reported. The prone position was first reported to improve oxygenation in a case series of children with pediatric ARDS (PARDS) in 1994. Children in this report had improved oxygenation after spending only 30 minutes in the prone position.
In the early 2000s, prone positioning became widely studied as a potential therapy for PARDS. Curley, Kornecki, Bruno, and Casado-Flores all published studies of children with PARDS [3–6]. These studies were all small, single-center trials with various study designs, including differing inclusion criteria and protocols for use of prone position. In all of them, children served as their own controls, comparing oxygenation markers between prone and supine positions. They all showed consistent findings of improved oxygenation with the use of the prone position. Children in these studies who were maintained in the prone position for 8–12 hour cycles had a response rate ranging from 78% to 90% [3, 4, 6]. Another consistent finding in these studies was the safety of prone positioning, demonstrating extremely low rates of adverse events in placing patients in the prone position. Additionally, no specialized equipment or drug is required, making the cost-benefit ratio in the use of prone position highly favorable.
Because of this, a large multicenter randomized controlled trial was undertaken by Curley et al. [7]. In this study, patients with acute lung injury, defined by PaO2/FiO2 ratio less than 300 mm Hg, were randomized within 48 hours to standard supine positioning or prone positioning for 20 hours daily. Similar to the previous smaller trials, 90% of prone patients in this trial responded with improved oxygenation. Despite this, the study was stopped on interim analysis due to finding that outcomes did not differ between study groups. Ventilator-free days, mortality, and cognitive function, among other outcomes assessed, were not improved by use of the prone position despite its apparent effect on improved oxygenation. Of note, use of the prone position was demonstrated to be generally safe in this relatively large trial [8].
In contrast to studies of pediatric patients, some trials of adult patients have demonstrated improved outcomes in addition to improved oxygenation. A 2008 meta-analysis of 1559 patients with acute hypoxemic respiratory failure again showed that prone position is associated with improved oxygenation, but not with improved outcomes such as improved survival [9]. However, in a follow-up subanalysis of 555 patients with severe hypoxemia, defined as a PaO2/FiO2 ratio less than 100 mm Hg, a survival benefit was demonstrated [10].
In 2013, Guérin et al. reported the results of the largest multicenter randomized controlled trial of prone positioning in adults with severe ARDS, known as the PROning SEVere ARDS patients (PROSEVA) trial [11]. Severe disease was defined by PaO2/FiO2 ratio less than 150 mm Hg with an FiO2 of at least 0.60 and a PEEP greater than or equal to 5 cm H2O. Four hundred sixty-six patients were randomized to standard supine position or prone position for 16 hours daily. All-cause 28-day mortality in patients randomized to the prone arm was 16%, compared to 33% in patients randomized to the supine arm (hazard ratio, 0.39; 95% CI, 0.25–0.63; p < 0.001). This 50% relative risk reduction in mortality suggests a massive potential benefit in adults with the most severe disease. Extrapolation of this result to pediatric patients is questionable and may be answered by the ongoing PROSPECT study. Even without such data, experts from the Pediatric Acute Lung Injury Consensus Conference (PALICC) stated that, while prone positioning “cannot be recommended as routine therapy in PARDS…it should be considered an option in cases of severe PARDS” [19].
The optimal protocol for prone positioning to maximize its benefit is unknown. Improvements in oxygenation are seen quickly. However, a retrospective analysis in 2003 showed oxygenation was improved more in patients who were kept in the prone position for a prolonged period of 18–24 hours compared to a short period of 6–10 hours. Additionally, the improvement in oxygenation was more stable after 12 hours in the prone position [12]. The PROSEVA trial demonstrated improved outcomes with 16 hours per day in the prone position, while the study in pediatric patients by Curley et al. showed improved oxygenation but not improved outcomes using 20 hours per day in the prone position. The difference in the results of these studies is likely due to differences in inclusion criteria (only severe patients in the PROSEVA trial), or differences between adult and pediatric patients, and/or differences in concurrent therapies, but duration of the use of prone positioning is theoretically a factor.
Another consideration in use of the prone position is the way in which the patient is turned. Specialized beds are available for use in adults that can be used in larger pediatric patients. These beds use continuous rotation of the prone patient from side to side up to 60° in either direction, termed continuous rotational therapy. While not extensively studied, this method shows comparable improvements in oxygenation to prone positioning in a standard bed, but may have adverse hemodynamic effects [13]. Care of larger patients in these beds may be perceived to be easier; however, they may substantially increase cost and expertise required in order to utilize the prone position. Of note, patients in the PROSEVA trial were placed in the prone position in their standard ICU bed.
In summary, prone positioning clearly can improve oxygenation in a vast majority of patients with acute hypoxemic respiratory failure. Improvements in other outcomes, such as improved survival, are questionable in pediatric patients. Patients with severe PARDS may have improved benefits compared to those with less severe disease, and further study is warranted (and ongoing) in this population. Due to the highly favorable safety record of prone position in prior trials, fear for patient safety or patient care limitations should not limit its use. While prone positioning cannot be recommended as standard of care, its use should be considered in cases of severe PARDS, especially given the low cost and potential improvements in patients with severe disease.
Pulmonary Vasodilators
The vascular endothelium synthesizes nitric oxide (NO) by the action of NO synthase on the precursor L-arginine. NO diffuses into an adjacent vascular smooth muscle cell, where it directly stimulates soluble guanylate cyclase (sGC). NO has local action only, as it undergoes rapid oxidative inactivation in the serum. Guanylate triphosphate is converted to cyclic guanylate monophosphate (cGMP) by sGC, which exerts a vasodilatory effect by indirectly blocking calcium influx into the smooth muscle cytoplasm. cGMP is inactivated by phosphodiesterase-5 (PDE5).
Prostaglandin I2 (PGI2) is also synthesized in the vascular endothelium, via the arachidonic acid – cyclooxygenase pathway. It then activates adenylate cyclase on the smooth muscle cell membrane, converting adenylate triphosphate to cyclic adenylate monophosphate (cAMP). cAMP acts through protein kinase A to lead to smooth muscle relaxation. cAMP is inactivated by phosphodiesterase-3 (PDE3).
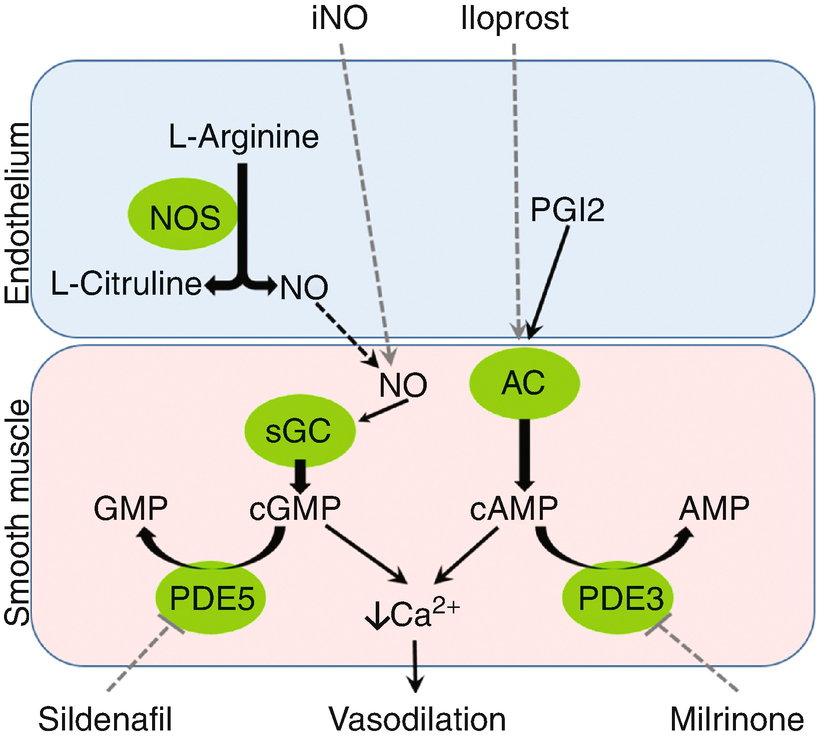
Physiology and pharmacology of pulmonary vasodilation. iNO inhaled nitric oxide, NO nitric oxide, NOS nitric oxide synthase, PGI2 prostaglandin I2, AC adenylate cyclase, sGC soluble guanylate cyclase, cGMP cyclic guanylate monophosphate, cAMP cyclic adenosine monophosphate, PDE5 phosphodiesterase 5, PDE3 phosphodiesterase 3
Inhaled Nitric Oxide
Inhaled nitric oxide (iNO) has been considered a potentially ideal pulmonary vasodilator in use for PARDS due to its local action in well-ventilated portions of the lung. Since it is inhaled, it will exert maximal effect in the best ventilated regions of the lung, and little-to-no effect in poorly ventilated regions. This will cause blood to shunt away from poorly ventilation regions to well-ventilated regions, and ultimately improve ventilation-perfusion matching. The net effect of this is overall improved gas exchange, leading to improved oxygenation. Therefore, iNO has been used in PARDS to improve oxygenation, with the hope of ultimately having a favorable affect on the outcome of the disease. Standard dosing for iNO is up to 20 parts per million (ppm), although doses as low as 1 ppm may be effective in improving oxygenation [13, 14].
After several case series reported rapid improvements in oxygenation with the use of iNO in patients with PARDS, three randomized controlled trials were performed. In 1997, Day et al. demonstrated improvements in oxygenation compared to control in a small study, which was not designed to assess mortality [15]. Then, in 1999, Dobyns et al. reported a larger, multicenter randomized controlled trial of children with severe acute hypoxemic respiratory failure (with oxygenation index >15) [16]. One hundred eight children were randomized to iNO at 10 ppm or a placebo-control. This trial confirmed the beneficial effect of iNO on oxygenation, but survival did not differ between groups. Finally, Ibrahim et al. reported in 2007 a smaller trial of 32 patients with severe PARDS randomized to iNO in prone position, iNO in supine position, or without iNO in prone position. This again confirmed a benefit on oxygenation but not in other outcomes such as mortality. More recently, Bronicki et al. reported on 55 children from 9 centers with PARDS and showed that ECMO-free survival was more common in the treated group (92% vs. 52%, p < 0.01), though overall survival was not.
A Cochrane meta-analysis was published in 2011 including pediatric and adult patients [17]. Improved oxygenation was confirmed, with no improvement in survival, duration of mechanical ventilation, length of ICU stay, or any other outcome. A concerning finding of increased incidence of renal impairment was found in patients treated with iNO.
Despite the improvement in oxygenation, there is no conclusive evidence to support the use of iNO for patients with PARDS given the lack of consistent improvement in patient outcomes. However, as indicated in a consensus statement from the European Society for Pediatric and Neonatal Intensive Care, its use can be considered in patients with pulmonary hypertension or severe right ventricular dysfunction [18]. iNO use may be beneficial in this population not solely due to its effect on oxygenation, but rather its effect on decreasing pulmonary vascular resistance and therefore improving cardiac output and/or decreasing right ventricular strain. The benefit of iNO on mortality remains unproven in this scenario; however, there is sufficient theoretical benefit to consider its use. More research is needed to further guide therapy decisions in patients with PARDS and pulmonary hypertension or severe right ventricular dysfunction.
As extracorporeal membrane oxygenation (ECMO) increases in use in severe PARDS, the role of iNO to rescue from ECMO or bridge to ECMO remains unstudied. Because of the lack of evidence, experts from PALICC concluded that iNO could be considered in severe PARDS as a rescue from or bridge to ECMO [19].
Because of the considerable cost and potential toxicities associated with iNO use, a cost-benefit analysis should be carefully considered before initiating therapy. Once used, an assessment of clinical benefit should be undertaken, and steps should be made to wean and discontinue it as soon as possible [19]. Renal function should be carefully monitored especially with concurrent use of nephrotoxins. While methemoglobinemia is a potential concern at very high doses of iNO, at standard doses of iNO less than or equal to 20 ppm, it is a rare occurrence and routine lab monitoring for this toxicity with co-oxymetry may not be necessary [20].
Inhaled Prostaglandin Therapy
Inhaled PGI2 can be considered similarly to iNO [21]. As stated above, PGI2 causes pulmonary vasodilation, although via a different pathway from iNO. Since it is inhaled, it has many of the same potential benefits of improving ventilation-perfusion matching in patients with PARDS. It has not been studied sufficiently to make claims on outcomes, but there is no reason to believe it would differ substantially from iNO. While the cost of its use may be favorable to the cost of using iNO, there is much less experience with its use. At this time, its use in the management of PARDS is not recommended [19]. Until further evidence is available, inhaled PGI2 could be considered to likely have similar effects to iNO.
Systemic Pulmonary Vasodilators
Systemic medications such as PGE5 inhibitors, PGE3 inhibitors, and intravenous prostaglandins can be used as pulmonary vasodilators, but should not be considered specifically to treat PARDS. Their use for pulmonary hypertension or severe right ventricular dysfunction may be warranted based on the management strategies for those conditions. However, in PARDS, the widespread pulmonary vasodilation in the setting of heterogeneous ventilation has the potential to worsen ventilation-perfusion matching and worsen, or at least not improve, oxygenation [22].
Inflammatory Modulation
Glucocorticoids
Glucocorticoids are drugs which exert various actions through binding with the glucocorticoid receptor, having a similar effect to the naturally occurring hormone cortisol. Commonly used glucocorticoids include hydrocortisone, prednisone, methylprednisolone, and dexamethasone, among others. They are commonly used in many aspects in medical care as broad-spectrum anti-inflammatory drugs. Their use in PARDS has been considered because of the tremendous inflammation present in the disease.
The systemic use of glucocorticoids has been studied more in adults with ARDS than in children. Two meta-analyses have been published using different methodologies to evaluate the current available evidence in trials of glucocorticoids for adults with ARDS [23, 24]. In the first, no definitive role of glucocorticoids for ARDS was established [23]. In the second, an association between glucocorticoid use and improved survival was only found when combining randomized controlled trials (four trials, n = 341) with cohort studies (five studies, n = 307). With this full analysis, glucocorticoid use had a relative risk of mortality of 0.62 (95% CI, 0.43–0.91) [24]. However, combining the various study types into one analysis introduces more risk for confounding or bias in the results. Therefore, there is still no clear determination of the role of glucocorticoids for adults with ARDS. Evidence quality for glucocorticoid use in pediatric patients is poor, consisting only of case reports and case series [19].
Treatment protocols studied have varied by the drug used, the dose, the duration, the use of dose tapering, and the timing during the disease process. This variation further complicates the analysis of the efficacy of glucocorticoids for ARDS [24]. While meta-regression analysis of these types of variations in treatment strategy did not detect differing efficacy, power was insufficient to detect small or even modest effects in many of the variables.
Further study is certainly needed to determine the treatment effect as well as optimal dosing strategies and patient selection for glucocorticoid use in PARDS, and their routine use is not recommended [19]. If used, a low dose (equivalent of methylprednisolone 2 mg/kg/day) and short duration (total 7 days of therapy with or without a dose taper) may be sufficient to achieve the potential benefit while minimizing potential adverse effects.
Etanercept
Tumor necrosis factor-α (TNF-α) is an important pro-inflammatory mediator in many diseases, including PARDS. The idiopathic pulmonary syndrome (IPS) in patients following hematopoietic stem cell transplant is one disease in which high levels of TNF-α are found in the serum and broncho-alveolar lavage fluid. Because of this finding, interest was generated for the potential use of etanercept (a soluble TNF-α-binding protein, which inactivates TNF-α) in IPS. In uncontrolled trials, a combination of etanercept and corticosteroids has been associated with improved survival in patients with IPS compared to historic controls [25, 26]. Response rates were highest when the therapy was initiated before mechanical ventilation was initiated, indicating that timing of inflammatory mediation has an important role in its efficacy. A randomized controlled trial of this therapy in adults with IPS showed a 17% absolute increase in survival, but this did not reach statistical significance as the trial was unfortunately stopped early due to slow subject accrual [27]. The available evidence suggests etanercept is a promising therapy for this specific syndrome. This and other methods of specific immunomodulation could potentially impact the course of PARDS in other patient populations as well, but the utility remains to be determined and this use should currently be reserved for the research setting.
Exogenous Surfactant
The use of exogenous surfactant in the neonatal respiratory distress syndrome has revolutionized the care of babies born extremely prematurely. As its use has become standard of care in this population, outcomes for these patients have dramatically improved. Because of this observation, it is tempting to try to extrapolate this strategy to older children with severe hypoxic respiratory failure.
While neonatal respiratory distress syndrome is characterized by surfactant deficiency due to immature type 2 alveolar cells not producing it, the acute respiratory distress syndrome may have qualitative defects of surfactant due to the inflammatory pathophysiology [28–30]. After case reports and case series of surfactant use in PARDS showed promise for the benefit of this therapy, surfactant use was studied extensively in clinical trials. An early open-label, uncontrolled observational trial of calf lung surfactant extract (calfactant) conducted by Willson et al. in 1996 demonstrated a dramatic improvement in oxygenation in children with acute hypoxemic respiratory failure [31]. While this was an uncontrolled trial, the mortality among the 29 subjects was only 14%, which was better than survival estimates.
Subsequently, several small randomized controlled trials were conducted on children with respiratory failure. In two trials of surfactant use in children with bronchiolitis requiring invasive mechanical ventilation, Luchetti et all showed that porcine surfactant (curosurf) use improved oxygenation and ventilator parameters, and shortened ventilator durations and PICU stays [32, 33]. All patients in both of these trials survived their illness. In a more severely ill cohort of patients with acute hypoxemic respiratory failure, Willson conducted a prospective randomized controlled trial of 42 subjects, also showing improved oxygenation and shortened ventilator duration and PICU length of stay [34]. Finally, a multicenter trial of 35 children conducted by Möller et al. again showed improvement in oxygenation with surfactant use, along with a nonsignificant trend toward decreased mortality [35].
The promising findings in small randomized controlled trials led to the largest multicenter randomized placebo-controlled trial on surfactant use for PARDS to date [36]. For this trial, 153 pediatric patients with acute hypoxic respiratory failure were randomized to receive calfactant versus air placebo. As in previous studies, patients in the experimental surfactant arm demonstrated a significant improvement in oxygenation after treatment. However, this study revealed no difference in the primary outcome, ventilator-free days. Mortality was lower in surfactant-treated children (19% vs. 36%, p = 0.03), but the difference was not significant (OR for survival, 2.11; 95% CI, 0.93–4.79) after adjusting for immunocompromised state, which was unequally distributed between groups.
This led to the hypothesis that surfactant use in the immunocompromised patient would have an improvement in mortality. Unfortunately, a study to address this question suffered from poor enrollment [37]. In this study of children and young adults (aged 18 months to 25 years) with leukemia or lymphoma having undergone hematopoietic stem cell transplantation, and now suffering from severe acute hypoxic respiratory failure, 43 subjects were enrolled from 17 PICUs. There were no differences in PICU survival, oxygenation, ventilator-free days, or functional outcomes between treatment arms.
Additionally, another study was conducted including both adult and pediatric patients with acute respiratory distress syndrome [38]. In this trial, a different, double-concentrated formulation of calf’s lung surfactant was used. In this trial, only patients with direct lung injury were included (i.e., lung injury originating on the alveolar side of the alveolar-capillary membrane). Both adult and pediatric arms of this study were terminated after interim analysis demonstrated no effect on mortality. Also, this study failed to show an improvement in oxygenation, as had been shown in prior trials.
This result calls to question the potential importance of the surfactant formulation on its efficacy. While the double concentration of surfactant was chosen to decrease the volume necessary to instill into the lungs of larger pediatric and adult patients, this may have had an unintended adverse effect on the effective distribution of surfactant throughout the extremely large surface area of the mature lung.
Although surfactant is perhaps the most extensively studied specific ancillary pulmonary treatment for PARDS, its role is still unclear. Early successes have been frustrated by more recent failures of clinical trials to show a benefit of surfactant use. It is quite possible that specific patient populations would benefit from the right formulation of exogenous surfactant, delivered in the appropriate way. Further study is warranted to evaluate specific populations, dosing forms, and delivery regimens. For now, exogenous surfactant use is not recommended for routine use in PARDS [19].
Pulmonary Hygiene
Endotracheal Suctioning
Maintenance of a patent endotracheal tube is of obvious importance in the care of patients with PARDS requiring invasive mechanical ventilation. However, methods to clear pulmonary secretions from the airway are variable and likely based more on institutional culture and routine practice than on medical evidence.
While clearance of the airway is crucial, care must be taken to avoid the unintended consequence of alveolar derecruitment caused from application of highly negative pressures within the airway or from disruption of continuous positive pressure ventilation. Because of the lung volume loss associated with ventilator circuit disruption, closed suctioning systems should be used [39]. Also, deep suctioning with uncontrolled pressures should be avoided, as this is associated with significant risk for lung collapse [40].
The utility and risks of lavage to aid in secretion clearance are also undetermined. Saline lavage may at times be necessary for removal of thick secretions, but its routine use is not recommended [41]. Additionally, routine periodic suctioning of the endotracheal tube may cause more harm than benefit, and suctioning should be performed only when secretions are present at the most shallow depth necessary [19]. Attention to avoid excessive trauma and bleeding is also recommended.
Chest Physiotherapy
There are a myriad of mechanisms available that have the goal of aiding in pulmonary secretion clearance to maintain patent lower airways and improve alveolar recruitment. These include hand percussion and various devises that provide vibration, intrapulmonary percussive ventilation, chest wall oscillation, and rapidly alternating pressures, among other mechanisms. There are no trials evaluating these various mechanisms in PARDS. Decisions to apply chest physiotherapy in the presence of atelectasis must be made after weighing the potential benefit against the risks and significant costs. Routine prophylactic use against the development of atelectasis in the PARDS patient is not warranted.
Nebulized Therapies
The nebulization of medications to aid in pulmonary secretion clearance is common practice in the PICU, but is not supported by medical evidence. The use of N-acetylcysteine, dornase alfa, and hypertonic 3% saline lacks sufficient evidence to promote routine use in PARDS. The prophylactic use of 3% saline in mechanically ventilated children was associated with no difference in duration of ventilation or mechanical ventilation parameters taken before and after treatment, compared to placebo [42]. As with chest physiotherapy techniques, nebulization of medications to aid in secretion clearance and to prevent lower airway obstruction and atelectasis is not routinely warranted in PARDS. Individual use must weigh the potential benefit with the risks and costs of these therapies.
β-agonist therapy has also not been studied specifically in PARDS, but studies are available in adult patients with acute lung injury. A meta-analysis of these studies showed an association with β-agonist treatment and increased morbidities such as reduced mechanical ventilation-free days and organ failure-free days [43]. Therefore, β-agonist therapy is not recommended for use in PARDS outside of a specific alternative indication.
Bronchoscopy
The diagnostic and therapeutic application of bronchoscopy in PARDS is also not well defined in the medical literature. It may be considered in a case-by-case basis to obtain bronchoscopic broncho-alveolar lavage samples for their diagnostic utility. The therapeutic application for clearance of secretions from large airways (with or without lavage) may also be considered. In cases of persistent lobar atelectasis, this method to clear potential mucus plugs of the large airways may be warranted after consideration of risks and benefits. Further evidence is needed to determine the optimal utility of bronchoscopy for both diagnostic and therapeutic reasons.
Bronchoscopy has also been used to instill various medications, such as dornase alfa, directly to the airways. Again, evidence is limited to few case reports, and routine use of this treatment strategy is not recommended. Finally, bronchoscopy has also been reported as a modality to deliver surfactant [44, 45]. Further study is warranted to investigate this strategy, which may improve the distribution of surfactant in larger patients.
Stem Cell Therapy
Endothelial cell damage of the pulmonary capillaries is one of the inflammatory hallmarks of ARDS. The recruitment of circulating endothelial progenitor cells, which leave the bone marrow into the blood stream and can reconstitute the endothelium, may be one important step in recovery from ARDS. Because of this, cell-based therapies have become a novel area of research in developing new treatments for ARDS [46]. Currently, animal studies are showing the utility of both intravenous and endotracheal administration of mesenchymal stem cells or endothelial progenitor cells in animal models of ARDS [47–49]. The future development of these therapies may be an exciting new frontier in the treatment of PARDS.

Full access? Get Clinical Tree
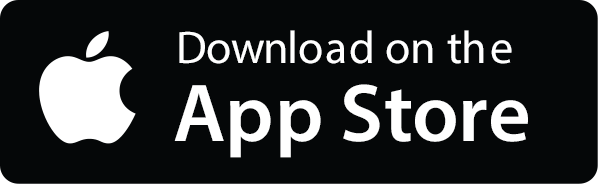
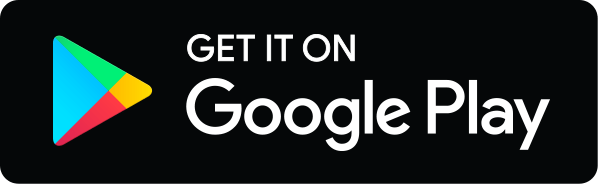