This chapter will discuss pulmonary stenosis, where it exists as an isolated finding. Where pulmonary stenosis exists as part of a more complex anomaly, such as tetralogy of Fallot, the reader is directed to the complete descriptions found in the relevant chapters.
GENETICS, EMBRYOGENESIS AND INCIDENCE
Genetics
The first descriptions of pulmonary stenosis were recorded by Giovanni Baptista Morgagni, of Padova in Italy, in 1761. 1 This lesion is now known to be common, and comprises about one-tenth of all congenital cardiac malformations.
The incidence of pulmonary stenosis in the United Kingdom or North America is about 0.4%. The rate of familial recurrence, however, is considerably higher, at up to 4%, suggesting that a proportion of cases at least are linked to a genetic basis. Further investigations within families have supported this hypothesis, suggesting that pulmonary stenosis can be caused by a single gene defect with or without additional abnormalities within the phenotype. Clearly there are a number of genetic abnormalities involved. At the time of writing, a search on the Online database for Mendelian Inheritance in Man 2 for variants of pulmonary or pulmonic, stenosis, or valve stenosis, revealed 92 reports for conditions including pulmonary stenosis where Mendelian inheritance is suspected. Of these, the locus for a genetic abnormality, be it a point gene mutation, deletion or aneuploidy, were confirmed in two-thirds of the results, whilst the remaining third of the reports were of cases with Mendelian or suspected Mendelian inheritance. Perhaps the most frequently presenting patients with a genetic basis for their pulmonary stenosis are patients with Noonan’s or Williams’ syndromes.
Non-genetic factors have also been implicated. For instance, the congenital rubella syndrome, now very rarely seen because of adequate immunisation and prenatal maternal screening, includes severe peripheral pulmonary arterial stenosis.
Embryology
The precise embryology of the development of the pulmonary valve in the human, indeed both ventricular outflow tracts, is not fully understood. Because of the obvious constraints of studying human embryological development, other species of embryo have formed the basis of the understanding of embryology. The most common models have been proposed based on sequential sections and stains of the developing chick embryo or chick-quail chimera. Difficulties also exist because of differing nomenclature used between the groups studying the lesions.
As we have explained in Chapter 3 , the heart develops as a tubular structure with a solitary lumen when the embryo is 20 days old, differentiating into a sequential pulsatile pump by 28 days. By 30 days, partial separation has occurred, creating a chambered structure with interatrial and interventricular communications. Remodelling of the outflow tract produces two vessels, each with its own arterial valve. The tissues of the outflow tract are derived from different sources. The cardiac mesenchyme contributes to the myocardium and some of the endothelium, with other endothelial contributions from migrating cells of the non-cardiogenic regions of the lateral plate and head mesenchyme. Initial septation is produced by ingrowth of mesenchyme, derived from the neural crest, into the cushions developing throughout the outflow tract. These cushions then fuse with each other, and also with the back wall of the aortic sac between the origins of the arteries supplying the fourth and sixth aortic arches. This process achieves separation of the intrapericardial components of the aorta and the pulmonary trunk. The proximal part of the outflow tract remains encased in a sleeve of outflow myocardium. It is division of the distal part of this persisting muscular outflow tract, again by the cushions packed with cells from the neural crest, which produces the primordiums of the developing aortic and pulmonary valves. It is the outflow cushions themselves, by a process of cavitation, that are remodelled at their distal ends to produce the leaflets and sinuses of the valves. The role of cells derived from the neural crest is demonstrated by ablation of the neural crest itself, which results in failure of septation, and a persistent common arterial trunk. Tissue derived from sources other than the neural crest also plays a role in differentiation and separation of the ventricular outflow tracts, and the origin of this tissue is still not known. 3 The reader is directed to a complete review of the literature and discussion of the role of tissues derived from the cardiac neural crest in the development of the cardiac outflow tracts provided by Waldo and colleagues. 4
The proximal portions of the left and right pulmonary artery branches derive from the caudal portion of the aortic sac, which also feeds the arteries of the sixth pharyngeal pouches. The distal portion of the right sixth branchial arch regresses completely, while the distal portion of the left branchial arch persists as the arterial duct, and latterly the ligament of the arterial duct. The peripheral pulmonary vasculature, distal to the origin of the branches of the pulmonary trunk, derives from the postbranchial pulmonary vascular plexus, which develops in close relationship with and alongside the developing lung buds.
Incidence
Pulmonary stenosis is the second most common congenital cardiac malformation. In a large prospective study of all live born infants, including also data from autopsies of stillborn infants, 5 the total incidence of congenitally malformed hearts was 6.6 per 1000 live births. Of these, pulmonary stenosis accounted for 5.8%. Half of all congenitally malformed hearts include pulmonary stenosis as a component of the defect.
MORPHOLOGY
As far as stenosis within the pulmonary outflow tract is concerned, obstruction at valvar level is by far the most common lesion. Understanding completely the mechanisms responsible for stenosis mandates a clear appreciation of normal valvar anatomy. 6 At present, such understanding is constrained by varying use of the term annulus to describe the structure of the normal valve. Paradoxically, it is only when the valve is stenotic that the attachments of the leaflets approximate to an annular arrangement.
The Normal Pulmonary Valve
The essence of normal valvar anatomy at the ventriculo-arterial junction is the suspension of the leaflets in semilunar fashion within the sinuses of the pulmonary trunk. The key feature is that the hinges of the leaflets cross the anatomic ventriculo-arterial junction. The arrangement is best seen when the normal outflow tract is spread open, having already removed the leaflets ( Fig. 43-1 ). The dissection shows that each leaflet is attached at its two extremities at the sinutubular junction, whilst the basal attachment is supported by the musculature of the subpulmonary infundibulum. True anatomical rings can then be identified at the level of the sinutubular junction, and also at the anatomic ventriculo-arterial junction, this latter structure being the locus over which the fibroelastic walls of the pulmonary trunk are supported by the muscular subpulmonary infundibulum. A third ring can then be constructed by joining together the basal attachments of the leaflets, but this latter ring is a geometric construction, rather than an anatomic reality. The semilunar line of attachment of each of the leaflets, which marks the haemodynamic ventriculo-arterial junction, crosses the anatomical junction between the muscular infundibulum and the pulmonary trunk in two places. Because of this, crescents of muscular infundibulum are incorporated at the base of each pulmonary sinus, while three tapering triangles of fibrous pulmonary truncal wall extend beyond the anatomical ventriculo-arterial junction as parts of the ventricular outflow tract, reaching to the level of the sinutubular junction ( Fig. 43-2 ).


In this normal arrangement, the free edge of each leaflet is appreciably longer than the cord of the sinus that supports it, thus permitting the three leaflets to fit snugly together when closed so as to produce a competent valvar orifice. It is the semilunar nature of suspension of the leaflets, therefore, that permits competent closure and unobstructed opening of the valve, with the zones of apposition between the adjacent leaflets extending in triradiate fashion from the centroid of the valvar orifice to the sinutubular junction at its periphery ( Fig. 43-3 ).

Pulmonary Stenosis
It is fusion of the adjacent leaflets along their commissures, or zones of apposition, which is the essence of valvar stenosis. The fusion along the zones of apposition is typically uniform. It begins peripherally, so that the valvar orifice is narrowed to a central opening. The more the fusion extends towards the centre of the valve, the narrower will be the central opening, and the more severe will be the valvar stenosis. When stenosis of a trifoliate valve is mild to moderate, the opening has a triangular configuration ( Fig. 43-4 ).

In contrast, in the most severe forms which produce typical neonatal critical stenosis, the extent of fusion is sufficient to leave only a central pin-prick opening. In this so-called domed stenosis, the central part of the dome tends to be smooth, with evidence of the fused zones of apposition seen to varying degrees as peripheral raphes with tethering at the sinutubular junction ( Fig. 43-5 ).

When the critically stenotic valve is opened, then because of the obliteration of the zones of apposition between the leaflets, there is also a reduction in the extent of their semilunar hingeing, so that the line of attachment within the pulmonary root achieves a more circular configuration ( Fig. 43-6 ). This is the annular paradox, where ring-like attachments become evident only when the valve is malformed. In this respect, it should also be noted that, although it is frequent to describe the orifice of the normal pulmonary valve in terms of the annulus, the diameter usually measured in this fashion by the echocardiographer is at the level of the virtual basal ring ( Fig. 43-7 ).


As we have already demonstrated, when such a normal valve is opened, it is the semilunar attachments of the leaflets which dominate the picture. An attachment approximating to a ring is never seen unless the valve is stenotic (see Fig. 43-6 ).
In some instances, it is possible to identify four raphes, suggesting that the valve itself initially had four leaflets. Indeed, quadrifoliate non-stenosed pulmonary valves are by no means unusual, and were discovered as incidental benign lesions once in each 250 postmortems by Becker. 7 The incidence of a bifoliate, or biscuspid, and stenosed pulmonary valve is reported to be between 7% and 17%, diagnosed with echocardiography or by the surgeon. Archives of autopsied hearts, including our own, have not replicated such a high incidence. 7–10
The typical lesion producing pulmonary valvar stenosis, therefore, is uniform fusion of the peripheral zones of apposition of a trifoliate valve, leaving a central aperture. Usually this arrangement is associated with some degree of thickening at the union of the zones of apposition with the sinutubular junction, this arrangement being described surgically as tethering (see Fig. 43-4 ). Accentuation of such tethering can produce marked narrowing at the sinutubular junction, giving an hourglass appearance that is often described as supravalvar. In reality, the sinutubular junction is an integral part of the valvar complex. In some instances, valvar stenosis is the consequence of dysplasia of the leaflets, making them thick and mucoid. Such thickening is sufficient to produce obstruction of the valvar orifice, even should the leaflets themselves not be fused along their zones of apposition. Clinical experience indicates, however, that such thickened leaflets usually co-exist with a narrowed sinutubular junction. Most of the patients with Ullrich-Noonan’s syndrome have this type of stenosis. 11
Although pulmonary valvar stenosis may be considered a simple lesion, it is rare for the lesion to be totally isolated. Almost always there is hypertrophy of the right ventricular wall. In the neonate with critical valvar stenosis, such hypertrophy may be severe, with accompanying fibrosis of the endocardium and thickening of the tension apparatus of the tricuspid valve. The right ventricle then resembles the situation seen at the better end of the spectrum of pulmonary atresia with intact septum (see Chapter 30 ). The findings of areas of subendocardial right ventricular infarction in the absence of coronary arterial disease, 12 coupled with discovery of extensive areas of disarray in the myocardial and arterial walls in hearts from patients aged 6 days to 9 years, 13 point to the disease being more generalised than would be expected from a simple valvar lesion producing direct haemodynamic effects. When the right ventricular wall is hypertrophied, the size of the ventricular cavity is usually relatively reduced, and this can be the consequence of overgrowth and obliteration of the apical trabecular component, again as seen in pulmonary atresia with intact septum (see Chapter 30 ).
An interatrial communication, be it a probe patent oval foramen or a true septal deficiency, is more frequently seen in patients with pulmonary stenosis, occurring in up to three-quarters of cases. Indeed the presence of a left-to-right shunt at atrial level may serve to accentuate the physical signs of pulmonary stenosis, which could otherwise be mild. This combination of pulmonary stenosis, atrial septal defect, and right ventricular hypertrophy was originally described by French investigators as the trilogy of Fallots, 14 recalling the initial description of Fallot, 15 when he identified such a combination as an alternative cause of la maladie bleue, and distinguished it from the tetralogy. Despite this excellent historical pedigree, there now seems little justification for retaining the notion of a trilogy.
In days gone by, 16 it was also the case that cardiac cirrhosis was noted in adults dying with pulmonary stenosis, but such hepatic changes are unlikely to be encountered now. Early fibrotic changes in the livers from two children dying at the age of 6 and 7 years, nonetheless, have been reported. 17
Infundibular Stenosis
Pure narrowing of the muscular subpulmonary infundibulum is rare in the setting of an intact ventricular septum, or with an apical ventricular septal defect. Of the cases seen at the Hospital for Sick Children, Toronto, over a period of almost 25 years, only 2.7% had this type of stenosis. 17 It is probable that many of the cases described as having isolated infundibular stenosis have previously also had a ventricular septal defect that closed spontaneously.
Combined Valvar and Infundibular Stenosis
Hypertrophy of the subpulmonary infundibulum occurs along with hypertrophy of the rest of the right ventricle in response to valvar stenosis. This reduces the infundibular diameter, and endocardial fibroelastosis may be seen along with the hypertrophy. Fixed stenosis of the infundibulum co-existing with valvar stenosis is exceedingly rare. 17 The reactive stenosis, however, is an important component to be noted when judging the results of balloon valvoplasty, since time is needed for its regression.
Other Types of Stenosis Occurring Within the Right Ventricle
These are very rare when the ventricular septum is intact, but they must be considered as possible causes of pulmonary stenosis. Hypertrophy of the body of the septomarginal trabeculation, which in its severest form produces the typical two-chambered right ventricle, is usually found with a ventricular septal defect. 18 It has been described, nonetheless, with an intact septum. 19 In other patients, the valve of the embryonic venous sinus can persist, and become so expanded and aneurysmal that it can pass through the tricuspid valve and obstruct the pulmonary outflow tract (see Chapter 26 ). This is described as the spinnaker syndrome. 20 A similar case has been described in which a huge aneurysm of the membranous septum produced subpulmonary stenosis. 21 More rarely, tumours can cause subvalvar right ventricular obstruction, 22 as may aneurysm of the right coronary sinus of the aorta. 23 Hypertrophic cardiomyopathy can also afflict the right ventricle, particularly in the setting of lentiginosis, although left ventricular obstruction usually dominates.
Pulmonary Arterial Stenosis
Stenoses of the pulmonary arterial tree are frequent in association with complex malformations such as tetralogy of Fallot or transposition. They can also complicate more simple lesions such as pulmonary valvar stenosis or ventricular septal defect. Stenoses in the pulmonary arteries can also occur in isolation, and in various parts of the pulmonary arterial tree. Thus, the stenosis may be localised and central, within the pulmonary trunk or its right and left branches, localised and peripheral at the sites of branching of major intrapulmonary arteries, or more extensive, then existing as a hypoplastic arterial segment commencing either at the end of the right or left pulmonary artery or a major intrapulmonary branchpoint. When found peripherally, such stenoses may be unilateral. Reported cases were well summarised by Rowe, 24 who suggested that an anomaly of medial elastic tissue, together with intimal proliferations, could be the cause. It is likely that the process of disease will not be limited to the pulmonary arteries, but will involve also the systemic vessels. 25 In this respect, it is noteworthy that the pulmonary arteries are also involved in the so-called Macaroni syndrome, a condition that primarily narrows the ascending aorta.
Pathogenesis
It is now well recognised that dome-shaped stenosis of the pulmonary valve develops as an acquired condition during intra-uterine life, 26 often progressing to produce pulmonary valvar atresia with an intact ventricular septum (see the extensive discussion of this topic in Chapter 30 ). The degree of severity seen at birth depends upon the extent of the process during gestation. Peripheral pulmonary arterial stenoses may be caused by congenital rubella, 27 and they are seen in recognisable syndromes of malformation such as Alagille’s syndrome 28 and Williams’ syndrome. 29–31 Alagille’s syndrome, also known as arteriohepatic dysplasia, has been linked to several genetic malformations, such as Jag1 32,33 and NOTCH2, 34 and often includes severe intrapulmonary arterial stenoses associated with other abnormalities, including problems of the liver, eyes, and spine. Indeed, the hepatic malformation may appear to be more severe than the pulmonary arterial pathology, although experience has shown that aggressive management, such as hepatic transplantation, can be complicated by the pre-existing pulmonary arterial pathology and intracardiac abnormalities. 35 Williams’ syndrome, a deficiency of elastin with a gene located on 7q22, 30 is associated with peripheral pulmonary stenosis, supravalvar aortic stenosis, and descending aortic hypoplasia. 29,36
Pulmonary Arterial Disconnection
An important group of patients have an apparent disconnection of one pulmonary artery from the pulmonary trunk. 37,38 The disconnected pulmonary artery may have originally been connected by the arterial duct to the aorta or the subclavian artery, and is therefore a true embryological failure, with luminal and adventitial discontinuity from the pulmonary trunk, though sometimes a vestigial duct is still demonstrable ( Fig. 43-8 ). Alternatively, the disconnection may have been acquired as a process of coarctation, where ductal tissue has constricted the lumen of the pulmonary artery to the point of obliteration. In this case, of course, there is still adventitial continuity between the pulmonary trunk and the disconnected pulmonary artery. These considerations are important when surgical reconstruction of the connection is considered, as is the size of the disconnected pulmonary artery at the hilum. Demonstrating the size and integrity of the native vessel when no arterial or ductal connection exists can be difficult. Echocardiography, computerised tomography and magnetic resonance scanning may not be helpful. Cardiac catheterisation and wedge intubation of the pulmonary vein on the side of the disconnected artery for a careful retrograde injection of contrast, may be the only helpful investigation 39 ( Fig. 43-9 ). Where no interatrial communication exists, transarterial retrograde intubation of the pulmonary vein, 40 or needle puncture of the atrial septum permitting a trans-septal approach, will be required.


CLINICAL DIAGNOSIS
Presentation
The presentation of pulmonary stenosis depends on age and severity. In the neonate, critical pulmonary stenosis presents with life-threatening cyanosis, with a right-to-left shunt at atrial level producing cyanosis. The differential diagnosis would include other forms of neonatal cyanosis, including transposition, and the various forms of pulmonary atresia. Such patients are likely to depend on their arterial duct to provide the flow of blood to the lungs. Hence, palliation in the short term, by maintaining ductal patency with intravenous infusions of prostaglandin, is life-saving, until a more definitive diagnosis can be made and an appropriate intervention planned.
Outside of the neonatal period, mild or moderate pulmonary stenosis is not likely to cause major symptoms, unless there are associated lesions or other factors. For example, patients with the typical phenotype of Noonan’s syndrome may have difficulty with feeding and gaining weight, separate in most cases to the severity of the pulmonary stenosis. Discovery of disease of mild or moderate severity is most likely when physical signs are detected during consultations for separate matters, when most commonly a cardiac murmur is heard. In patients with more severe pulmonary stenosis, the onset of symptoms can still be delayed into late childhood or early adult life. In a small proportion of patients with pulmonary stenosis, cyanosis can be present, especially on exercise. This is a late physical sign because the cyanotic shunt is at atrial level, depending firstly on an interatrial communication, and secondly on the diastolic properties of the right ventricle. With severe right ventricular hypertrophy and poor right ventricular diastolic compliance, the passage of unoxygenated blood across an interatrial communication into the left atrium is facilitated. Reported symptoms include exercise intolerance, or dyspnoea. Chest pain of an ischaemic nature, possibly due to subendocardial ischaemia of the right ventricle, syncope, and severe dyspnoea on minimal exertion, are late findings and should prompt rapid intervention.
Physical Examination
The physical examination findings are summarised in Table 43-1 . There may be a thrill at the second left intercostal space, which is not felt over the carotid arteries, differentiating the cause of the thrill from aortic stenosis. Palpating for a thrill in the suprasternal notch should be avoided, since thrills here can be due to either aortic or pulmonary stenosis.
Severity of Pulmonary Stenosis | |||
---|---|---|---|
Physical Sign | Mild | Moderate | Severe |
Cyanosis | Absent | Absent | Possible, if interatrial septal defect |
Jugular venous pulse | Normal | Normal |
|
Praecordial thrill | Absent | Probable | Pronounced |
Right ventricular heave | Absent | Probable | Pronounced |
Systolic ejection click | Present | Expiratory | Probably absent |
Ejection systolic murmur | Mid systolic, ejection | Long ejection systolic | Long, ejection systolic, past aortic closure sound |
Second heart sound | Normal | Wide, variable | Absent pulmonary closure sound |
Widened splitting of the second heart sound, and an ejection systolic murmur, is maximally heard here, with radiation into the back. It is the delayed closure of the valvar leaflets that causes the widened splitting. This is not due to electrical prolongation of systole, but rather to continued flow through the pulmonary valve even after right ventricular pressures have fallen to levels that would usually have resulted in valvar closure. This is likely to be a ventriculo-arterial interaction, favoured by a normal or increased impedance of the pulmonary vascular bed. It can be termed pulmonary hangout.
In most cases of valvar pulmonary stenosis, a systolic ejection click precedes the onset of the systolic murmur. The click is usually heard loudest separate from the area of maximal intensity of the cardiac murmur, nearer the apex, or at the left lower sternal edge. The systolic ejection click originates from the rapid deceleration of the pulmonary valvar mechanism, as the fusion along their zones of apposition prevents the valvar leaflets from reaching their intended positions against the walls of the valvar sinuses. Presence of the click also depends on the timing of opening of the pulmonary valve. In mild disease, without right ventricular hypertrophy, the pulmonary valve opens in ventricular systole with the emission of an ejection click. In severe disease, with right ventricular hypertrophy and impaired diastolic compliance, the valve opens in atrial systole, without the rapid deceleration cause by ventricular systole. Thus, no click is heard. In moderate pulmonary stenosis, inspiration augments systemic venous flow, and atrial filling. The augmented atrial pressure allows atrial systole to open the pulmonary valve. Hence, the systolic ejection click is heard only in expiration. In some patients with pulmonary stenosis and tricuspid regurgitation, the right ventricular pressure is high enough to cause a pansystolic murmur at the left lower sternal edge.
The physical findings in patients with supravalvar stenosis are similar, except for the absence of the ejection click. Stenosis further out into the pulmonary arterial tree can be heard as long systolic or continuous murmurs in the axillas, or over the lung fields at the back. Careful general phenotypic assessment may show the signs or syndromic diagnosis, such as Noonan’s syndrome, Alagille’s syndrome, or Williams’ syndrome.
Basic Investigations
Basic investigations are directed towards assessing the effects and the severity of the pulmonary stenosis (Table 43-2) .
Mild | Moderate | Severe | |
---|---|---|---|
Electrical axis | Normal | 90–130 degrees | 110–150 degrees |
R:S ratio | Normal | Up to 4:1 in V 1 | Inverted in left leads also |
V 1 R amplitude | Normal | 10–20 mV | >28 mV |
Right atrial enlargement | Normal | Possible | Present |
The Electrocardiogram
Electrocardiography may be normal, or reveal the degree of right ventricular hypertrophy, or indeed right atrial enlargement, which will be seen only in the more severe cases. There are no features of the electrocardiogram that are unique to the site of pulmonary stenosis, although the severity of stenosis is reflected in the degree of right ventricular hypertrophy and strain. A full right bundle branch block pattern is rare, but an RSR pattern can be seen, and reflects a lesser degree of right ventricular hypertrophy than the equivalent exclusive R wave complex in V 1 . 41,42 Rudolf proposed that, in children, the voltage of a pure R-wave recorded at V 1 correlates with approximately one fifth of the right ventricular pressure. 43 It turns out that the QRS axis may be a more reliable indicator of right ventricular pressure. 44 Indeed the prediction of right ventricular pressure gradient is optimised by use of a number of variables in addition to the measurements of voltage on the electrocardiogram, as proposed by Ellison and colleagues, 45 as follows:
Δ P = RV 1 + 1.5 • ( SV 6 ) + 15 • ( murmur [ grade 0 − 6 ] ) + Clinical Score − 30
The clinical score included presence of cyanosis, worth + 25; the pulmonary component of the second sound, if diminished worth 15, or 25 if inaudible; a QRS axis of greater than 90 degrees, worth 10; and negative T waves in aVF and V1, worth 35, and with RV1 above 10 mm worth 15.
This complex formula has been superseded by the quicker, more accurate, and more reliable use of Doppler echocardiography.
The Chest X-Ray
The investigation shows the features of right ventricular hypertrophy, and if present, post-stenotic dilation of the pulmonary trunk. Right ventricular hypertrophy is sees as an upturned cardiac apex. The dilated pulmonary trunk is seen as a prominence of the left upper heart border, inferior to the aortic knuckle ( Fig. 43-10 ). Right atrial enlargement causes a more pronounced convexity of the right lower heart border.

Echocardiography
The gold standard investigation for the assessment of the morphology of pulmonary stenosis is cross sectional echocardiography, coupled with Doppler analysis of the flow in the right ventricular outflow tract and pulmonary arteries. M-mode echocardiography no longer has a part to play in the diagnostic process, although many echocardiographers will still employ M-mode to analyse timing of pulmonary valvar motion.
Cross sectional echocardiography will define whether the obstruction is at the level of the valvar leaflets ( Fig. 43-11 ), or is subvalvar ( Fig. 43-12 ) or supravalvar ( Fig. 43-13 ). The presence and severity of restricted motion of the leaflets, and dysplasia, can readily be assessed, along with any post-stenotic dilation of the pulmonary trunk ( Fig. 43-14 ). The diameter of the pulmonary valve, at the haemodynamic ventriculo-arterial junction, can be measured accurately ( Fig. 43-15 ), facilitating the planning of intervention.





Distal to the valve, it is possible to identify stenosis within the proximal pulmonary arterial tree, although other forms of imaging are necessary to assess pulmonary arterial pathology distal to the bifurcation. The presence of additional abnormalities, such as atrial septal defects, can be recorded.
Previously, electrocardiography was the mainstay of non-invasive assessment of severity of pulmonary stenosis, although electrocardiographic correlates of right ventricular hypertrophy are relatively weak. Since the advent of Doppler echocardiography, assessment is greatly simplified. Colour flow shows turbulent blood originating at the point of stenosis ( Fig. 43-16 ). Both pulsed and continuous wave interrogations permit measurement of the maximal velocity of the flow across the stenosis ( Fig. 43-17 ). The pressure gradient can be estimated more accurately using the modified and simplified Bernoulli equation, where the valvar pressure gradient, expressed in millimetres of mercury, or ∆P, is calculated from the maximal Doppler velocity at metres per second, or V, across the pulmonary valve:
Δ P = 4 • V 2
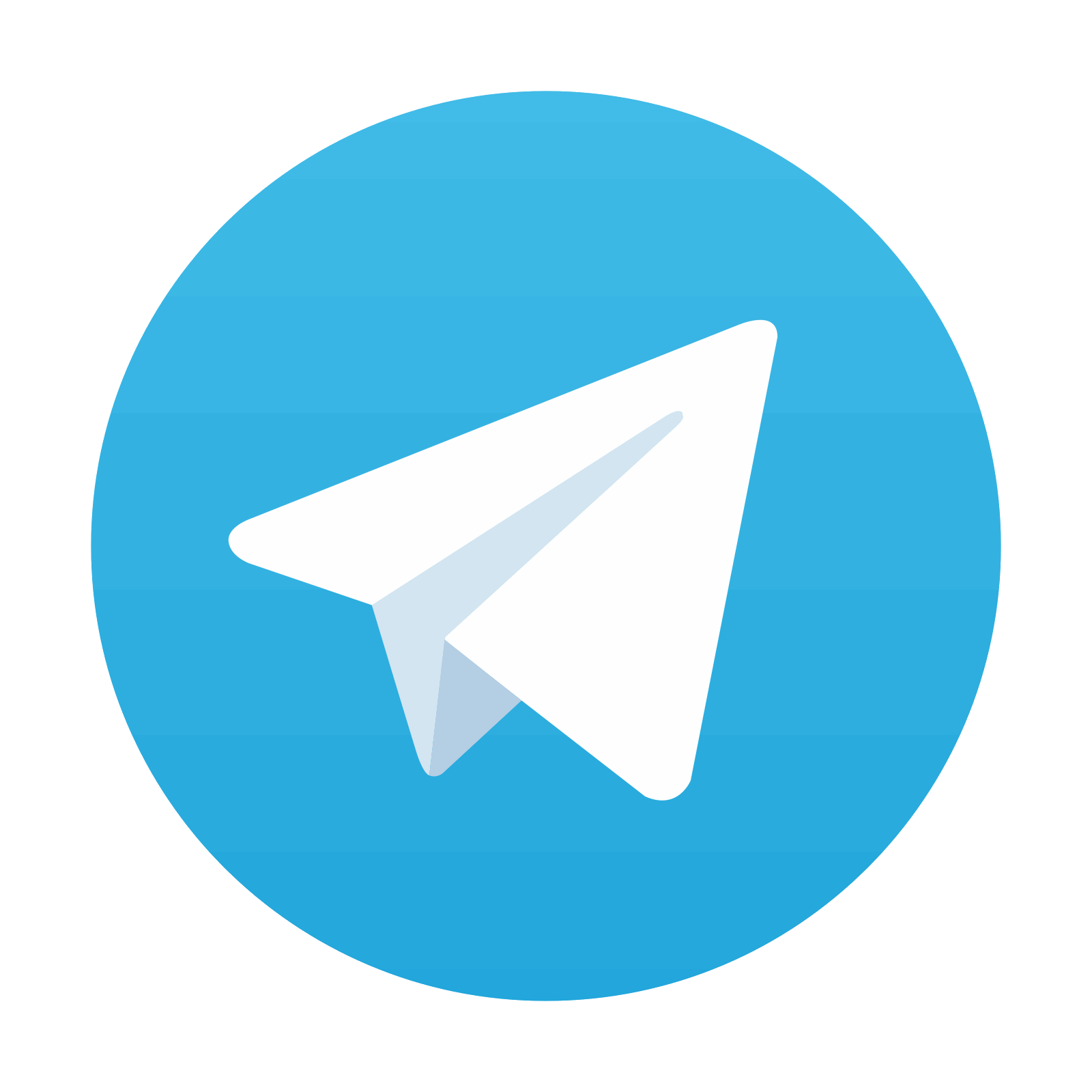
Stay updated, free articles. Join our Telegram channel

Full access? Get Clinical Tree
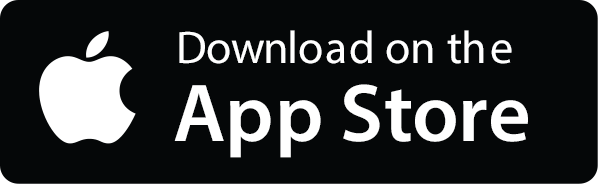
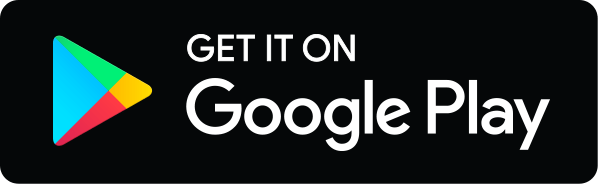