Epidemiology
Pulmonary complications occur in 5 to 10 percent of all surgical patients and have been shown to prolong hospital stay by 1 to 2 weeks. Approximately 25 percent of deaths within the first week after surgery are due to pulmonary complications.
Pathophysiology
Pneumonia occurs in 10 to 40 percent of patients after major surgery and is associated with a mortality rate of 30 to 45 percent. General anesthesia can decrease lung volumes by 30 to 40 percent, and this decrease may persist for 1 to 2 weeks postoperatively.
Clinical features
Cardiothoracic surgery is associated with the highest frequency of pulmonary complications. Risk factors include the type of surgery, general anesthesia, length of surgery and general anesthesia, smoking, chronic obstructive pulmonary disease, age, obesity, functional status, and a history of dyspnea.
Diagnostics
Preoperative evaluation should include a directed history and physical examination to identify those patients at particular risk for pulmonary complications. Pulmonary function tests with spirometry and arterial blood gases (ABGs) should be obtained for any patient undergoing lung resection. For further risk stratification, cardiopulmonary exercise testing (CPET) is indicated in high-risk patients who do not have adequate predicted postoperative lung function. Alternatives to CPET include the shuttle-walk or stair-climb test.
Bronchoscopy is an essential tool in the preoperative work for diagnosis, staging, and surgical planning.
Treatment
Inhaled bronchodilators may successfully reverse some cases of obstructive pulmonary disease.
Outcomes
Preoperative pulmonary function evaluation can stratify patients for risk, thus identifying those who can safely undergo major cardiothoracic surgery and minimizing the risk of significant morbidity and mortality.
Appropriate nodal staging is essential in the workup of lung cancer patients and essential for surgical planning.
Pulmonary complications in the perioperative period remain a significant cause of morbidity and mortality and occur in 5 to 10 percent of all surgical patients. Not only have these complications been shown to prolong hospital stay by an average of 1 to 2 weeks but 25 percent of deaths within the first week after surgery are reportedly due to pulmonary complications.1–4 One way to minimize these complications is with the appropriate use of preoperative testing. Both noninvasive preoperative testing such as pulmonary function testing and minimally invasive testing such as bronchoscopy can add valuable information to the workup of the preoperative cardiothoracic patient. In this chapter, we will review the burden of postoperative complications, basic pulmonary physiology, the preoperative pulmonary workup for the cardiothoracic surgical patient, and the role of bronchoscopy for the diagnosis and staging of lung cancer.
In the postoperative period, there are a wide range of pulmonary complications (Table 3-1) that can occur in patients with underlying lung disease. Postoperative pneumonia can occur in 10 to 40 percent of patients and is associated with a mortality rate of 30 to 45 percent after major surgical procedures. The benefits of a thorough preoperative evaluation include the identification of patients with underlying lung disease, modification of risk factors, optimization of medical management, avoidance of elective surgery in high-risk patients, and prevention of inappropriate surgical resection by appropriately staging for lung cancer.
There are multiple risk factors for postoperative pulmonary complications including patient related factors as well as the specific type of surgery and anesthesia. The location and type of surgery being performed are the major determinants of pulmonary complication risk. Cardiothoracic surgery has been repeatedly shown to have the highest frequency of pulmonary complications.5 Anesthesia is also important in determining postoperative risks as it has been shown that general anesthesia can decrease lung volumes by 10 to 30 percent for which these changes may persist for 1 to 2 weeks postoperatively. The combination of thoracic surgery and general anesthesia often leads to decreased lung volumes, worsening gas exchange, and impairment in airway clearance, all of which may result in severe complications, especially in patients with underlying lung disease.
Patient-specific risk factors that have been identified are listed in Table 3-2. Appropriate identification and modification of reversible risk factors is an important component of the preoperative evaluation and one that can significantly reduce operative risk. One of the most important modifiable risk factors is smoking cessation several weeks prior to elective surgery;6 cessation 2 weeks prior may in fact increase postoperative complications, likely as a result of the impaired mobilization of secretions.
Identifying patients who are at increased risk of pulmonary complications begins with a detailed history and physical examination. Any unexplained dyspnea at rest or on minimal exertion should warrant a more thorough evaluation prior to any elective surgical procedure. Smoking cessation should be recommended to all patients, ideally having them quit at least 2 weeks before the surgery. If a patient has any history of prior lung disease, significant tobacco use, or dyspnea with mild exertion, they warrant pulmonary function testing before any high-risk elective surgical procedure.
The American College of Physicians recommends preoperative pulmonary function testing for the following patients7:
All patients with a history of tobacco use or dyspnea who are undergoing cardiothoracic or upper abdominal surgery.
Any patient with unexplained dyspnea or pulmonary symptoms who is undergoing head, neck, orthopedic, or lower abdominal surgery.
All patients undergoing lung resection.
Preoperative pulmonary function testing most often consists of spirometry, diffusion capacity, and sometimes arterial blood gas (ABG) analysis. Patients undergoing lung resection surgery are clearly at a particularly high risk of pulmonary complications and warrant a more thorough evaluation.
There are several risk factors that have been identified to increase complications in patients undergoing lung resection. Lung resection surgery is associated with significant morbidity in up to 50 percent of patients and death in up to 8 percent.8 The type of resection is a major determinant of operative risk, with pneumonectomy having a greater operative morbidity and mortality than lobectomy.9
Both pulmonary function testing with spirometry and an ABG measurement should be obtained on all patients considered for lung resection surgery.10 Baseline hypercapnia and hypoxia at rest or during exertion predicted an increased surgical morbidity.11
Spirometry is the most accurate and predictive pulmonary function test available. It can identify low-risk patients who can proceed directly to surgery as well as higher-risk patients who warrant further investigation. It is recommended that patients have a postoperative forced expiratory volume in 1 s (FEV1) of greater than 0.8 L or 30 percent of predicted and also a postoperative diffusing capacity of carbon monoxide (DLCO) of greater than 40 percent predicted. If the preoperative FEV1 is greater than 2.0 L for a pneumonectomy, or greater than 1.5 L for a lobectomy, no further pulmonary testing is required, and the patient can proceed directly to lung resection. All patients who have an FEV1 below these cutoffs require more extensive evaluation prior to lung resection (Fig. 3-1). These additional tests are used to help predict postoperative pulmonary function. The quantitative perfusion scan is used to estimate the percent of functional lung that is being resected.12 The estimate of postoperative pulmonary function is calculated with the following equation:
The same equation can be used to calculate the predicted postoperative DLCO. Any patient with a predicted postoperative FEV1 and DLCO above the minimum values outlined above should have a standard risk of pulmonary complications. A patient who does not have an adequate predicted postoperative lung function based on the above testing should not be denied surgery without further evaluation if he or she otherwise appears to be an appropriate surgical candidate. These higher-risk patients should undergo cardiopulmonary exercise testing (CPET) for further risk stratification. The measurement of the maximal oxygen consumption, V̇O2max, by CPET is the most specific predictor of postoperative pulmonary complications following lung resection surgery.13 If the measured V̇O2max is greater than 20 mL/kg/min, the risk of postoperative pulmonary complications is low, and the patient may be safely offered surgical resection. Patients with a V̇O2max <15 mL/kg/min have a moderately increased risk of surgery, and a V̇O2max less than 10 mL/kg/min is associated with a high surgical risk.
Since lung resection is the only cure for many patients with localized lung cancer, the strategies of pulmonary function testing have been designed to maximize their sensitivity in identifying all patients who can safely tolerate lung resection; these strategies must be tailored to the goals of the individual patient.
ABG analysis is a readily available test that provides useful information to identify the high-risk surgical patient. The ABG measurement should be performed at rest and breathing ambient air, if possible. The partial pressure of oxygen (PO2) reflects the adequacy of gas transfer from the alveoli to the blood and when, measured at sea level, the normal Po2 ranges from 85 to 100 mm Hg. The PO2 as a measurement of oxygenation should be interpreted in terms of the oxygen dissociation curve (Fig. 3-2). Since a majority of oxygen in the blood is bound to hemoglobin, relative changes in PO2 can reflect a wide range of changes in oxygen carrying capacity depending on the slope of the oxygen dissociation curve. Changes in PO2 on the steep part of the oxygen dissociation curve reflect large changes in blood oxygen content. In contrast, changes in PO2 on the flat part of the oxygen dissociation curve, PO2 greater than 70 mm Hg, lead to only minor differences in oxygen content. The alveolar–arterial oxygen gradient [A–a gradient = PiO2 − (PaCO2/R) − PaO2] is a more sensitive measurement of gas exchange problems. Since pulmonary function normally declines with age, the normal values for A–a gradient depend on the age of the patient and can be calculated based on the following equation.
An elevated A–a gradient can be due to ventilation/perfusion mismatching, diffusion defects, or shunt.
The partial pressure of carbon dioxide, PCO2, is a measurement of CO2 production (V̇CO2) and alveolar ventilation (V̇A); that is, PaCO2 = V̇CO2/V̇A. Therefore, an increase in CO2 production or a decrease in alveolar ventilation leads to an increase in PCO2. Normally PCO2 is tightly regulated, with normal values at rest between 38 and 42 mm Hg, and is not affected by age. Hypercapnia can be due to a wide range of causes. Alveolar ventilation (VA) is dependent on both minute ventilation (VE) and dead-space ventilation (VD) in the relationship where VT is the tidal volume:
VA = VE (1 − VD/VT)
Conditions such as emphysema or interstitial lung disease lead to an increase in V̇D and a corresponding decrease in V̇A, which can result in hypercapnia with exertion or at rest. Hypoventilation can be due to a number of causes besides parenchymal lung disease, including musculoskeletal and neurologic causes. ABG analysis provides a simple measure of oxygenation and ventilation and the presence of hypoxia or hypercapnia should identify a higher-risk patient who warrants a more thorough evaluation.
Spirometry was first used by John Hutchinson in 1848 and is the most common test performed in the pulmonary function laboratory. Spirometry measures the changes in volume and flow during tidal breathing, maximal forced expiration, and inhalation. The graphic interpretation of the test, the flow volume loop depicts both inspiration and expiration tracings. At least three tests are performed to ensure reproducible effort. Figure 3-3 shows the flow volume loops of a normal individual compared with the patterns seen in obstructive and restrictive lung disease. Spirometry provides several measurements that are important to understand, including the forced vital capacity (FVC), FEV1, and FEV1/FVC ratio. The FVC is a measure of the volume exhaled during the entire forced expiratory effort. The FVC is decreased in both obstructive and restrictive lung diseases. It can also be decreased as a result of chest wall deformities, pleural disease, or neuromuscular diseases. The FEV1 measures the volume of gas exhaled in the first second of exhalation (Fig. 3-4). In restrictive lung disease, the FEV1 is decreased in similar proportion to the FVC; therefore, the FEV1/FVC ratio is normal or even increased. In contrast, patients with obstructive lung disease have difficulty emptying the air within the lungs because of an increased resistance to expiratory flow and a loss of elastic recoil, and the FEV1 is decreased to a greater degree than the FVC. The expiratory flow limitation seen in obstructive lung disease leads to a characteristic decrease in the FEV1/FCV ratio and a classic “scooped out” expiratory flow-volume loop. Spirometry is the only pulmonary function test needed to make the diagnosis of obstructive lung disease, and an FEV1/FVC ratio of less than the fifth percentile of predicted is the diagnostic criterion of the American Thoracic Society (ATS).14 After a diagnosis of airway obstruction has been made, the severity of obstruction is usually determined by the percent predicted of the FEV1, as shown in Table 3-3.
Spirometry is suggestive of restrictive lung disease when both FVC and FEV1 are decreased but the FEV1/FVC ratio is normal; however, measured lung volumes (a distinct test) must demonstrate a decreased total lung capacity to make the diagnosis. Spirometry can also be ordered with and without the use of inhaled bronchodilators to determine the amount of reversible airway obstruction. Reversible airway obstruction is defined as greater than a 12 to 15 percent improvement in the FEV1 or FVC after treatment with an inhaled bronchodilator. The identification of patients with reversible airway obstruction can be an important component of a preoperative pulmonary evaluation, with a view to decreasing airway obstruction and minimizing surgical morbidity. Although spirometry is the most common of all the pulmonary function tests, more extensive testing of lung volumes or gas transfer is often needed.
Since spirometry measures only the volume of air exhaled (vital capacity, VC), it is unable to measure the residual volume (RV) or the total lung capacity (TLC). RV is the air left in the lung after a complete exhalation. TLC equals the RV plus the VC (Fig. 3-5). Several different techniques can be used to determine the TLC and RV. The three most common techniques to measure lung volume include inert gas dilution, nitrogen washout, and body plethysmography. A detailed discussion of each of these techniques is beyond the scope of this chapter; however, it is important to recognize that neither the inert gas technique nor the nitrogen washout technique measures the volume from poorly ventilated areas of the lung. Therefore, both these techniques can underestimate the TLC in patients with obstructive lung disease and air trapping, that is, patients with emphysema. Plethysmography will give an accurate measurement of lung volumes in these patients. As mentioned above, lung volumes are required to make the diagnosis of restrictive lung disease, which is defined by a TLC of less than 80 percent predicted and can result from a wide variety of causes, including pulmonary fibrosis, chest wall disorders, obesity, or neuromuscular disease. After confirming the presence of restriction, the VC is commonly used to quantify the severity of restriction, as shown in Table 3-4.
Figure 3-5
Graphic representation of spirometric relationships. (From surgical tutor.org.) ERV, expiratory reserve volume; FRC, functional residual capacity; IC, inspiratory capacity; IRV, inspiratory reserve capacity; RV, residual volume; TLC, total lung capacity; TV, tidal volume; VC, vital capacity.

For patients with airway obstruction, the total lung capacity is usually normal or increased. Lung volumes are important for patients with airway obstruction and a decreased FVC to look for the presence of air trapping or coexistent restrictive lung disease. Air trapping is the inability to empty areas of the lung and consequent hyperinflation. Lung volumes in this setting demonstrate an elevated RV, greater than 130 percent predicted, and often an elevated TLC. A decreased TLC in the presence of airway obstruction indicates a combination of obstructive and restrictive disease. The combination of obstruction and restriction can make the determination of pulmonary function difficult to quantify but is important to recognize. Lung volumes are not indicated in all patients who undergo spirometry, but they can be useful in patients with a decreased FVC or in patients who are suspected of having restrictive lung disease of any cause.
The third most common test ordered from the pulmonary function lab is the diffusing capacity. It measures the transfer of gas from the air in the alveolar space to the hemoglobin of the red blood cells. Since it is technically difficult to measure the diffusion of oxygen, the pulmonary function lab measures the DLCO that closely mimics the transfer of oxygen and is used as a surrogate. A normal DLCO is approximately 20 to 30 mL/min/mm Hg and is influenced by age, sex, and height. Since the diffusion of gas is influenced by the oxygen-carrying capacity of blood, it is important to recognize the presence of anemia, which can significantly lower the measured DLCO. Pathologic conditions that can lower the DLCO to less than 80 percent predicted include pulmonary fibrosis, emphysema, pulmonary hypertension, right-to-left intracardiac shunt, and anemia. A few rare conditions can elevate the DLCO (greater than 130 percent predicted), which include asthma, alveolar hemorrhage, mild congestive heart failure (CHF), and left-to-right intracardiac shunt. The combination of spirometry, lung volumes, and DLCO can provide a wealth of information about the pathophysiology of lung diseases. Table 3-5 depicts the expected changes seen in different lung diseases.
Category | FEV1/FVC | FVC | RV | DLCO |
---|---|---|---|---|
Asthma | Normal or ↓ | ↓ | Normal or ↑ | Normal |
Emphysema | ↓ | Normal or ↓ | ↑ | ↓ |
Chronic bronchitis | ↓ | ↓ | Normal | Normal |
Interstitial lung disease | Normal or ↑ | ↓ | Normal | ↓ |
Pulmonary hypertension | Normal | Normal | Normal | ↓ |
Neuromuscular disease | Normal | ↓ | Normal or ↑ | Normal |
In summary, the measurement of pulmonary function is readily available, minimally invasive, and can provide a large amount of information that can influence the perioperative and intraoperative management of patients with lung disease. It is important to remember that borderline pulmonary function tests should not exclude a patient from surgery if he or she is otherwise a good surgical candidate. More extensive exercise testing may be useful in patients with borderline pulmonary reserve who are being considered for lung resection.
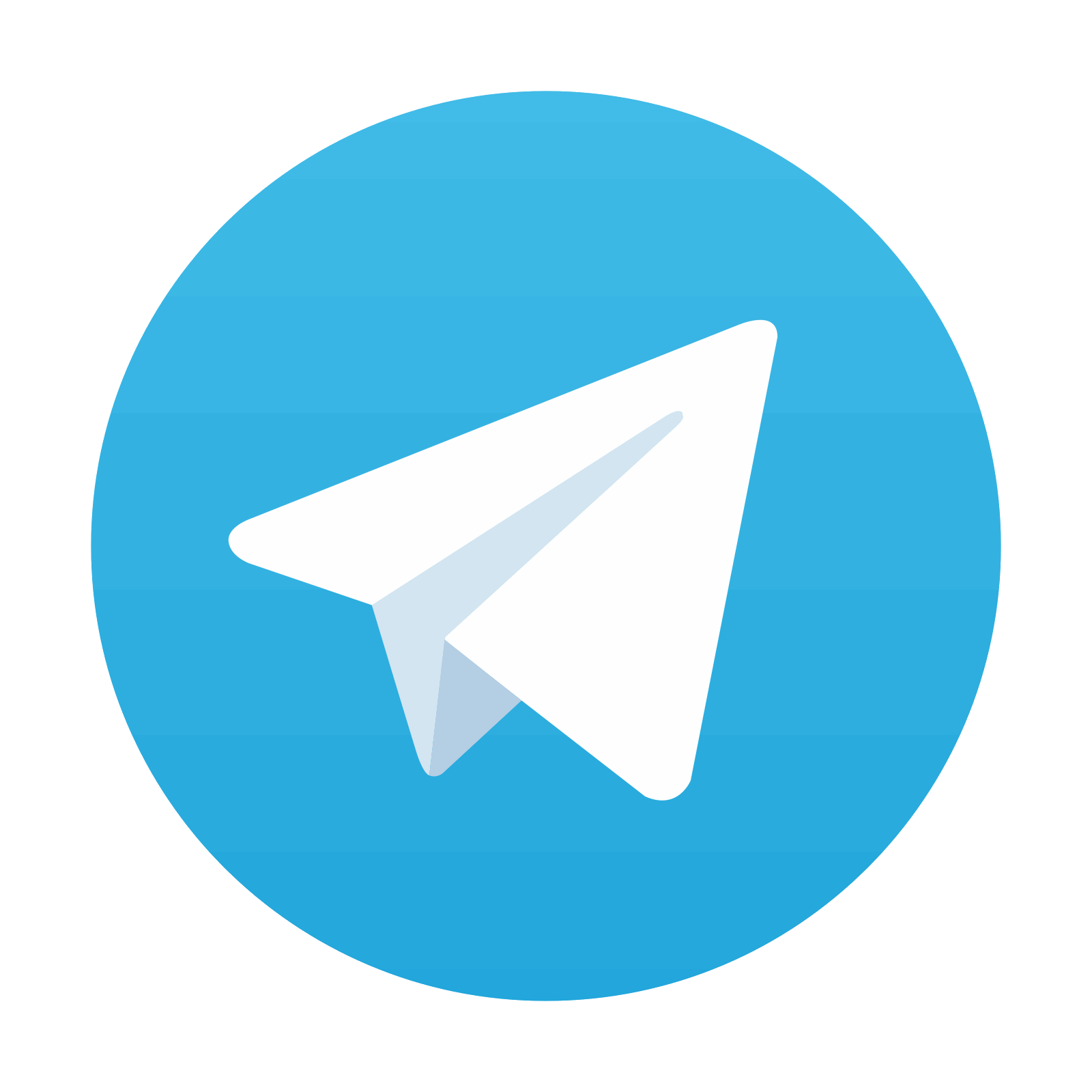
Stay updated, free articles. Join our Telegram channel

Full access? Get Clinical Tree
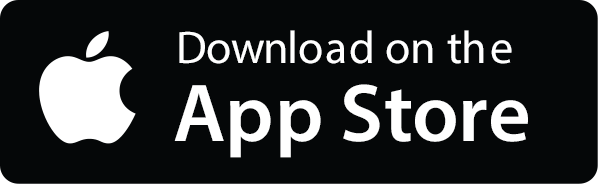
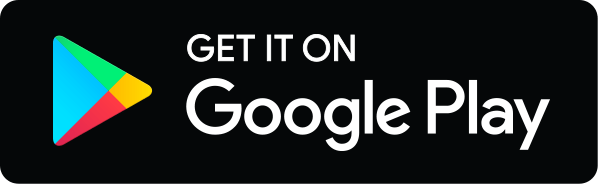