Key Points
- 1.
Pulmonary hypertension (PH) is a rare disease with a high degree of morbidity and mortality for patients undergoing noncardiac surgery.
- 2.
PH, although having several classification schemes, can be largely divided into high pulmonary venous pressure (typically from left-sided heart disease) and normal pulmonary venous pressure (typically from lung, embolic, or intrinsic disease).
- 3.
The morbidity and mortality from PH rest on the impact of significant afterload to the right ventricle, which normally works against a low-resistance circuit. The right ventricular (RV) dysfunction leads to the symptomatology of low cardiac output (CO) and venous congestion.
- 4.
The initial presenting symptom for a patient with PH is dyspnea on exertion caused by the inability of the right ventricle to provide an adequate CO in the presence of increasing afterload. Signs and symptoms of frank RV failure such as jugular venous distention, hepatomegaly, ascites, and peripheral edema are often late findings and portend a poor prognosis.
- 5.
The pillars of hemodynamic management of a patient with PH include maintaining coronary perfusion pressure via systemic vasopressors, reduction of pulmonary pressures via selective pulmonary vasodilation and maintenance of RV contractility via inodilators.
- 6.
During anesthesia, preventing increases in pulmonary vascular resistance (PVR) is imperative through avoidance of hypoxia, hypercarbia, and acidosis.
- 7.
Regional anesthetics such as neuraxial and peripheral techniques are useful as primary anesthetics or as adjuncts to general anesthesia. Care must be exercised in sedation during regional block placement or during monitored anesthesia care. Postoperative regional anesthesia may help to prevent postoperative pain with attendant increases in PVR as well as to reduce narcotic-induced hypoventilation.
- 8.
Several novel therapies aimed at reducing pulmonary pressures are used in patients preoperatively such as phosphodiesterase inhibitors and prostaglandins. These medications should be continued perioperatively because abrupt cessation may lead to lethal rebound PH.
- 9.
Echocardiography and right heart catheterization are key components in the preoperative workup. Both modalities provide insight into the right heart’s response to increased afterload as well as the potential use of pulmonary vasodilators perioperatively.
- 10.
Factors suggestive of perioperative decompensation include right atrial pressure greater than 12 mm Hg, RV end-diastolic pressure greater than 15 mm Hg, mean pulmonary artery pressure greater than 55 mm Hg, PVR greater than 1000 dynes • s • cm −5 , cardiac index less than 2 L/min per m 2 , and a lack of a vasodilator response.
- 11.
Patients with factors suggestive of decompensation should have elective cases delayed until the patient is hemodynamically optimized.
- 12.
Postoperatively, patients with severe PH require adequate monitoring in an intensive care unit to continue appropriate hemodynamic management as well as avoidance of hypoxia, hypercarbia, acidosis, and pain.
Pulmonary arterial hypertension (PAH) is a disorder in which flow to the pulmonary arterial circulation is constrained because of vascular remodeling and proliferation, resulting in increased pulmonary vascular resistance (PVR) and ending with right heart failure and death. PAH is a rare disease, with an estimated prevalence of 1% of the global population and an incidence of 1 to 2 cases per million people in the United States.
Pulmonary hypertension (PH), whether from PAH or another cause, is a known risk factor for perioperative complications, with an increased perioperative morbidity and mortality.
The choice of anesthetic technique together with patient and surgical characteristics are crucial factors in perioperative management. The main principles of perioperative management are to avoid systemic hypotension, as well as acute elevations in pulmonary arterial pressure, as both can lead to right ventricular (RV) ischemia and failure. This chapter focuses on perioperative management of pulmonary hypertension patients undergoing noncardiac surgery.
Classification of Pulmonary Hypertension
By definition, PH constitutes a mean pulmonary artery pressure (mPAP) greater than 25 mm Hg and a PVR greater than 300 dynes • s • cm −5 . Severe PH is defined as an mPAP greater than 50 mm Hg and a PVR greater than 600 dynes • s • cm −5 .
Pulmonary hypertension classification began in 1973 at the World Health Organization (WHO) conference and has since undergone multiple changes as the appreciation of the disease and treatment of PH has evolved. The Fourth World Symposium on PH held in 2008 in Dana Point, California, was the first international meeting to focus not only on PAH but also on other forms of PH caused by left heart disease, chronic lung disease, chronic venous thromboembolism, and other related diseases. Consensus during the recent Fifth World Symposium on PH was to maintain the same classification of PH into five distinct subgroups of patients sharing specific features ( Box 7.1 ).

- •
Group I: IPAH, heritable PAH, HIV infection, and other subtypes of PAH
- •
Group II: left heart disease, cardiomyopathies and LV failure, aortic and mitral valvular pathologies
- •
Group III: respiratory disease and hypoxemia, COPD, sleep disorders, and ILD
- •
Group IV: CTEPH
- •
Group V: miscellaneous causes
COPD, Chronic obstructive pulmonary disease; CTEPH, chronic thromboembolic pulmonary hypertension; HIV, human immunodeficiency virus; ILD, interstitial lung disease; IPAH, idiopathic pulmonary arterial hypertension; LV, left ventricular; PAH, pulmonary arterial hypertension.
Another classification of PH assessed by right heart catheterization (RHC) divides PH into precapillary and postcapillary groups. Precapillary PH (groups I, III, IV, and V of the Fifth World Symposium classification) is characterized by mPAP greater than 25 mm Hg with normal pulmonary capillary wedge pressure (PCWP; i.e., <15 mm Hg, with a PVR >300 dynes • s • cm −5 ). Postcapillary PH (group II), the most common form of PH, is caused by left heart disease and is characterized by mPAP greater than 25 mm Hg and PCWP greater than 15 mm Hg with normal PVR. Differentiating PAH from pulmonary venous hypertension (PVH) in group II is important given the high prevalence of left heart disease ( Table 7.1 ).
Pulmonary Arterial Hypertension | Pulmonary Venous Hypertension | |
---|---|---|
RA size | Enlarged | May be enlarged |
LA size | Small | Large |
Interatrial septum | Bows from right to left | Bows from left to right |
RVOT notching | Common | Rare |
Aortic pressure | Normal or low | Normal or high |
PCWP (mm Hg) | <15 | >15 |
PADP-PCWP (mm Hg) | >7 | <5 |
Idiopathic Pulmonary Arterial Hypertension
Idiopathic pulmonary arterial hypertension (IPAH) is a rare, debilitating disorder that affects the pulmonary vasculature, leading to PH and, consequently, right heart failure and death. IPAH, associated with a prothrombotic diathesis, excessive endothelial proliferation, and antiapoptotic cells within the vascular lumen, is identified when no other cause for the PH is identified. The endothelium displays an imbalance between vasoconstrictors relative to vasodilators.
“Plexogenic pulmonary arteriopathy” is the pathologic hallmark of primary PH, and it reflects a dysregulation of phenotypically altered endothelial growth.
Idiopathic pulmonary arterial hypertension is a panvasculopathy mostly affecting small and medium-size arterial vessels with a wide range of abnormalities, including intimal hyperplasia, medial hypertrophy, adventitial proliferation, and plexiform arteriopathy.
Pathogenesis
The pulmonary vasculature is a low-pressure, high-flow circuit balancing vasodilation and constriction, with a tendency toward vasodilation. The endothelium modulates vascular smooth muscle cell activity through the production of various vasodilators (prostacyclin and nitric oxide [NO]) and vasoconstrictors (thromboxane A 2 and endothelin). The endothelium of the lung is markedly different from endothelium of the systemic vasculature. Although the stimuli that trigger primary PAH may differ, an undefined injury to the endothelium occurs that causes increased coagulation, proliferation, and vasoconstriction that are instrumental to the development of PAH. Mediator imbalance results in pulmonary vasoconstriction that leads to the disordered endothelial cell proliferation in combination with proliferation of intimal cells that form the characteristic plexiform lesions.
There is, however, no specific histopathologic lesion that defines primary PAH. Evidence of proliferative and obliterative intimal lesions composed of myofibroblasts is found in the pulmonary arteries and arterioles from increased production of endothelial mediators. Additionally, thickened arterial smooth muscle (isolated medial hypertrophy), destruction and fibrosis of vessel walls, and in situ thrombosis lead to stiffening of the arteries and greater vasoconstriction. Eventually, the endothelial mediators that maintain the low-pressure, high-flow pulmonary circulation (NO, endothelium-derived hyperpolarizing factor, natriuretic peptides, adrenomedullin, and α 2 -agonists) are lost, and PA pressure increases at rest and with exercise. Regardless of the primary pathophysiologic pathways to PAH, a similar endpoint is reached.
Hypercoagulability is enhanced by increased platelet activity and elevated levels of serotonin, plasminogen activator inhibitor, and fibrinopeptide A together with a decrease in thrombomodulin levels. Endothelin (ET)-1, a potent vasoconstrictor, was found in high levels in arterial plasma compared with venous plasma in IPAH, suggesting that ET-1 may contribute to elevated PVR in these patients.
Idiopathic pulmonary arterial hypertension has been associated with increased local expression of ET-1 in pulmonary vascular endothelial cells, contributing to the pathogenesis of PAH. Additionally, the inhibition of voltage-gated (Kv) channels raises the membrane potential activating the voltage gated L-type calcium channel, which increases the calcium levels, leading to vasoconstriction and possibly initiating cell proliferation.
Clinical Manifestations
The diagnosis of PH is often significantly delayed because of the nonspecific symptoms that may mimic known underlying pulmonary or cardiac disorders. Gradual onset of shortness of breath is the earliest and most common symptom of PH reflecting an inability to increase cardiac output (CO) in proportion to activity. Right-sided chest pain is prevalent in PH despite normal coronary arteries. Additional symptoms include syncope, fatigue, and worsening peripheral edema. Hemoptysis may occur as a result of rupture of dilated and distended pulmonary vessels. Enlarged pulmonary arteries may compress the recurrent laryngeal nerve, leading to hoarseness in these patients.
Physical examination focuses on signs of PH, right ventricular hypertrophy (RVH), and right-heart failure ( Table 7.2 ). Accentuation of the pulmonic component of the second heart sound might be the only finding on physical examination and is easily overlooked. Hepatomegaly and peripheral edema, although less subtle on physical examination, typically signify advanced PH with concomitant right heart failure.
Sign | Implication |
---|---|
Physical Signs That Reflect the Severity of PH | |
Accentuated pulmonary component of S 2 (audible at apex in >90%) | High pulmonary pressure increases force of pulmonic valve closure |
Early systolic click | Sudden interruption of opening of pulmonary valve into high-pressure artery |
Midsystolic ejection murmur | Turbulent transvalvular pulmonary outflow |
Left parasternal lift | High RV pressure and hypertrophy present |
RV S 4 (in 38%) | High RV pressure and hypertrophy present |
Increased jugular a-wave | Poor RV compliance |
Physical Signs That Suggest Moderate to Severe PH | |
Holosystolic murmur that increases with inspiration | Tricuspid regurgitation |
Increased jugular v-waves | |
Pulsatile liver | |
Diastolic murmur | Pulmonary regurgitation |
Hepatojugular reflux | High central venous pressure |
Advanced PH with RV failure | |
RV S 3 (in 23%) | RV dysfunction |
Distention of jugular veins | RV dysfunction, tricuspid regurgitation, or both |
Hepatomegaly | RV dysfunction, tricuspid regurgitation, or both |
Peripheral edema (in 32%) | |
Ascites | |
Low blood pressure, diminished pulse pressure, cool extremities | Reduced CO, peripheral vasoconstriction |
Physical Signs That Suggest Possible Underlying Cause or Associations of PH | |
Central cyanosis | Abnormal V/Q, intrapulmonary shunt, hypoxemia, pulmonary-to-systemic shunt |
Clubbing | Congenital heart disease, pulmonary venopathy |
Cardiac auscultatory findings, including systolic murmurs, diastolic murmurs, opening snap, and gallop | Congenital or acquired heart or valvular disease |
Rales, dullness, or decreased breath sounds | Pulmonary congestion or effusion or both |
Fine rales, accessory muscle use, wheezing, protracted expiration, productive cough | Pulmonary parenchymal disease |
Obesity, kyphoscoliosis, enlarged tonsils | Possible substrate for disordered ventilation |
Sclerodactyly, arthritis, telangiectasia, Raynaud phenomenon, rash | Connective tissue disorder |
Peripheral venous insufficiency or obstruction | Possible venous thrombosis |
Venous stasis ulcers | Possible sickle cell disease |
Pulmonary vascular bruits | Chronic thromboembolic PH |
Splenomegaly, spider angiomata, palmary erythema, icterus, caput medusa, ascites | Portal hypertension |
The first step in diagnosing PH is having a high index of suspicion in patients with these described signs and symptoms or those at risk such as coexisting left heart disease or pulmonary disease. Chest radiography and electrocardiogram (ECG) may display markers indicative of PH such as enlarged pulmonary arteries or right-axis deviation, respectively, but with very low specificity. Provided there is a high index of suspicion from the history and physical examination, echocardiography is the most appropriate next step. Echocardiography is sensitive to the effects of PH on the right heart and estimation of pulmonary artery (PA) pressures is possible in the presence of tricuspid regurgitation. Additional details of the echocardiographic examination of patients with PH is included below. Computed tomography (CT) and magnetic resonance (MR) imaging are new techniques available to further explore the diagnosis. RHC with assessment of PVR and right atrial (RA) and ventricular pressures together with left-sided pressure estimation are required to confirm the diagnosis and to define the hemodynamic profile with greater precision. Fig. 7.1 outlines an algorithm for the evaluation of PAH.

The number of patients with PH related to pulmonary venous hypertension, chronic lung disease with hypoxemia, and thromboembolic disease pulmonary disease is far greater than the number of patients with IPAH. The term “secondary” PAH is being used less because of the many therapies for PAH regardless of etiology and often the underlying disease overshadows the clinical manifestations of PAH. After the etiology of PAH is identified, treatment should begin immediately with the goal of preventing the onset of right heart failure. Unfortunately, at presentation, PAH has often progressed to the point that the value of any treatment is limited to palliation of incapacitating symptoms. Decisions about whether institution of therapy and the specific therapy chosen are made on a case-by-case basis and are best performed at centers with experience in managing PH. One factor in the evaluation of patients includes an assessment of RV function, typically by echocardiography and RHC, because the presence of RV dysfunction plays a major role in prognosis.
Right Ventricular Pathophysiology: Effect of Pulmonary Hypertension on the Right Ventricle
The right ventricle is a unique, asymmetric, crescent-shape structure that is designed to accommodate the entire venous return while maintaining a low RA pressure and providing sustained low-pressure perfusion through the lungs. Normally, the RV receives coronary blood supply throughout the cardiac cycle (i.e., during systole and diastole) because of the continuous coronary perfusion pressure gradient between the aorta and the right ventricle. Caution must be applied when treating systemic hypertension in a patient with severe PH as this can compromise RV perfusion pressure, leading to ischemia in an already compromised ventricle.
Unlike the left ventricle, which generates high pressure to optimize organ perfusion, the right ventricle ejects blood from the right atrium to the lungs via the low-pressure, low-resistance, high-compliance circuit of the pulmonary vascular bed. Functionally and anatomically, the right ventricle is adapted for the generation of sustained low-pressure perfusion. The right ventricle is divided by a circular muscular band that separates the inflow portion; the sinus, which generates pressure during systole; and the outflow portion, the conus, which regulates this pressure. RV contraction occurs in three phases; first, contraction of the papillary muscles; then a bellows-like peristaltic movement of the RV free wall toward the interventricular septum (IVS); and, finally, contraction of the left ventricle causes a “wringing” type of motion of the IVS that further empties the RV. The net effect is the generation of pressure starting from the apex moving toward the compliant conus, which is able to decrease the peak RV and PA pressures, thereby prolonging ejection.
The RV tolerates small changes in venous return well without changing end-diastolic pressure or volume; however, this is not true with larger amounts of venous return. The RV pressure-volume loop has a triangular shape in contrast to the rectangular shape of the LV pressure-volume loop, meaning that the right ventricle has longer periods of ejection and shorter periods of isovolumetric contraction and relaxation. The prolonged low-pressure ejection of the right ventricle is very sensitive to changes in PA pressures as is seen with PH. Consequently, the RV pressure–volume loop adapts to the same rectangular shape as the left ventricular (LV) pressure-volume loop, with prolonged isovolumetric contraction and a shortened ejection time causing an increase in myocardial oxygen consumption. When acute or chronic RV dysfunction ensues, the thin-walled, crescent shape allows the RV to accommodate a large increase in preload with minimal change in RV end-diastolic pressure (RVEDP). Consequently, the initial primary compensatory mechanism of RV dysfunction is dilation that is usually well tolerated. Compared with the left ventricle, the normal right ventricle has half the wall thickness (~5 mm) and performs one quarter the stroke work. The IVS is responsible for approximately half the RV CO; in fact, during RV infarction with loss of the RV free wall, the septum continues to generate RV systolic pressure.
It is important to note that the afterload effect on RV systolic function is typically more significant than the afterload effect on LV performance. Increased RV afterload not only decreases the RV ejection fraction in a linear fashion; it also leads to increased RV wall tension and RV oxygen demand, thereby increasing susceptibility to ischemia. After RV afterload increases and ventricular dilation ensues, decreased RV oxygen supply compromises RV contractility. Normally, the IVS is shifted toward the RV free wall, contributing to RV ejection. However, when the RV pressure increases, the IVS paradoxically shifts toward the left ventricle, impairing its contribution to RV ejection. The decreased RV output and septal shift toward the LV result in decreased LV filling, decreased LV output, and systemic hypotension, further reducing RV perfusion and oxygen supply. Increasing the systemic vascular resistance (SVR) can help increase LV pressure, counter the septal shift, and thereby restore the contribution of the IVS to RV ejection. Systemic vasoconstrictors can therefore be used to increase RV ejection in addition to increasing coronary perfusion pressure.
Diagnostic Evaluation
The development of acute or chronic right heart failure is a devastating end-stage complication of PH that is associated with significant morbidity and mortality. Evidence of significant RV dysfunction should prompt a reevaluation of the need for elective surgery and will significantly impact the anesthetic management if the decision is made to proceed. Therefore a key component of the evaluation of PH is the assessment of the ability of the RV to compensate for the elevated afterload. There are several ways to diagnose right ventricular failure (RVF). In addition to the clinical signs and symptoms detailed earlier, which are very nonspecific, there are diagnostic modalities such as cardiac biomarkers, cardiac MRI, echocardiography, and RHC, which are more definitive and may reveal contributing causes ( Box 7.2 ). Cardiac MRI accurately assesses RV size and function, low RV stroke volume, and RV dilation; impaired LV filling independently predicts mortality.

RV Pressure Overload
- •
Pulmonary embolism
- •
Pulmonary hypertension
- •
Pulmonary stenosis
- •
Pericardial disease
- •
Left-sided valvular disease
- •
Left-sided cardiomyopathy
- •
RV outflow obstruction
- •
After cardiac and lung transplantation
RV Volume Overload
- •
Tricuspid regurgitation
- •
Pulmonary regurgitation
- •
Intracardiac shunt
RV, Right ventricular.
Predictors of survival after the first year of therapy with epoprostenol include functional class and improvement in exercise tolerance, cardiac index (CI), and mean PA pressure. A 6-minute walk test (6MW) was found to be an independent predictor of survival in several studies, leading to use of this test as the primary endpoint for many prospective trials. N-terminal pro–brain natriuretic peptide (NT-proBNP) levels are independent predictors of survival and correlate well with RV enlargement and dysfunction. The presence of cardiac troponin T potentially suggests RV ischemia and therefore confers poor prognosis ( Box 7.3 ).

ECG
- •
T inversion in leads V 1 to V 4 or lead III and aVF
- •
Sinus tachycardia
- •
New RBBB
- •
S1Q3T3 pattern
ECHO (+TEE)
- •
RV dilation (without mitral valve lesion and LV disease)
- •
RV free wall motion hypokinesia with sparing of apex (McConnell sign)
- •
Increased RV afterload
- •
Change to a more concentric RV morphology
- •
PH as TR with jet velocity >2.8 m/s
- •
Paradoxical septal motion
- •
60/60 sign
- •
Lack of inspiratory collapse of the IVC
- •
Pulmonary artery dilation and RA enlargement
Right Heart Catheterization
- •
Evidence of pulmonary arterial obstruction (precapillary pressure >20 mm Hg with PCWP <19 mm Hg)
- •
Impaired LV diastolic filling
Increased Levels of Biomarkers
- •
cTnT >0.07 µg/L
- •
proBNP ≥600 ng/L
cTnT, Cardiac troponin T; ECG, electrocardiogram; ECHO, echocardiogram; IVC, inferior vena cava; LV, left ventricular; PCWP, pulmonary capillary wedge pressure; PH, pulmonary hypertension; proBNP, pro–brain natriuretic peptide; RA, right atrial; RBBB, right bundle branch block; RV, right ventricular; TEE, transesophageal echocardiography; TR, tricuspid regurgitation.
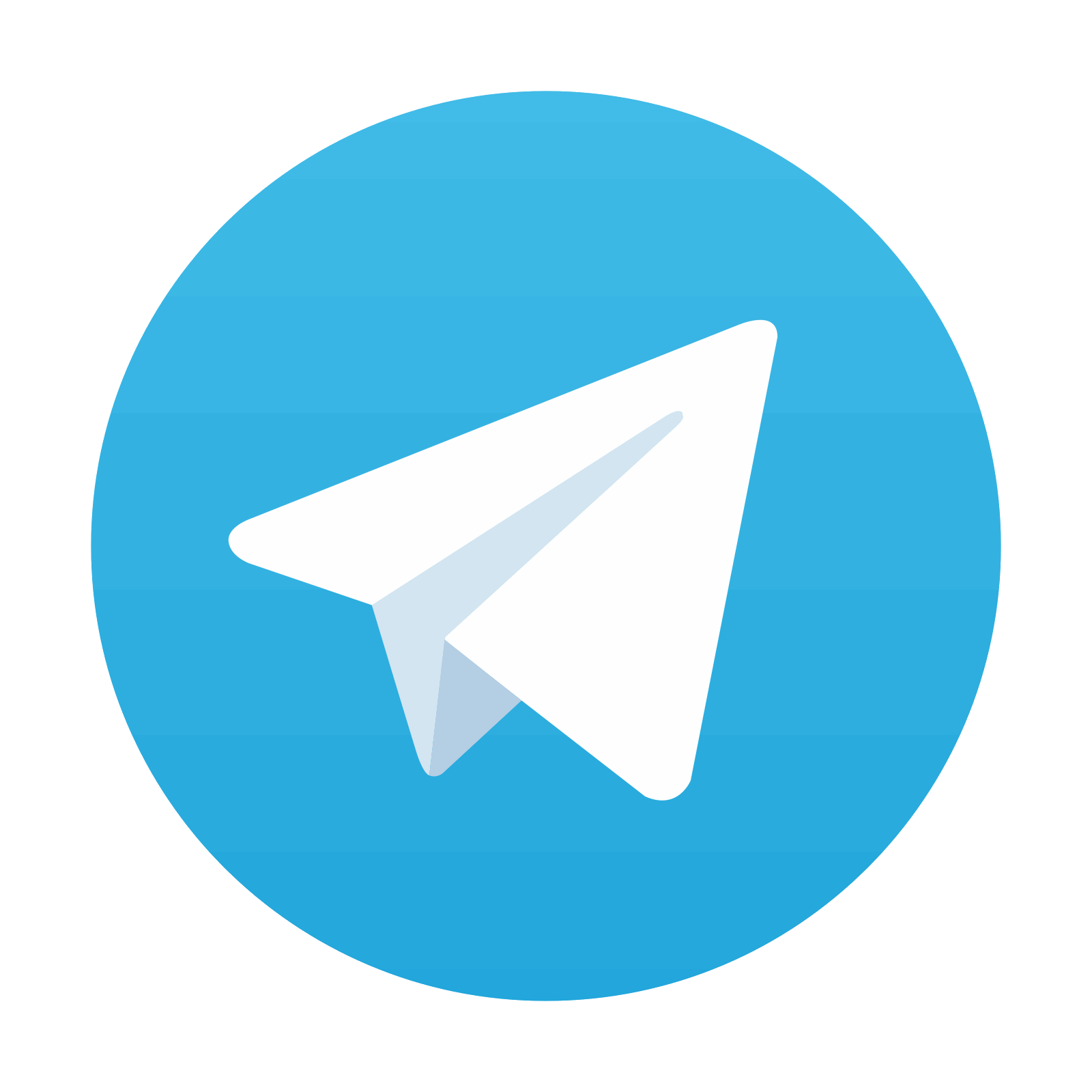
Stay updated, free articles. Join our Telegram channel

Full access? Get Clinical Tree
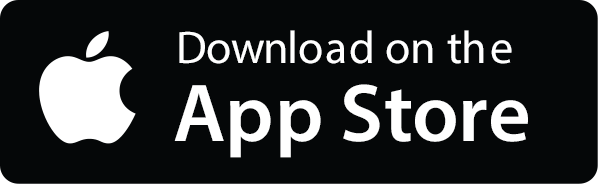
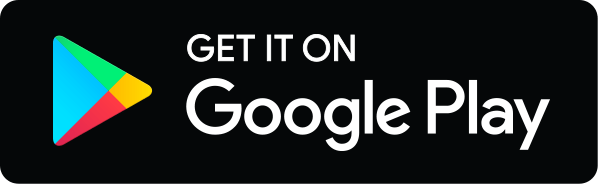
