Fig. 5.1
Group 2 pulmonary hypertension subcategories (left heart disease). Reproduced with permission [1]
Table 5.1
Definitions used in the description of pulmonary hypertension
Nomenclature | Description | Physiologic definition | Hemodynamic criteria in literature |
---|---|---|---|
Pulmonary hypertension (PH) | Sustained elevation of PAP at rest | Pre-capillary, post-capillary, mixed, high flow state | Mean PAP ≥ 25 mmHg (2 SD above normal) |
Pulmonary arterial hypertension (PAH) | PH with “normal” left-sided filling pressure | Pre-capillary vasoconstriction, remodeling, thrombosis-in-situ | Mean PAP ≥ 25 mmHg |
PCW, LAP, LVEDP ≤ 15 mmHg | |||
PVR > 3 WU | |||
Pulmonary venous hypertension (PVH) | PH with elevated left-sided filling pressure | Post-capillary passive congestion | Mean PAP ≥ 25 mmHg |
PCW, LAP, LVEDP > 15 mmHg | |||
TPG ≤ 12–15 mmHg | |||
PVR ≤ 2.5–3.0 WU | |||
Mixed PH or PH out-of-proportion to left-sided filling pressure | PH with elevated left-sided filling pressure and elevated pulmonary vascular resistance (PVH + PAH) | Pre- and post-capillary (passive congestion with excessive arterial vasoconstriction ± vascular remodeling) | Mean PAP ≥ 25 mmHg |
PCW, LAP, LVEDP > 15 mmHg | |||
TPG > 12–15 mmHg | |||
PVR > 2.5–3.0 WU | |||
Reversible, reactive, or vasoreactive PH | Component of mixed PH that is acutely or chronically responsive to pharmacologic (diuretics, vasodilators, inodilators) and/or mechanical circulatory support device therapies | With vasodilators/inodilators: | |
TPG ≤ 12–15 mmHg | |||
PVR ≤ 2.5–3.0 WU | |||
Irreversible, fixed, refractory, or persistent PH | Component of mixed PH that is not responsive to above strategies | Despite vasodilators/inodilators: | |
TPG > 12-15 mmHg | |||
PVR > 2.5–3.0 WU | |||
High-flow PH | PH with high cardiac output state or high pulmonary flow | Pre-, post-, or mixed depending on etiology (e.g., AV shunt, chronic anemia, thyrotoxicosis, nutritional or obesity, cardiomyopathies, congenital heart disease) | Mean PAP ≥ 25 mmHg |
PCWP, LAP, LVEDP variable | |||
TPG variable | |||
PVR variable | |||
High cardiac output |
Heart Failure with Reduced Ejection Fraction
Left ventricular systolic failure in the Western world is most commonly due to coronary artery disease or dilated cardiomyopathy due to systemic hypertension. A range of other heritable and acquired diseases and toxins can result in systolic failure. Presence of left ventricular systolic failure may result in exertional dyspnea, orthopnea, paroxysmal nocturnal dyspnea, cough which may be frothy in the setting of frank pulmonary edema, and development of concomitant right heart failure with corresponding signs and symptoms. Presence of pulmonary hypertension heralds a worse prognosis in patients with left ventricular (LV) systolic failure [2]. A study of patients with LV systolic failure who had ambulatory right ventricular pressure monitors implanted demonstrated that those patients who had subsequent clinical events had higher pulmonary artery systolic pressures, estimated pulmonary artery diastolic pressures, and right ventricular end-diastolic pressures than those who did not have such events [3]. For convenience, LV systolic failure is often abbreviated as HFrEF (heart failure with reduced ejection fraction), while HFrEF accompanied by pulmonary hypertension may be referred to as PHHFrEF (pulmonary hypertension with heart failure with reduced left ventricular ejection fraction).
Mechanisms of pulmonary hypertension in HFrEF include simple passive elevation in pulmonary artery pressure as a consequence of elevated left heart filling pressures. Presence of left heart filling pressure elevation may also induce vasoconstriction of the pulmonary vasculature, contributing a “reactive” component, further elevating the pulmonary artery pressure. This is described as “mixed” pulmonary hypertension. In such circumstances, acute administration of a vasodilator such as nitroprusside will often both correct the elevation in left heart filling pressure and dilate the pulmonary vasculature, ideally resulting in full normalization of the pulmonary artery pressure and pulmonary vascular resistance. In advanced LV systolic failure, particularly if volume overload is present, acute vasodilator challenge will often improve, but not normalize LV filling pressures; in such a situation, continued diuresis and potentially a period of inotropic support in the ICU will often further improve the hemodynamics. These techniques are routinely employed in assessing suitability of patients for cardiac transplantation, since irreversible elevation in pulmonary vascular resistance is a risk factor for right ventricular (RV) failure and impaired survival following transplantation [4]. Longstanding elevation in pulmonary venous pressure may result in remodeling of the pulmonary vasculature. In this situation, correction of the elevation in left heart filling pressure will not immediately fully correct the pulmonary artery pressure. The sine qua non of this situation is that seen in mitral stenosis, where very longstanding and severe pulmonary venous pressure elevation can result in pulmonary artery systolic pressures over 100 mmHg. Correction of the mitral stenosis will result in some immediate improvement in pulmonary artery pressure, but further improvement usually occurs with passage of time, apparently reflecting reverse remodeling of the pulmonary vasculature [5, 6]. The same phenomenon has been observed in patients undergoing left ventricular assist device implantation for refractory cardiac failure. For many of these patients, the pulmonary artery pressures approach normal values within days of device implant, while in some patients, continued fall will occur over additional months, thereby facilitating subsequent cardiac transplantation (Fig. 5.2) [7]. In occasional patients, device implantation does not result in resolution of the pulmonary hypertension, presumably either because of failure of reverse remodeling of the pulmonary vasculature, or because causes other than the prior elevation in venous pressure are driving the presence of the pulmonary hypertension and vasculopathy.
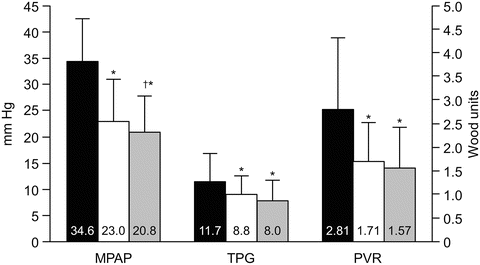
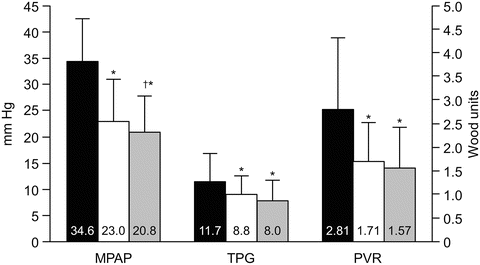
Fig. 5.2
Pulmonary hemodynamics in 58 patients at baseline and after left ventricular assist device (LVAD) support. Early improvements in hemodynamics were sustained during late support. The black, white, and striped bars represent baseline (median 1 day pre-implant), early (median 1 day post-implant) and late (median 75 days post-implant) LVAD support, respectively. Values are mean ± standard deviation. MPAP mean pulmonary artery pressure (mmHg), TPG transpulmonary gradient (mmHg), PVR pulmonary vascular resistance (Wood units); *p < 0.001 compared with baseline; † p = 0.028 compared with early LVAD support. Nair PK JHLT 2010:29. Reproduced with permission [7]
Pulmonary hypertension accompanying HFrEF can be analyzed based on a variety of hemodynamic parameters, including pulmonary artery (PA) systolic pressure, mean pulmonary artery pressure (MPAP), transpulmonary gradient (TPG) (defined as the difference between mean PA pressure and pulmonary capillary occlusion pressure), and pulmonary vascular resistance (PVR) (defined as transpulmonary gradient divided by cardiac output, and expressed in Wood units (mmHg × min/L), or TPG divided by cardiac index and then expressed in Wood units × m2. Multiplying by 80 converts the resistance parameter to dynes × s/cm5. A transpulmonary gradient of 12 mmHg or less is often used as a definition of purely passive pulmonary hypertension, while a TPG ≥ 12 mmHg refers to mixed pulmonary hypertension. Of course the significance of TPG is dependent on the accompanying cardiac output (CO); if the CO is high, an elevated TPG can occur in the presence of normal PVR. The higher the transpulmonary gradient in the setting of HFrEF, the worse the prognosis; a TPG greater than 18 mmHg has recently been associated with particularly increased risk [2]. Gradients in risk are also seen with mean pulmonary artery pressure (MPAP), pulmonary capillary wedge pressure (PCWP), PVR, and pulmonary artery compliance defined as stroke volume divided by pulmonary artery pulse pressure, with a PA compliance ≤2 mL/mmHg demonstrating particularly increased risk (Figs. 5.3 and 5.4).
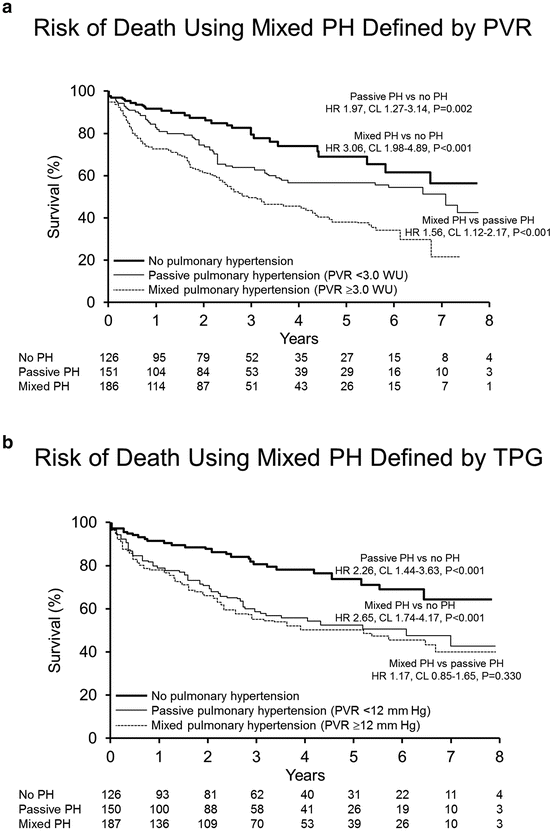
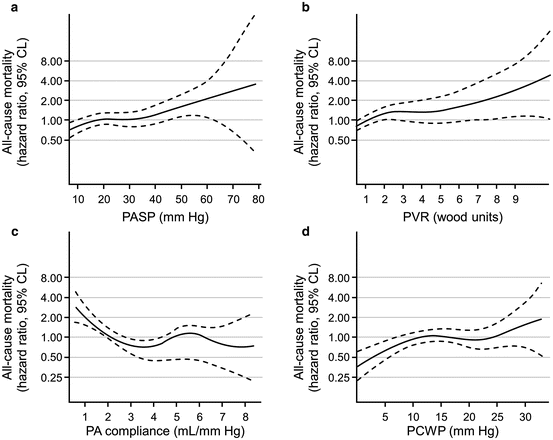
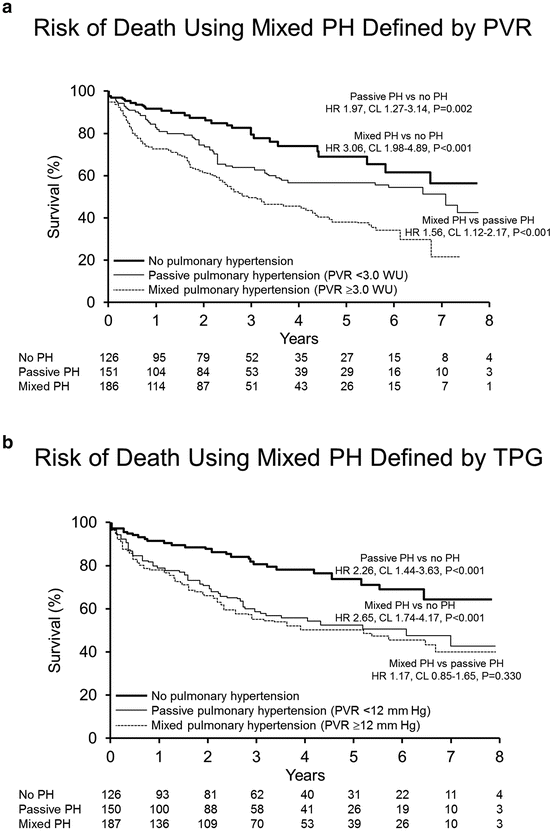
Fig. 5.3
Survival of HFREF patients with PH. (a) Kaplan–Meier estimates of survival in patients with heart failure of reduced left ventricular ejection fraction (HFREF) relative to passive pulmonary hypertension (PH) and mixed PH as defined by pulmonary vascular resistance (PVR) < or ≥3.0 Wood units (WU) and no PH. (b) Kaplan–Meier estimates of survival in patients with HFREF relative to passive PH and mixed PH as defined by transpulmonary gradient (TPG) < and ≥12 mmHg and no PH. Reproduced with permission [2]
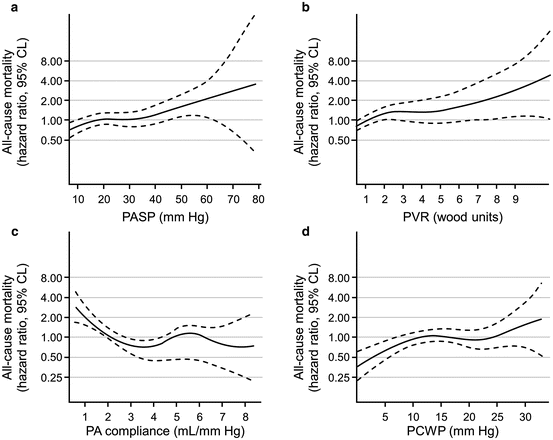
Fig. 5.4
All-cause mortality and hemodynamic parameters. (a) Unadjusted risk (hazard ratio [HR]: 95 % confidence limits [CL]) of all-cause mortality for sPAP analyzed as continuous variables. (b) Unadjusted risk (HR: 95 % CL) of all-cause mortality for PVR analyzed as continuous variables. (c) Unadjusted risk (HR: 95 % CL) of all-cause mortality for PAC analyzed as continuous variables. (d) Unadjusted risk (HR: 95 % CL) of all-cause mortality for PCWP analyzed as continuous variables. PA pulmonary artery, PASP pulmonary artery systolic pressure, PCWP pulmonary capillary wedge pressure, PVR pulmonary vascular resistance. Reproduced with permission [2]
The difficult conundrum of biventricular failure has resulted in a search for approaches to treatment that includes consideration of pulmonary vasodilators. Currently such an approach must be considered unproven, and not without risk. In addition, the attractiveness of such an approach may interfere with recognition of the tremendous value of optimization of conventional heart failure therapies in such patients. Table 5.2 shows hemodynamic measurements before and after nitroprusside administration in the catheterization laboratory in a patient with idiopathic dilated cardiomyopathy who was referred for discussion regarding management of pulmonary hypertension in the context of what was felt to be optimally managed heart failure including ACE inhibition, beta-blockade, digoxin and diuretics. The dramatic hemodynamic response to nitroprusside demonstrated the potential for additional systemic vasodilator therapy. The patient responded very favorably to gradual up titration of the ACE inhibitor and adjustment of diuretic dose.
Table 5.2
Acute hemodynamic effects of nitroprusside in a patient with LV systolic failure and mixed pulmonary hypertension
Blood pressure | RA | PA | PCW | TPG | CO/CI | PVR | |
---|---|---|---|---|---|---|---|
Baseline | 120/74 | 21 | 88/35/57 | 26 | 31 | 5.7/2.8 | 5.5 |
NTP | 100/37 | 13 | 47/20/31 | 16 | 15 | 7.0/3.5 | 2.1 |
Elevation of the pulmonary capillary wedge pressure in patients with left heart failure also has adverse effects on pulmonary arterial compliance. PA compliance reflects the ability of the pulmonary vasculature to accept an incoming bolus of volume from the ejecting right ventricle during systole. Ordinarily, there is a tight hyperbolic inverse relationship between pulmonary arterial compliance and resistance. However, for any given pulmonary vascular resistance, a rise in the pulmonary capillary wedge pressure will reduce the pulmonary arterial compliance, making it more difficult for the RV to eject into the pulmonary bed (Fig. 5.5) [8].
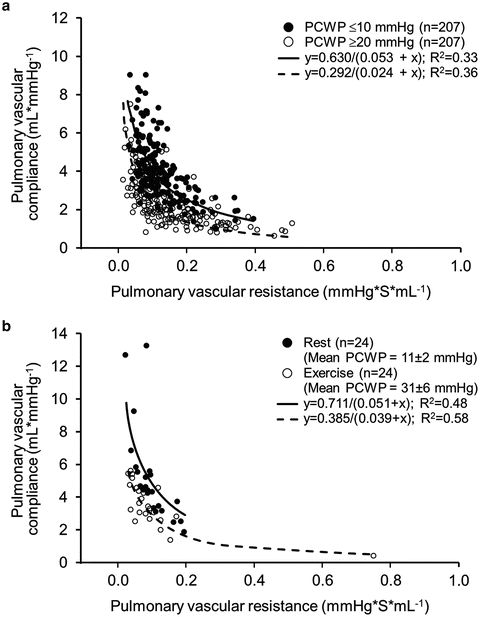
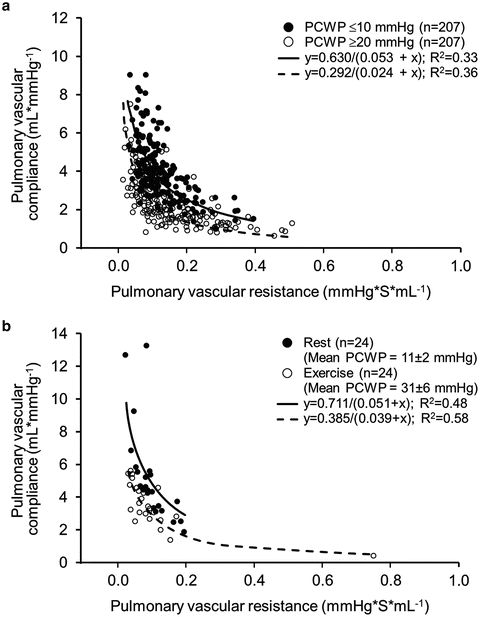
Fig. 5.5
Effect of pulmonary vascular resistance–compliance relationship (RPA–CPA) relationship by change in pulmonary capillary wedge pressure (PCWP) within an individual patient. (a) RPA–CPA for each patient is plotted at two different study times: at a low PCWP (PCWP ≤ 10 mmHg) and at a high PCWP (PCWP ≥ 20 mmHg). (b) Effect of increasing PCWP during supine exercise on RPA-CPA in 24 patients with early heart failure with preserved ejection fraction. Reproduced with permission [8]
The tendency to underutilize conventional heart failure therapy may in part explain the heralded success of pulmonary artery pressure monitoring in improving pulmonary hypertension and reducing heart failure hospitalizations in New York Heart Association (NYHA) Class III patients with LV systolic failure demonstrated in the CardioMEMS Heart Sensor Allows Monitoring of Pressure to Improve Outcomes in NYHA Class III Heart Failure Patients (CHAMPION) study. Patients in the active monitoring group ended up on higher doses of angiotensin-converting enzyme inhibitors and beta-blockers, and were more likely to end up on nitrates, also suggesting that more active titration of a standard heart failure program is advantageous over a more passive approach [9]. The take-home message here is that the most proven approach to pulmonary hypertension in the setting of LV systolic failure is to actively adjust standard heart failure therapies to optimal levels [8].
Other factors which may contribute to pulmonary hypertension in left heart failure include sleep disordered breathing, which may be central, obstructive, or mixed, and is quite common. Hypoxemia of any cause will aggravate pulmonary hypertension and right heart failure, and should be aggressively sought out and corrected. The possibility of concomitant pulmonary emboli must also be carefully considered.
Patients with HFrEF who have a more exaggerated rise in pulmonary artery pressures during exercise have worse exercise capacity and outcomes compared to patients without such a response [10]. In patients with PHHFrEF despite what is felt to be optimal medical therapy, case series and small randomized trials suggest the potential for phosphodiesterase-5 inhibition (PDE5i) to improve exercise tolerance and cardiac output response to exercise [11]. These encouraging preliminary data resulted in an NIH-funded multicenter study that was to have examined the impact of PDE5i with tadalafil in patients with PHHFrEF despite optimal medical therapy (Pitch-HF). Unfortunately this trial was not completed. It is hoped this concept will be studied further in the future. At the present time, use of PDE5i in PHHFrEF cannot be endorsed outside of research protocols.
Riociguat, the first in a new class of drugs which act via stimulation of soluble guanylate cyclase, was recently studied in a placebo-controlled, randomized trial of patients with PHHFrEF [12]. This trial did not reach its prespecified primary endpoint of a reduction in mean pulmonary artery pressure, but did result in a drop in pulmonary vascular resistance and systemic vascular resistance, with improvement in cardiac output and in Minnesota Living with Heart Failure scores [13]. Given the failure of the study to meet its primary endpoint, these additional analyses must be considered hypothesis generating, and suggest that additional studies of riociguat with more wisely chosen primary endpoints may be of value.
Heart Failure with Preserved Ejection Fraction
Approximately half of heart failure occurs in patients with preserved left ventricular ejection fraction (HFpEF). Most of these patients have accompanying pulmonary hypertension, and the severity of pulmonary hypertension is associated with prognosis [14]. Patients presenting with a syndrome compatible with HFpEF need careful scrutiny in an effort to identify specific diseases that may require very different management strategies. These include infiltrative cardiomyopathies such as amyloidosis, restrictive cardiomyopathy, and constrictive pericarditis. Traditional therapy for HFpEF includes optimal blood pressure control and use of diuretics. There are no proven treatments for HFpEF. A small randomized study of sildenafil in patients with HFpEF suggested improvement in hemodynamics, but the patient population was very unusual and very different from the usual HFpEF phenotype, having right atrial pressures on average over 20 mmHg, so the applicability of such results is highly questionable [15]. The robustly designed and executed, NIH-funded RELAX trial of PDE5i in HFpEF was recently completed [16, 17]. In this study, patients were randomized to receive sildenafil or placebo. The primary endpoint was peak oxygen consumption on cardiopulmonary exercise testing. Presence of pulmonary hypertension was not necessary for study inclusion, but many of the patients did have pulmonary hypertension. Sildenafil had no effect on peak oxygen consumption, 6 min walk, nor a composite hierarchical endpoint that included death, hospitalization for cardiovascular or renal causes, and quality of life. Furthermore, there were statistically significant adverse changes in creatinine, cystatin C, uric acid, N-terminal pro brain natriuretic peptide, and endothelin-1. Accordingly, PDE5i as a treatment strategy cannot currently be endorsed for use in patients with HFpEF. The search for differing therapeutic approaches to HFpEF must continue, but is complicated by the divergent phenotypic pictures of HFpEF, and the fact that such patients are at substantial risk of clinical events not directly related to their HFpEF.
The need to identify and treat other causes of pulmonary hypertension such as pulmonary emboli, hypoxemia, and sleep-disordered breathing in patients with HFpEF applies in a fashion similar to that discussed with regard to HFrEF. Key points regarding pulmonary hypertension in left heart disease are summarized in Table 5.3.
Table 5.3
Key points regarding pulmonary hypertension in left heart disease
• Exclude superimposed thromboembolic disease. Ventilation/perfusion lung scanning is an excellent tool in this regard, avoiding risk of contrast nephropathy, and being highly sensitive for thromboembolism |
• Actively seek and correct nocturnal and daytime hypoxemia. Sleep-disordered breathing is common in left heart disease, and will aggravate the biventricular failure |
• Optimize left heart filling pressures by methodical titration of left heart therapies including vasodilators and diuretics |
• Right heart catheterization with acute nitroprusside challenge can be helpful in understanding the extent to which optimization of vasodilator therapy may improve pulmonary hypertension and cardiac output. Additional correction of volume overload will further improve both LV filing pressures and pulmonary hypertension |
• Regarding PH with Heart Failure with reduced Ejection Fraction: |
– In advanced HFrEF, it may not be possible acutely to correct left heart filling pressures adequately to know whether the pulmonary hypertension would be reversible if this could be achieved. This is especially relevant in patients being considered for heart transplantation. Leaving a pulmonary artery catheter in place and using a combination of inotropes and intravenous diuretics for a few days may further clarify reversibility of the pulmonary hypertension. In some cases placement of a left ventricular assist device as a “bridge to decision” may be necessary to clarify reversibility of pulmonary hypertension with normalization of LV filling pressures
![]() Stay updated, free articles. Join our Telegram channel![]() Full access? Get Clinical Tree![]() ![]() ![]() |