Background
Measuring mean pulmonary artery pressure by right-heart catheterization is the gold standard for pulmonary hypertension (PH) diagnosis. However, its invasiveness and complication leads to its limited use. The aim of this study was to determine whether echocardiography-derived pulmonary artery acceleration time (PAAT) possesses adequate diagnostic performance for PH, using right-heart catheterization as a reference standard.
Methods
MEDLINE, Embase, PubMed, and the Cochrane Central Register of Controlled Trials were searched through July 2016 for studies evaluating PAAT for the diagnosis of PH. Methodologic quality was assessed using the Quality Assessment of Diagnostic Accuracy Studies tool. For each study, the sensitivity, specificity, and diagnostic odds ratio, along with 95% CIs, were calculated to determine the diagnostic accuracy of PAAT. Meta-regression was conducted to evaluate the impact of potential confounding factors.
Results
Of 430 articles, 21 studies (1,280 patients) were identified, including three studies that used transesophageal echocardiography and 18 studies that used transthoracic echocardiography. The pooled sensitivity across studies was 0.84 (95% CI, 0.75–0.90), the pooled specificity was 0.84 (95% CI, 0.78–0.89), and the pooled diagnostic odds ratio was 28 (95% CI, 16–49). The arrhythmia ratio in the population did not affect the specificity of PAAT’s diagnostic performance and increased the sensitivity of PH detection.
Conclusions
The results of this study suggest that PAAT is useful for PH detection.
Highlights
- •
PAAT measured by echocardiography has high pooled sensitivity and specificity across studies in PH prediction, regardless of etiology.
- •
The location of PAAT measurement did not affect its diagnostic performance.
- •
The arrhythmia ratio in the population did not affect the specificity of PAAT’s diagnostic performance.
Pulmonary hypertension (PH) is a clinically significant disease, and its severity reflects different prognosis. Patients with PH carry a high risk for cardiovascular morbidity and mortality. Early diagnosis and treatment for PH is important, because it can slow disease progression. PH is defined by a mean pulmonary artery pressure (PAP) > 25 mm Hg at rest. Measuring PAP usually requires right-heart catheterization, and right-heart catheterization carries a certain risk for complications. Echocardiography is less invasive and is an alternative choice for PAP estimation. The most commonly used echocardiographic technique for PAP estimation is measurement of the pressure gradient between the right ventricle and right atrium from tricuspid regurgitation (TR). However, in about 40% of patients, an adequate Doppler signal cannot be obtained, and in half of patients, estimation error is >15%. The pulmonary artery acceleration time (PAAT) is the time interval from the beginning of right ventricular (RV) ejection to peak flow velocity across the pulmonary valve. The concept that flow accelerates more rapidly in the RV outflow tract (RVOT) in patients with PH than in those with normal PAP was first reported in 1983 by Kitabatake et al. Measuring PAAT is technically easy, and many studies have shown that mean PAP and PAAT have a negative curvilinear relationship, across a range of pulmonary pressures.
Previous studies measured PAAT in patients with different etiologies of, and the measurement locations differed among these studies. Many studies derived regression equations from their analyses, but none of the pulmonary pressure estimation equations was the same. Therefore, the aim of this study was to evaluate the diagnostic performance of PAAT in PH, using right-heart catheterization or pulmonary artery catheterization as the reference standard, in adult patients.
Methods
We performed a systematic literature search without language restrictions of the electronic databases MEDLINE, Embase, PubMed, and the Cochrane Central Register of Controlled Trials, for human studies up to and including July 2016. Our searches used the term “pulmonary artery hypertension” or “pulmonary hypertension” in combination with “acceleration time” or “pulmonary artery acceleration time.” The articles identified during the literature search process were first screened by examining their titles and abstracts. The screening process was undertaken independently by two authors (Y.-C.W. and C.-H.H.). Disagreement was resolved by discussion and consensus. Article titles and abstracts were reviewed for eligibility. Moreover, the reference lists of retrieved articles and reviews were screened for relevant studies. Authors were contacted through e-mail in cases of queries or missing information regarding their published studies. A study was included if it met the following criteria: it assessed PAAT as a diagnostic tool to evaluate patients for the presence of PH; PH was defined by pulmonary artery catheterization as the reference test; the inclusion subjects were >18 years of age; and the absolute numbers of true-positive, false-positive, true-negative, and false-negative findings were available, or these data were derivable from the results presented. A study was excluded if it was a conference article, was a review, or was not conducted in humans. Studies published only as abstracts or letters were also excluded.
Extracted information included author, journal, year of publication, and country; details of study design; patient demographic features (such as number of patients, mean age, percentage of male patients, and percentage of patients with arrhythmia), diagnostic tool (transthoracic echocardiography [TTE] or transesophageal echocardiography [TEE]), location of PAAT measurement (RVOT, main pulmonary trunk, or branched pulmonary arteries), etiology of PH, and numbers of true-positive, false-positive, true-negative, and false-negative findings.
PAAT was measured from the onset of systolic pulmonary artery flow to peak velocity using pulsed-wave Doppler. PAAT could be measured using either TTE or TEE. For TTE, patients were placed in the left lateral decubitus position, and the pulmonary artery could be obtained in the upper esophageal transverse pulmonary artery view.
For TEE, PAAT could be measured in the main pulmonary trunk in the upper esophageal view or midesophageal short-axis view. The other choice for measurement is to use the transgastric RV inflow outflow view to align the cursor with the RVOT. Examples of PAAT measurements are shown in Figure 1 . PH was defined as a mean PAP > 25 mm Hg by right-heart catheterization in our analysis according to 5th World Symposium on PH. Some studies used mean PAP > 20 mm Hg as the cutoff point for the diagnosis of PH. We retained the trials that enabled us to retrieve the original data and recalculated the cutoff point as mean PAP > 25 mm Hg.

Methodological quality was assessed using the Quality Assessment of Diagnostic Accuracy Studies (QUADAS) tool (scale, 0–14). In brief, the assessment was based on 14 items, including covered patient spectrum, reference standard, disease progression bias, verification bias, review bias, clinical review bias, incorporation bias, test execution, study withdrawals, and indeterminate results. A score of 7 of 14 indicates high quality, and scores < 7 indicate low quality. The QUADAS tool is an evidence-based quality assessment tool to be used in systematic reviews of diagnostic accuracy studies.
For extracted data, categorical variables were expressed as percentages, and continuous variables were expressed as mean values. On the basis of the extracted 2 × 2 contingency tables, pooled measures for diagnostic performance, including sensitivity, specificity, diagnostic odds ratio, summary receiver operating characteristic (ROC) curve, and area under the curve (AUC) with 95% CIs, were calculated. Between-study statistical heterogeneity was assessed using I 2 and the Cochrane Q test on the basis of the mixed-effects analysis. Publication bias was examined using the associated regression test of asymmetry described by Deeks et al., with a P value < .10 for the slope coefficient indicating significant asymmetry.
We used bivariate multilevel logistic regression for random-effect meta-analysis. The parameters of the bivariate model were estimated in a single model, so the potential correlations between sensitivity and specificity could be taken into account. The summary ROC curves were also created using this model to estimate the AUC. We also conducted meta-regression regarding sex, percentage of PH, percentage of pulmonary arterial hypertension, cutoff value for PAAT, arrhythmia, use of TTE or TEE, and PAAT measurement site. We wanted to evaluate the influence of these factors on the diagnostic power of PAAT. Statistical analysis was performed using STATA version (StataCorp, College Station, TX).
Results
The primary literature research yielded 430 publications. After excluding duplicates from different electronic databases and conference articles, we identified 126 articles. By screening the titles and abstracts, an additional 96 articles were excluded. After review of the full text, 10 articles were excluded. The flowchart of the screening process is presented in Figure 2 .
Eventually, 21 articles published between 1983 and 2016, representing a total of 1,280 patients, were included in our systematic review. The main characteristics of the trials included in the systematic review and retained for meta-analysis are summarized in Table 1 and Supplemental Table 1 (available at www.onlinejase.com ). One fourth of the studies did not report the etiology of PH. Reported etiologies included left-heart disease, chronic hypoxia, and pulmonary arterial hypertension. Seven studies included patients in sinus rhythm. In the rest of the included studies, the arrhythmia ratio ranged from 28% to 45.7%. Three studies used TEE for PAAT measurements, and the others used TTE.
Study | Year | Country | Sample size | PH (%) | PAH (%) | TTE/TEE | Arrhythmia ratio (%) | Cutoff value (sec) | PAAT | Sensitivity (95% CI) | Specificity (95% CI) | |||
---|---|---|---|---|---|---|---|---|---|---|---|---|---|---|
TP | FP | FN | TN | |||||||||||
Cowie et al. | 2016 | Belgium | 98 | 40.4 | 0 | TEE | 0 | 107 | 30 | 3 | 10 | 55 | 0.75 (0.59–0.87) | 0.95 (0.86–0.99) |
Sohrabi et al. | 2016 | Iran | 300 | 80.7 | 0 | TTE | 38 | 104 | 231 | 21 | 11 | 37 | 0.95 (0.92–0.98) | 0.64 (0.50–0.76) |
Tousignant et al. | 2015 | Canada | 74 | 19.4 | 0 | TEE | 0 | 90 | 8 | 13 | 6 | 47 | 0.57 (0.29–0.82) | 0.78 (0.66–0.88) |
Tossavainen et al. | 2013 | Sweden | 56 | 62.5 | 57.1 | TTE | 0 | 90 | 21 | 4 | 5 | 26 | 0.81 (0.61–0.93) | 0.87 (0.69–0.96) |
Granstam et al. | 2013 | Sweden | 29 | 66 | 38 | TTE | 0 | 100 | 17 | 2 | 3 | 7 | 0.85 (0.62–0.97) | 0.78 (0.40–0.97) |
Serra et al. | 2010 | Italy | 19 | 52.6 | 52.6 | TTE | 0 | 100 | 4 | 0 | 6 | 9 | 0.40 (0.12–0.74) | 1.00 (0.66–1.00) |
Lanzarini et al. | 2005 | Italy | 86 | 57.0 | 5 | TTE | 0 | 93 | 34 | 13 | 15 | 24 | 0.69 (0.55–0.82) | 0.65 (0.47–0.80) |
Yamasa et al. | 1993 | Japan | 32 | 18.8 | 0 | TEE | 0 | 95 | 1 | 0 | 5 | 26 | 0.17 (0.00–0.64) | 1.00 (0.87–1.00) |
Campos Filho et al. | 1993 | Brazil | 61 | 70.5 | 6.6 | TTE | 31.3 | 100 | 38 | 1 | 5 | 17 | 0.88 (0.75–0.96) | 0.94 (0.73–1.00) |
Mirrakhimov et al. | 1992 | Kyrgyzstan | 36 | 30.6 | 0 | TTE | 31.8 | 101 | 10 | 3 | 1 | 22 | 0.91 (0.59–1.00) | 0.88 (0.69–0.97) |
Tramarin et al. | 1991 | Italy | 98 | 38.1 | 0 | TTE | 0 | 80 | 28 | 11 | 9 | 50 | 0.76 (0.59–0.88) | 0.82 (0.70–0.91) |
Liu et al. | 1991 | China | 18 | 88.9 | 0 | TTE | 0 | 100 | 13 | 0 | 3 | 2 | 0.81 (0.54–0.96) | 1.00 (0.16–1.00) |
Corinaldesi et al. | 1991 | Italy | 19 | 73.7 | 0 | TTE | 0 | 100 | 14 | 2 | 0 | 3 | 1.00 (0.77–1.00) | 0.60 (0.15–0.95) |
Hu et al. | 1990 | China | 17 | 100 | 11.8 | TTE | 29 | 100 | 13 | 0 | 4 | 0 | NA | NA |
Niederle et al. | 1989 | Czechoslovakia | 50 | 56 | 4 | TTE | 0 | 115 | 14 | 5 | 1 | 30 | 0.93 (0.68–1.00) | 0.86 (0.70–0.95) |
Eysmann et al. | 1989 | United States | 26 | 100 | 100 | TTE | 0 | 90 | 24 | 0 | 2 | 0 | NA | NA |
Torbicki et al. | 1989 | Poland | 74 | 44.3 | <9 | TTE | 0 | 79.5 | 24 | 6 | 9 | 35 | 0.73 (0.54–0.87) | 0.85 (0.71–0.94) |
von Bibra et al. | 1987 | Germany | 70 | 44.3 | 5.7 | TTE | 45.7 | 90 | 29 | 8 | 2 | 31 | 0.94 (0.79–0.99) | 0.79 (0.64–0.91) |
Dabestani et al. | 1987 | United States | 39 | 41 | 6.7 | TTE | 28 | 104 | 15 | 4 | 1 | 19 | 0.94 (0.70–1.00) | 0.83 (0.61–0.95) |
Isobe et al. | 1986 | Japan | 45 | 53.5 | 8.9 | TTE | 44.4 | 109 | 25 | 5 | 0 | 15 | 1.00 (0.86–1.00) | 0.75 (0.51–0.91) |
Kitabatake et al. | 1983 | Japan | 33 | 39.4 | 6.1 | TTE | 39.4 | 103 | 12 | 3 | 1 | 17 | 0.92 (0.64–1.00) | 0.85 (0.62–0.97) |
Summary estimates | 0.84 (0.75–0.90) | 0.84 (0.78–0.89) |
The methodologic quality of the 21 studies was assessed using the QUADAS tool. Review of the QUADAS checklist for all studies showed that all studies (21 of 21) were scored >7, which is considered good quality. During QUADAS assessment, most studies were found to have problems with the representativeness of included patients, questionable time gaps between index test and reference test, unclear masking during interpretation of the reference test, masked reading of the index test, and a lack of reporting for uninterpretable results, which may have resulted in bias ( Supplemental Table 2 , available at www.onlinejase.com ).
The I 2 index showed substantial heterogeneity with regard to sensitivity and moderate heterogeneity with regard to specificity for all index tests. The PAAT cutoff point for PH prediction is listed in Table 1 . Most studies (30.4%) used 100 msec as their cutoff point, followed by 90 msec (17.4%) and 104 msec (13.0%). Of the total 21 studies, sensitivity was >90% in nine studies and <50% in three studies. The specificity for all the index tests was >60%. The funnel plot and regression tests showed a statistically nonsignificant P value of .78 for the slope coefficient, indicating symmetry in the data and a low likelihood of publication bias ( Figure 3 ).
The pooled sensitivity across studies for PAAT was 0.84 (95% CI, 0.75–0.90), and the pooled specificity across studies was 0.84 (95% CI, 0.78–0.89; Table 1 , Figure 4 ). The pooled positive diagnostic likelihood ratio across studies was 5.26 (95% CI, 3.86–7.17), and the pooled negative diagnostic likelihood ratio was 0.19 (95% CI, 0.12–0.30). The diagnostic odds ratio was 28 (95% CI, 16–49). The summary ROC curves are shown in Figure 5 A. The global AUC across all included studies was 0.90 (95% CI, 0.83–0.95).
Eight studies compared both PAAT and tricuspid valve regurgitation pressure gradient (TVPG) with right-heart catheterization ( Table 2 ). PAAT measurement was successful for >90% of the patients in all studies. The successful rate for TVPG measurement varied across studies. The feasibility of TVPG measurement ranged from 24% to 86.2%, and the reasons for failure included insufficient TR, eccentric jet, and inadequate regurgitation flow contour. The pooled sensitivity across studies for TVPG in PH diagnosis was 0.93 (95% CI, 0.90–0.95), and the pooled specificity across studies was 0.75 (95% CI, 0.63–0.83). The summary ROC curves are shown in Figure 5 B. The global AUC across studies with TVPG was 0.93 (95% CI, 0.89–0.95).
Study | n | PH (%) | TTE/TEE | PAAT feasibility (%) | TVPG feasibility (%) | Echocardiographic cutoff point | Catheterization criteria | TR TP | TR FP | TR FN | TR TN |
---|---|---|---|---|---|---|---|---|---|---|---|
Sohrabi et al. (2016) | 300 | 80.7 | TTE | 98.6 | 72.9 | TRVTI | mPAP > 25 mm Hg | 234 | 14 | 15 | 37 |
Granstam et al. (2013) | 29 | 69.0 | TTE | 100 | 86.2 | TVPG > 30 mm Hg | sPAP > 38 mm Hg | 16 | 1 | 1 | 7 |
Lanzarini et al. (2005) | 86 | 57.0 | TTE | 91 | 69.8 | NA | |||||
Tramarin et al. (1991) | 98 | 38.1 | TTE | 98 | 30 | TVPG | sPAP > 35 mm Hg | 20 | 2 | 3 | 5 |
Liu et al. (1991) | 18 | 88.9 | TTE | 100 | 83.3 | TVPG > 30 mm Hg | mPAP > 25 mm Hg | 14 | 0 | 1 | 0 |
Eysmann et al. (1989) | 26 | 100 | TTE | 100 | 42.3 | NA | |||||
Torbicki et al. (1989) | 74 | 44.3 | TTE | 97 | 24 | TVPG > 32 mm Hg | sPAP > 35 mm Hg | 10 | 1 | 2 | 4 |
von Bibra et al. (1987) | 70 | 44.3 | TTE | 100 | 41.4 | NA |
Results from meta-regression are shown in Table 3 . Higher PH prevalence increased the sensitivity for PAAT ( P = .019), but pulmonary arterial hypertension percentage did not. A larger cutoff value increased the sensitivity of PAAT ( P = .044). There were five studies using 100 msec as a cutoff value for PH detection, and the adjusted sensitivity and specificity were 0.84 and 0.90, respectively. Arrhythmia in the population did not affect the specificity of PAAT’s diagnostic performance but increased the sensitivity of PH detection (adjusted sensitivity, 0.94; P < .001). In comparison with TEE, analyzing PAAT using TTE had higher sensitivity for PH detection (adjusted sensitivity, 0.88; P = .011). The RVOT was the most commonly chosen site for PAAT measurement, but measuring PAAT in the RVOT, main pulmonary artery, or right pulmonary artery did not result in statistically different sensitivity or specificity.
Variable | No. of studies | Sensitivity | Specificity | ||
---|---|---|---|---|---|
Adjusted | P | Adjusted | P | ||
Male, % | 16 | .706 | .217 | ||
≤60 | 11 | 0.87 | .770 | 0.86 | .215 |
>60 | 5 | 0.84 | 0.76 | ||
PH, % | 19 | .019 | .099 | ||
≤60 | 13 | 0.80 | .112 | 0.85 | .404 |
>60 | 6 | 0.91 | 0.81 | ||
PAH, % | 19 | .358 | .375 | ||
≤30 | 16 | 0.86 | .289 | 0.83 | .406 |
>30 | 3 | 0.73 | 0.89 | ||
Cutoff value | 19 | .044 | .809 | ||
<100 | 7 | 0.71 | 0.83 | ||
100 | 5 | 0.84 | .183 | 0.90 | .423 |
>100 | 7 | 0.92 | .004 | 0.83 | .990 |
Arrhythmia, % | 19 | <.001 | .351 | ||
0 | 7 | 0.74 | <.001 | 0.85 | .489 |
≥1 | 12 | 0.94 | 0.81 | ||
TEE/TTE | |||||
TEE | 3 | 0.55 | .011 | 0.91 | .072 |
TTE | 12 | 0.88 | 0.82 | ||
PAAT measurement site | |||||
MPA | 2 | 0.56 | 1.00 | ||
RPA | 1 | 0.57 | .954 | 0.79 | .984 |
RVOT | 16 | 0.87 | .056 | 0.83 | .984 |
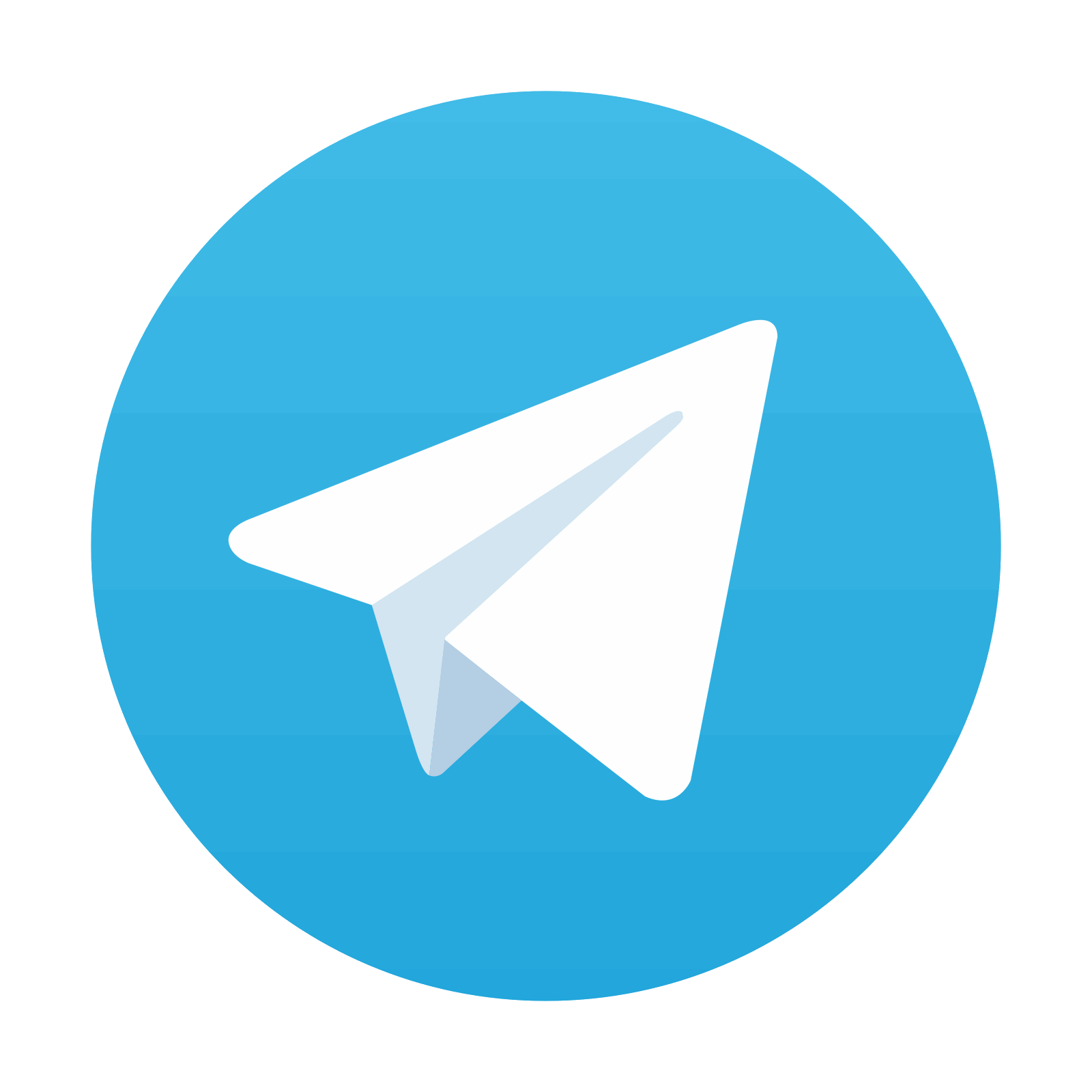
Stay updated, free articles. Join our Telegram channel

Full access? Get Clinical Tree
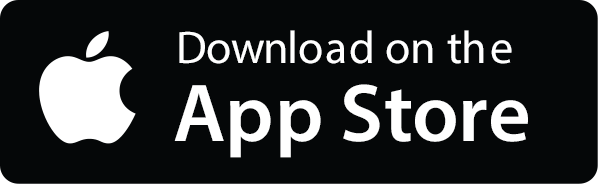
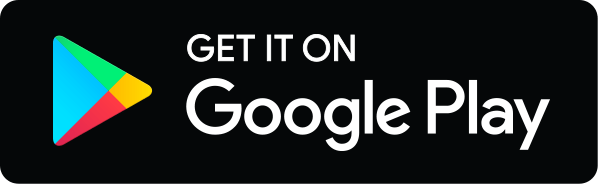
