Pulmonary Hypertension
Pulmonary hypertension describes a number of different diseases involving the pulmonary vasculature, which have common hemodynamic, histologic, and therapeutic features. The hallmark of these diseases is an abnormally high pulmonary vascular resistance. The histologic changes such as medial hypertrophy and intimal fibrosis do not vary significantly between different etiologies, whether the cause is idiopathic, hemodynamic, inflammatory, or toxin mediated. The hemodynamic and clinical consequences depend not only on the extent of vascular changes but also on the state of the subpulmonary ventricle, which is usually the morphologically right ventricle. Currently, pulmonary arterial hypertension is defined as a mean pulmonary arterial pressure of greater than 20 mm Hg at rest, or greater than 30 mm Hg while exercising, with a normal pulmonary capillary wedge pressure less than 15 mm Hg and a pulmonary vascular resistance index of more than 3 Wood units multiplied by total square meters of body surface area.
The different diseases resulting in increased pulmonary vascular resistance show a spectrum of responses to the currently available specific pulmonary antihypertensive substances, with disease-specific profiles of response beginning to emerge. With regard to the individual patient, nonetheless, a correct understanding of the precise pathophysiologic situation in which pulmonary vascular resistance is increased and causes pulmonary hypertension is of crucial importance for the correct commencement and evaluation of efficacy of treatment.
Classification of Pulmonary Hypertension
As early as 1891, Ernst von Romberg had described changes in the pulmonary vasculature in an autopsied patient with pulmonary hypertension, which he called sclerosis of the pulmonary arteries. Subsequently, the term primary pulmonary hypertension was coined in 1951. The original classification of chronic cor pulmonale was established by the World Health Organization in 1973 and has been revised numerous times. The most recent adult revision occurred in Nice, France, at the Fifth World Symposium on Pulmonary Arterial Hypertension with five groups ( Table 75.1 ). Because the associated diseases differ between children and adults with pulmonary hypertension, a pediatric-focused classification has also been proposed that has more emphasis on developmental and fetal origins of pulmonary hypertension ( Table 75.2 ). The clinically functional classification established by the New York Heart Association for cardiac disease ( Table 75.3 ) is used in an adapted form to assess the clinical severity and progression of the pulmonary vascular disease.
1 | Pulmonary arterial hypertension |
1.1 | Idiopathic |
1.2 | Heritable |
1.21 | BMPR2 |
1.22 | ALK-1, ENG, SMAD9, CAV1, KCNK3 |
1.23 | Unknown |
1.3 | Drug and toxin induced |
1.4 | Associated with: |
1.4.1 | Connective tissue disease |
1.4.2 | HIV infection |
1.4.3 | Portal hypertension |
1.4.4 | Congenital heart diseases |
1.4.5 | Schistosomiasis |
1′ | Pulmonary venoocclusive disease and/or pulmonary capillary hemangiomatosis |
1″ | Persistent pulmonary hypertension of the newborn |
2. | Pulmonary hypertension with left heart disease |
2.1. | Left ventricular systolic dysfunction |
2.2. | Left ventricular diastolic dysfunction |
2.3 | Valvar disease |
2.4 | Congenital/acquired left heart inflow/outflow tract obstruction and congenital cardiomyopathies |
3 | Pulmonary hypertension associated with lung diseases and/or hypoxemia |
3.1 | Chronic obstructive pulmonary disease |
3.2 | Interstitial lung disease |
3.3 | Other pulmonary disease with mixed restrictive and obstructive pattern |
3.4 | Sleep-disordered breathing |
3.5 | Alveolar hypoventilation disorders |
3.6 | Chronic exposure to high altitude |
3.7 | Developmental lung diseases |
4 | Chronic thromboembolic pulmonary hypertension |
5 | Pulmonary hypertension with unclear multifactorial mechanisms |
5.1 | Hematologic disorders: chronic hemolytic anemia, myeloproliferative disorders, splenectomy |
5.2 | Systemic disorders: sarcoidosis, pulmonary histiocytosis, lymphangioleiomyomatosis |
5.3 | Metabolic disorders: glycogen storage disease, Gaucher disease, thyroid disorders |
5.4 | Others: Tumoral |
1 | Prenatal or developmental pulmonary hypertensive vascular disease |
2 | Perinatal pulmonary vascular maladaptation |
3 | Pediatric cardiovascular disease |
4 | Bronchopulmonary dysplasia |
5 | Isolated pediatric pulmonary hypertensive vascular disease (isolated pediatric PAH) |
6 | Multifactorial pulmonary hypertensive vascular disease in congenital malformation syndromes |
7 | Pediatric lung disease |
8 | Pediatric thromboembolic disease |
9 | Pediatric hypobaric hypoxic exposure |
10 | Pediatric pulmonary vascular disease associated with other system disorders |
Class I | Patients with pulmonary hypertension, but without resulting limitation of physical activity. Ordinary physical activity does not cause undue dyspnea or fatigue, chest pain, or near syncope. |
Class II | Patients with pulmonary hypertension resulting in slight limitation of physical activity. They are comfortable at rest. Ordinary physical activity causes undue dyspnea or fatigue, chest pain, or near syncope. |
Class III | Patients with pulmonary hypertension resulting in marked limitation of physical activity. They are comfortable at rest. Less than ordinary activity causes undue dyspnea or fatigue, chest pain, or near syncope. |
Class IV | Patients with pulmonary hypertension with inability to carry out any physical activity without symptoms. These patients manifest signs of right heart failure. Dyspnea and/or fatigue may even be present at rest. Discomfort is increased by any physical activity. |
Associated Pediatric Pulmonary Hypertensive Diseases
Pulmonary Vascular Disease in Congenital Heart Disease
Congenital heart disease is one of the most common secondary causes of pulmonary vascular disease in children. Consequently, with the increasing number of children with congenital heart disease who survive to adulthood, the population of patients with pulmonary vascular disease due to congenital heart defects outnumbers those with idiopathic pulmonary hypertension. A patient with congenital heart disease can develop pulmonary hypertension by several different mechanisms due to a wide variety of congenital lesions. Pulmonary hypertension can occur in unrepaired heart disease, after appropriate complete repair, after incomplete repair or palliation, and acutely during the postoperative recovery after cardiopulmonary bypass.
Pulmonary Vascular Disease in Unrepaired Congenital Heart Disease
Unrepaired congenital heart disease can result in pulmonary vascular disease through several pathophysiologic mechanisms. These include excessive pulmonary blood flow (at normal or increased pressure), decreased pulmonary artery capacitance due to an underdeveloped pulmonary arterial bed, and postcapillary obstruction. The development of pulmonary vascular disease is also influenced by predisposing genetic syndromes such as Downs or Noonan syndrome.
Any congenital heart defect that is associated with excessive pulmonary blood flow can result in pulmonary hypertension. This includes the most common types of congenital heart disease, such as atrial septal defects, ventricular septal defects, and a patent ductus arteriosus, as well as rarer diseases such as common arterial trunk. An increase in the pulmonary blood flow can cause shear stress on the pulmonary arteries, altering the endothelin and nitric oxide pathways, predisposing to an elevated pulmonary vascular resistance. The timing of the development of pulmonary hypertension depends on the degree of excessive blood flow, the pressure of the shunted blood, and its oxygen content. Unrestrictive interarterial shunts will develop pulmonary vascular disease faster than ventricular or atrial shunts, due to exposure to both systolic and diastolic systemic arterial pressure. Ventricular shunts develop pulmonary vascular disease faster than do atrial shunts because they are exposed to systolic systemic pressure, whereas atrial level shunts are exposed only to increased flow and not increased pressure. Evaluating surgical candidacy in unrepaired congenital heart disease may require cardiac catheterization and pulmonary vasodilator testing ( Fig. 75.1 ).

Eisenmenger syndrome is seen when there is severe systemic cyanosis as a result of an unrepaired or incompletely repaired shunt (at the atrial, ventricular, or arterial level) that has right-to-left shunting due to persistent elevations in the pulmonary vascular resistance. As undiagnosed congenital heart disease becomes less common and there is improvement in earlier surgical repair, this syndrome is becoming less prevalent. The natural history of Eisenmenger syndrome is quite variable but eventually results in severe cyanosis and cardiac failure. In one study, half of a cohort of patients with unrepaired ventricular septal defect was alive 20 years after the diagnosis. Other symptoms include progressive shortness of breath, polycythemia, headache, and hemoptysis. Therapeutic options are limited and include supplemental oxygen therapy, anticoagulation, vasodilator therapy, and endothelin receptor antagonists. Bosentan and sildenafil have both been shown to increase functional capacity. Lung and heart-lung transplantation may be offered but have high 1- and 5-year mortalities. Oral contraceptives should be prescribed for females because pregnancy in such patients has a high incidence of maternal and perinatal death and so should be actively discouraged and avoided.
Pulmonary vascular disease can develop if, during fetal life, the pulmonary arteries are either very hypoplastic or absent. This occurs primarily with tetralogy of Fallot with pulmonary atresia. The severity of this disease is highly dependent on the presence and size of the native pulmonary arteries and the presence of major aortopulmonary collaterals. Small true pulmonary arteries have higher pulmonary vascular resistances and, if there are aortopulmonary collaterals, may be exposed to higher flow and pressure. The degree of pulmonary vascular disease will depend on the growth potential of the pulmonary arteries and establishing adequate pulmonary blood flow after surgical repair.
Finally, “downstream” obstruction can cause pulmonary artery hypertension. Obstruction may occur at the level of the pulmonary veins, left atrium (e.g., cor triatriatum), mitral valve, or atrial septum in functionally single ventricle lesions. These conditions all cause elevation in the pulmonary venous pressure that necessitates that the pulmonary arteries are exposed to higher pressures. Over time, this abnormal pressure and flow pattern can cause similar endothelial damage and intimal hypertrophy characteristic of pulmonary artery hypertension.
Pulmonary Artery Hypertension After Repair of Congenital Heart Disease
Pulmonary hypertension after repair or palliation of congenital heart disease has the same pathophysiology as described earlier. Pulmonary overcirculation can occur with residual ventricular level shunts or with any significant aortopulmonary shunts (i.e., Blalock-Taussig shunts, aortopulmonary collaterals). Another example is residual postcapillary obstruction after repair of total anomalous pulmonary venous return. Postoperative pulmonary vein stenosis carries a poor prognosis, and progression is difficult to predict. Extension of stenosis into segmental veins carries an especially poor prognosis. Currently, treatment regimens have included transcatheter interventions, chemotherapy agents, surgical repair, and lung transplantation. All treatment modalities have an unacceptably high mortality. Transcatheter interventions have been shown to be acutely successful but with extremely high reintervention rates and limited long-term benefit. Recently, repeated transcatheter interventions with systemic chemotherapy targeting myofibroblast-like cells has shown promise compared with historical controls.
Similarly, postcapillary obstruction as an etiology of long-term postoperative pulmonary vascular disease occurs in mitral valve repairs and repairs that are associated with elevated left ventricular end diastolic pressures. Multilevel left-sided obstruction (e.g., Shone complex) is particularly notable because the left-sided outflow tract obstruction, including subaortic stenosis and coarctation, predispose to an elevated left ventricular end-diastolic pressure, and pulmonary venous hypertension. Postcapillary obstruction is also a cause of pulmonary vascular disease in those with an atrial switch operation, usually due to pulmonary venous baffle obstruction.
Single ventricle palliation provides a unique challenge when it comes to the development of pulmonary vascular disease. Due to passive pulmonary blood flow present in the Fontan circulation, pulmonary vascular resistance often becomes the limiting factor to total cardiac output. Because there is no subpulmonary ventricle to provide a pump, even a relatively mild elevation in the pulmonary vascular disease limits the ability of blood to flow through the lungs. This can be further exacerbated by the limited growth potential of the pulmonary arteries. The staged palliation currently in practice limits the volume load to the ventricle at the expense of pulmonary blood flow beginning with the Glenn operation (superior cavopulmonary anastomosis). This change in pulmonary blood flow and the lack of pulsatility of flow in a cavopulmonary anastomosis may limit the growth potential of the pulmonary arteries and thus increases pulmonary vascular resistance. Because pulmonary vascular resistance is the bottleneck of cardiac output in patients palliated with a Fontan circulation, targeted modulation of the pulmonary vascular systems has been proposed as a treatment for some complications of Fontan physiology. Studies using phosphodiesterase inhibitors and endothelin antagonists have shown variable effects on exercise capacity and symptoms such as protein-losing enteropathy and plastic bronchitis, and their role remains to be determined. Further investigations into pulmonary vascular–directed therapy are currently underway in this population.
Acute Postoperative Pulmonary Hypertension
Acute postoperative pulmonary hypertension is a transient clinical and pathophysiologic condition that is a consequence of cardiopulmonary bypass and is an important contributor to postoperative morbidity. Although the pathophysiology is incompletely understood, cardiopulmonary bypass leads to transient endothelial injury and disruption of nitric oxide production. As a result, there is a transient elevation of pulmonary vascular resistance and increased sensitivity to vasoconstrictive stimuli. The resistance can be lowered by supplementation of elements from the l-arginine–nitric oxide pathway and by blockade of the endothelin receptors, suggesting a combined pathophysiology of different pathways as a cause. This pathophysiology may persist for several days, in extreme cases making the lung very vulnerable to different stimuli such as handling, suctioning, movement, and hypoventilation. Such stimuli can be associated with life-threatening pulmonary vasospasm. This is the so-called pulmonary hypertensive crisis, which results in a sharp increase in pulmonary vascular resistance and pulmonary arterial pressure, acute right ventricular decompensation with raised central venous pressure, decreased pulmonary blood flow with cyanosis, and left atrial and systemic hypotension ( Fig. 75.2A ). In infants and young patients, this phase is directly associated with a decrease in lung compliance (see Fig. 75.2B ), hypoventilation, hypercarbia, and further pulmonary vascular restriction, producing a vicious circle that can only be interrupted by manual forced and energetic hyperventilation and that may require other immediate measures aimed at resuscitation ( Fig. 75.3 ). This bronchopulmonary interaction has also been described elsewhere, such as in the variation of both pulmonary compliance and flow of blood to the lungs and asthma in pulmonary hypertension.


The incidence of life-threatening postcardiopulmonary bypass pulmonary hypertensive crises has markedly decreased, from 20% in the 1980s to only 2% to 5% 25 years later. This change reflects improved surgical techniques, treatments to lessen the impact of cardiopulmonary bypass, and, most importantly, earlier age at operation before the pulmonary vasculature has been injured. However, the pathophysiology nevertheless remains relevant for complex patients, particularly for those with the Fontan circulation. Risk factors for pulmonary hypertensive crisis include patient age, type of cardiac lesion, and presence of other genetic syndromes such as trisomy 21. Basic strategies for preventing pulmonary hypertensive crisis include avoidance of triggering stimuli such as acidosis and hypercarbia. Inhaled pulmonary vasodilators including nitric oxide and iloprost have been shown to reduce pulmonary artery pressures postoperatively and, in the case of nitric oxide, shorten length of stay. Other commonly used pulmonary vasodilators include sildenafil, milrinone, and levosimendan. Advanced strategies such as extracorporeal membrane oxygenation are indicated only for bridging a situation that may be expected to recover.
Pulmonary Hypertension Associated With Bronchopulmonary Dysplasia or Chronic Lung Disease
Patients with pulmonary hypertension associated with prematurity and bronchopulmonary dysplasia (BPD) represents another significant population with pulmonary hypertension in childhood. Infants born at less than 28 weeks’ gestation seem to have the highest risk. Other risk factors include low gestational age, small for gestational age, oligohydramnios, duration of oxygen therapy, and duration of mechanical ventilation. Early pulmonary hypertension in premature infants has also been shown as a risk factor for severe BPD and mortality. The incidence of pulmonary hypertension is loosely associated with the severity of BPD, with an estimated incidence of 4% in mild BPD and up to 33% in severe BPD. Recent estimates suggest that between 25% and 40% of neonates with BPD have some evidence of pulmonary hypertension. Histologic findings suggest an arrested alveolar development, or loss of alveoli, and a disruption in angiogenesis causing increased vascular tone and altered vasoreactivity. In contrast, preservation of vascular growth and endothelial survival may promote growth and may sustain the architecture of the distal air space.
Echocardiography is the most frequently used screening technique for pulmonary hypertension associated with BPD. Current guidelines recommend screening when an infant is diagnosed with moderate or severe BPD or for patients with worsening pulmonary status. Cardiac catheterization is reserved for those with severe pulmonary hypertension, primarily to evaluate for comorbidities and assess pulmonary vasoreactivity. An evaluation for concomitant conditions should be undertaken just as it is required in older patients. Evaluation should assess for the presence of pulmonary vein stenosis, atrial communications, left ventricle diastolic dysfunction, and aortopulmonary collaterals. The mainstay of treatment for BPD associated pulmonary hypertension includes oxygen to avoid hypoxemic vasoconstriction. Recently, other pulmonary vasodilators have been used with limited clinical data in this population. Specifically, sildenafil has limited clinical studies showing benefit. Pulmonary vasodilators may be considered after careful evaluation and exclusion of fixed pulmonary vascular resistance.
Congenital Diaphragmatic Hernia
This entity is characterized by pulmonary hypoplasia, with structural as well as functional anomalies, leading to a high pulmonary vascular resistance and pulmonary hypertension. A combination of compression of the lungs during fetal life, limited flow of blood to the lungs, and intrinsic pulmonary developmental arrest is responsible for the parenchymal pulmonary hypoplasia. In addition, a dysregulation of the expression of the receptors for endothelin-1 has been documented, which has been shown to decrease local nitric oxide and vascular relaxation. Evidence of altered autonomic innervation has also been seen in human lung specimens, which may contribute to hyperreactivity of the pulmonary vasculature. All these factors lead to the elevated pulmonary vascular resistance characteristic of congenital diaphragmatic hernia. The degree of pulmonary vascular resistance is a strong prognostic indicator for survival. The clinical management includes surgical repair in the early neonatal phase, followed by supportive care over the long term. With newer options for treatment, such as high-frequency oscillatory ventilation, intravenous infusion of prostaglandin, inhaled nitric oxide, and extracorporeal membrane oxygenation, better neonatal survival is achieved, but the number of patients with chronic lung disease, or recurrent or residual pulmonary hypertension, is also increasing. There are no controlled clinical trials on the use of vasodilators in long-term survivors with elevated pulmonary arterial pressures. Maximal exercise capacity was shown to be mildly decreased when a cohort of patients with this problem was compared with normal controls.
Pulmonary Venoocclusive Disease
This is a very rare condition that affects mainly, but not exclusively, children and young adults. Multiple possible factors for its development have been proposed, including infections, genetic changes, toxic exposure, and autoimmune disorders. The clinical presentation is very similar to idiopathic pulmonary arterial hypertension, with shortness of breath, chest pain, syncope, hypoxemia, and later signs of right-sided cardiac failure. Histologic findings include intimal fibrosis of the pulmonary veins, leading to narrowing and occlusion of affected vessels. From the hemodynamic aspect, there is elevated pulmonary arterial pressure, with a raised pulmonary capillary wedge pressure, but normal left atrial pressure. The chest radiograph typically shows enlargement of the pulmonary trunk, pulmonary edema with prominent Kerley B lines, and pleural effusions. On computed tomography (CT), the disease manifests with thickened interlobular septa, enlarged lymph nodes, and ground-glass opacities. Definitive diagnosis requires lung biopsy, a high-risk procedure in end-stage patients. Cardiac catheterization, with testing for vasodilation, may be indicated, but acute pulmonary edema has been described. Therapy includes supplemental oxygen and diuresis, as well as anticoagulation. There are also reports on the use of immunosuppressive therapy with corticosteroids and azathioprine, but this approach should be limited to patients with suspected or proven collagen vascular disease. Lung transplantation is most likely the only option for treatment, but the long waiting time for organs may limit the final outcome.
Alveolar Capillary Dysplasia
This is a relatively rare cause of persistent pulmonary hypertension of the newborn, but its incidence is most likely underestimated. A retrospective study on neonates treated with extracorporeal membrane oxygenation in the United Kingdom between 1997 and 2000 identified a total of nine patients with such irreversible lung dysplasia. The etiology is still uncertain, with familial cases suggesting a possible genetic mutation. The histologic findings include muscularization of the pulmonary arterioles, a deficiency in the number of alveolar units and pulmonary capillary vessels with thickening of the interalveolar septa, anomalous pulmonary veins accompanying the pulmonary arteries and bronchi, and dilated lymphatics. The newborn usually presents with severe hypoxemia, without response to treatment with oxygen or pulmonary vasodilators. Open lung biopsy has been recommended if a neonate with persistent pulmonary hypertension shows no clinical improvement after 72 hours despite maximal medical therapy. Cardiac catheterization may be helpful to complete the diagnostic work-up. Currently the disease is incurable, with a maximum reported survival time of approximately 3 months, albeit sometimes with a phase of mild improvement with the administration of vasodilating agents.
Pulmonary Hypertension Associated With Pulmonary Venous Obstruction
Pulmonary venous obstruction induces both pulmonary arterial and pulmonary venous hypertension. The condition may be related to anatomic abnormalities of the pulmonary veins and congenital heart disease, as described earlier, or less frequently may be congenital. Pulmonary vein stenosis has also been seen in cases of BPD believed to be due to the disruption of angiogenesis that is characteristic of BPD. Although rare, this is likely an underdiagnosed phenomenon.
Diagnosis of Pulmonary Hypertension
Diagnostic Approach
The diagnosis of pulmonary hypertension can be elusive because early signs and symptoms are often subtle and nonspecific. Once pulmonary hypertension has been diagnosed, testing has several purposes. Patients require assessment of ventricular function, disease severity, and functional capacity. A complete work-up for secondary causes of pulmonary hypertension should also be performed.
Presenting Symptoms
Despite multiple associated diseases, patients with pulmonary hypertension develop similar symptoms. In the early stages, pulmonary hypertension is asymptomatic until cardiac function becomes affected. Progressive dyspnea with activity that progresses over months is the most frequent symptom. Chest pain and syncope with exertion may become evident with more severe pulmonary hypertension and impaired right ventricular function. Peripheral edema and hepatomegaly develop in later stages. Syncope is a concerning symptom and has been associated with mortality risk independent of the degree of pulmonary artery pressure elevation or right ventricular dysfunction. Patients with pulmonary hypertension associated with left heart disease or pulmonary venous obstruction may present with pulmonary edema. Grading the limitations in exercise using the World Health Organization classification is useful for monitoring patient’s response to therapy (see Table 75.3 ).
Physical Findings
Depending on the degree of pulmonary artery pressure elevation, a patient may exhibit few if any physical signs. A loud second heart sound is present in most patients with elevated pulmonary artery pressures. Murmurs due to tricuspid regurgitation or pulmonary insufficiency may also be heard. In later stages, signs of right heart failure such as jugular venous distension, hepatomegaly, peripheral edema, or ascites become evident.
Chest Radiography
Typically, the central pulmonary arteries are enlarged, and there may be cardiomegaly. In addition, there are diminished peripheral pulmonary vascular markings. The presence of increased interstitial markings or pulmonary edema should prompt a work-up for interstitial lung disease, pulmonary venous obstruction, or left heart disease as an etiology of pulmonary hypertension.
Electrocardiogram
The electrocardiogram may be normal in a minority of patients. In most patients the electrocardiogram demonstrates right axis deviation, right atrial enlargement, and/or right ventricular hypertrophy, but the sensitivity and specificity are low.
Biomarkers
The most commonly used biomarker during evaluation of pulmonary hypertension is b-type naturetic protein (BNP) or NT-pro BNP. These are released by the right and left atrium in response to volume overload and stretch. They are not specific but have been shown to correlate with prognosis. In isolation, BNP values are likely not useful but may be a useful marker to trend.
Six-Minute Walk Test and Exercise Testing
The 6-minute walk test is a submaximal exercise test and has been useful in adults with more severe pulmonary hypertension because pretreatment results correlate with survival. The test is useful for longitudinal follow-up and to monitor response to treatment, although improvements in walk distance do not correlate with survival. The test is easy to perform and has been used as an end point in prospective clinical trials. The limitations of the test include limited utility in patients with less severe disease, subjective nature, and that patient motivation and cooperation can greatly affect results. In patients with less severe disease, serial cardiopulmonary exercise testing is useful.
Echocardiography
Echocardiography is one of the most important tools for screening and diagnosing pulmonary hypertension. Signs of pulmonary hypertension include right atrial and ventricular enlargement, possibly with decreased right ventricular function ( and ). Doppler echocardiography allows estimating the right ventricular systolic pressure by assessing the tricuspid regurgitant velocity. However, many patients do not have detectable tricuspid valve regurgitation or pulmonary insufficiency, and thus echocardiography may miss significant pulmonary hypertension. Other indirect signs of pulmonary hypertension are a flattened or paradoxical ventricular septum during systole (the eccentricity index is a surprisingly accurate surrogate of right ventricular pressure elevation), inferior vena cava dilation, and collapse. Echocardiography is very important to rule out structural heart disease as the etiology of pulmonary or right ventricular pressure elevation. An assessment of right ventricular function should also be obtained. Tissue Doppler imaging is able to demonstrate abnormal diastolic and systolic right ventricular function, as well as left ventricular diastolic function. Recent advancements in echocardiography have increased the available tools to measure right ventricular performance. Those with highest diagnostic accuracy include speckle tracking global longitudinal strain and right ventricular free wall strain. Tricuspid annular plane systolic excursion has also been investigated with less diagnostic accuracy.
Magnetic Resonance Imaging
Cardiac magnetic resonance imaging (MRI) is the gold standard for evaluation of right ventricular performance. Phase-contrast magnetic resonance measurements of flow in the pulmonary trunk correlate with hemodynamic changes, and the average velocity throughout the cardiac circle appears to mirror the pulmonary pressures and resistance. In patients clinically known to have pulmonary hypertension, the cardiac MRI ratio–derived left ventricular septal-to-free wall curvature was an accurate and reproducible index for estimation of right ventricular systolic pressure if compared with measurement at right heart catheterization. Several MRI-derived parameters including volume, stroke volumes, and right ventricular ejection fractions have been associated with mortality in pulmonary hypertension in adults. These findings need to be validated in the pediatric population, but cardiac MRI is an important monitoring tool of right ventricular function.
Cardiac Catheterization
Catheterization of the right heart remains the gold standard for diagnosis of pulmonary hypertension, but the procedure for patients with advanced pulmonary vascular disease is associated with significant mortality and morbidity risks. Catheterization in patients with severe pulmonary hypertension should be performed only in centers experienced with the management of pediatric pulmonary hypertension. The goal of initial catheterization is to confirm the diagnosis, assess the severity of disease, assess responsiveness to vasodilators, and exclude other potential treatable diagnoses. Subsequent catheterizations are useful to monitor disease progression, response to therapies, and assess candidacy for lung or heart-lung transplantation. A standard right heart catheterization should be performed with careful assessment of right atrial pressure, pulmonary arterial pressures, pulmonary capillary wedge pressure, and systemic blood pressure. If the pulmonary capillary wedge pressure is unusually high or low, direct measurement of left ventricular pressures are important in assessing for pulmonary venous obstruction. Measurement of systemic and pulmonary blood flows should be performed.
Acute vasoreactivity testing is an important component of catheterization to assess candidacy for use of calcium channel blocking medications and for prognosis. The definition of a responder in adults with isolated pulmonary hypertension include a fall in pulmonary artery pressure to less than 40 mm Hg and either preserved or increased cardiac output. An alternative definition is a fall in mean pulmonary artery pressure by 20% with preserved or increased cardiac index and preserved or decreased pulmonary vascular resistance/systemic vascular resistance ratio. Agents used for testing include inhaled nitric oxide, intravenous adenosine or sildenafil, or inhaled prostacyclins. The number of children who are responsive to vasodilators varies in the literature, with a range of 10% to 30%.
Lung Biopsy
This procedure is rarely indicated in the modern era. However, biopsy may be essential in diagnosing vasculitis, pulmonary venoocclusive disease, or pulmonary capillary hemangiomatosis. The most important limitation of an open lung biopsy is the high risk of mortality during anesthesia and the surgical procedure.
Genetic Testing
Mutations in several genes primarily from the transforming growth factor-β superfamily of receptors have been identified as a cause of pulmonary hypertension. The most commonly affected gene identified in patients is bone morphogenetic protein receptor 2 (BMPR2). BMPR2 mutations have been identified in approximately half of patients with familial pulmonary hypertension. Pulmonary hypertension may also be found in association with hereditary hemorrhagic telangiectasia, which can be caused by mutations in either ACVRL1, ENG, or ALK1 genes. Recently, a mutation in TBX4 has been identified in children and only rarely in adults.
Although genetic testing is not routinely used to guide patient management, pediatric pulmonary hypertension patients with BMPR2 mutations are less likely to respond to acute vasodilator testing than mutation-negative patients, and they also seem to have a more severe disease at diagnosis. According to current guidelines, genetic testing and professional counseling should be offered to the patients with idiopathic and familial disease and to the relatives of those patients with familial disease. Genetic counseling is performed to help families understand and cope with the complexities of testing and its psychosocial impact. For example, BMPR2 has incomplete penetrance and not everyone with a mutation develops pulmonary hypertension. This is suggestive that a second hit, either genetic or environmental, is necessary for the development of pulmonary hypertension. Genetic testing is most helpful identifying family members who are not genetically at risk and who can therefore forgo serial screening for pulmonary hypertension.
Diagnosing Secondary Causes of Pulmonary Hypertension
At the time of initial pulmonary hypertension diagnosis, patients require not only a formal evaluation of cardiac function but also testing for pulmonary hypertension etiology or classification. The amount and type of testing is dependent on the patient’s age and initial findings. As stated previously, a complete echocardiogram and cardiac catheterization should be obtained on all patients diagnosed with pulmonary hypertension. Etiologic laboratory testing includes studies for HIV infection, hypercoagulability, liver disease, hemoglobinopathies, and connective tissue disease.
Ventilation-perfusion scintigraphy has high sensitivity and specificity to detect embolic disease, particularly in adults and adolescence. Scintigraphy can reliably differentiate between large-vessel occlusive disease and small-vessel pulmonary vascular disease. In smaller children, chest CT angiogram may be better tolerated and can be used to detect pulmonary embolism. Chest CT with and without contrast should be considered to detect signs of interstitial lung disease, pulmonary venoocclusive disease, and pulmonary embolus. Findings on CT scan indicative of pulmonary hypertension include cardiomegaly, right atrial and ventricular enlargement, and dilation of the proximal pulmonary arteries.
In patients with risk factors for sleep-disordered breathing, a sleep study should be obtained. It is also recommended to obtain a sleep study in patients who have poor response to initiation of pulmonary hypertension medications.
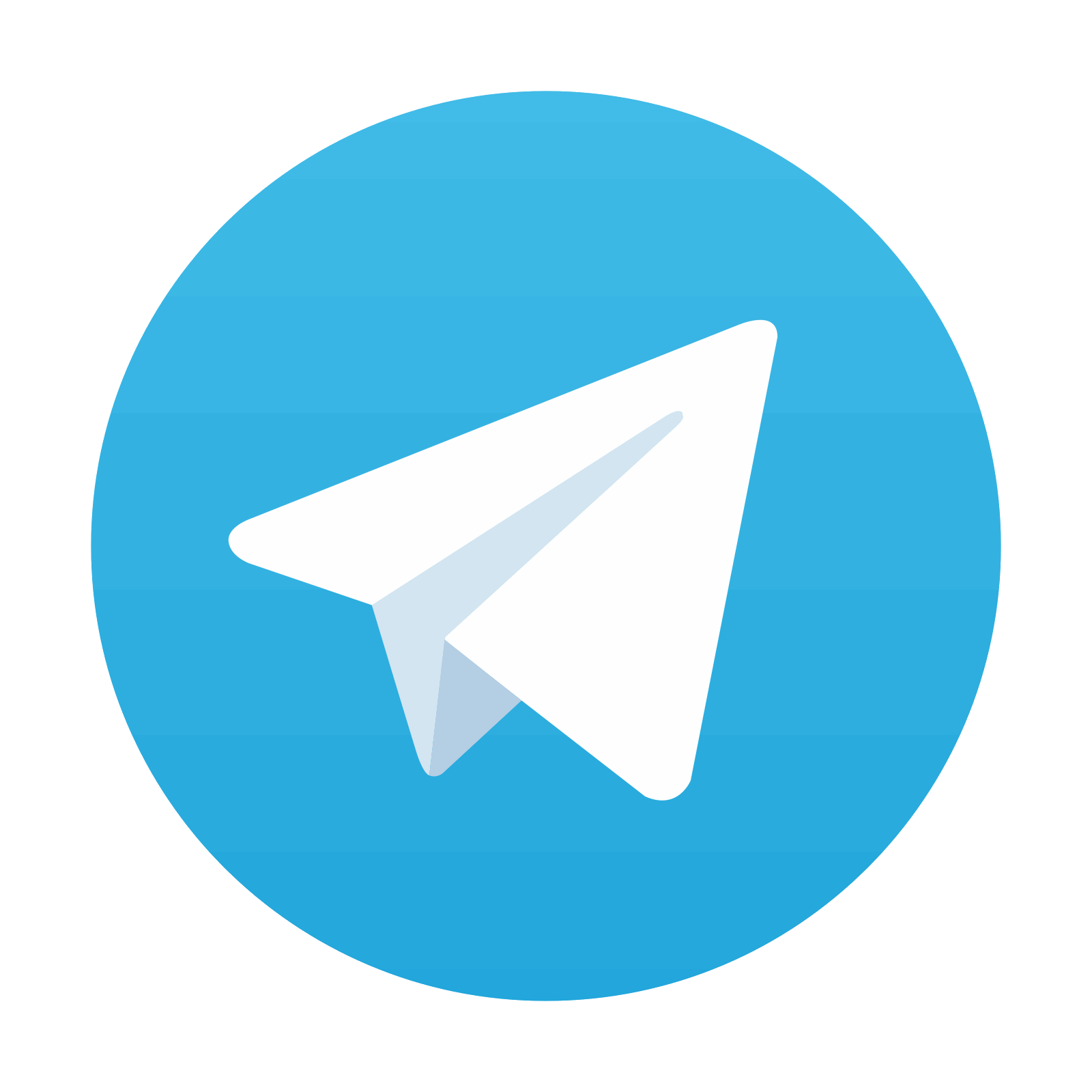
Stay updated, free articles. Join our Telegram channel

Full access? Get Clinical Tree
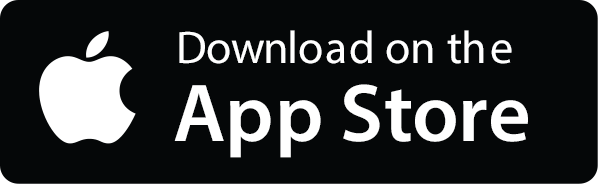
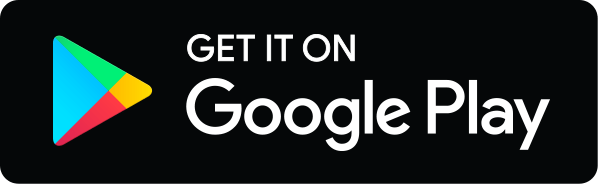