Abstract
Pulmonary hypertension is a formidable challenge to the care of children with congenital heart disease, with a burgeoning incidence in the perioperative period. Awareness of this problem, prospective clinical pathway development, and focused expertise are all needed to optimally equip multidisciplinary teams to meet this challenge. Here we present insights into epidemiology, costs, anatomy, pathophysiology, medical diagnostics, and pharmacotherapies involved in treating pulmonary hypertension in pediatric congenital heart disease patients. The emphasis is on identifying patients at risk, choosing appropriate diagnostics, optimizing perioperative outcomes, timely recognition and management of pulmonary hypertensive crisis, the molecular basis of pharmacotherapy, and guidelines for treatment. Anticipatory guidance is also provided for transferring children from hospital to home, and frontiers of research in the field are considered.
Key Words
pulmonary hypertension, child, pulmonary vasodilator, inhaled vasodilator, pulmonary vascular disease, pulmonary vascular resistance, perioperative risk, nitric oxide, prostacyclin, endothelin receptor antagonist, phosphodiesterase inhibitor, right heart failure, congenital heart disease
Pulmonary Hypertension in Congenital Heart Disease
Epidemiology, Physiology, and Anatomy of Pulmonary Vascular Disease
Abnormally high pulmonary vascular tone has been known to be an adversary to optimal cardiac function in the perioperative period in premier pediatric cardiac surgical programs for decades. This challenge has seemingly intensified recently, as the recognition and targeted care of pulmonary hypertension (PH) have skyrocketed in pediatric inpatients across the board—including those with congenital heart disease (CHD). This dynamic upswing in PH care in the United States is attested to by an increase in inflation-adjusted national inpatient hospital charges for care of this problem from $926 million to $3.12 billion between 1997 and 2012.
Pulmonary arterial hypertension (PAH) is a clinical condition of precapillary PH defined in adults by a mean pulmonary artery pressure (mPAP) of 25 mm Hg or higher. PAP can be elevated either from increased pulmonary vascular resistance (PVR) or from increased blood flow. According to Ohm’s law the voltage difference (i.e., pressure difference) is equal to the current (i.e., blood flow) multiplied by the resistance to flow (i.e., PVR).
Pressure = ( Flow ) × ( Resistance )
Although many children with PH in the setting of CHD have acquired this problem because of elevated PVR, two additional clinical scenarios are worth mentioning, and both may be understood based upon the relationships described by the previous equation. First, elevated PVR may exist when mPAP is normal (<25 mm Hg) if pulmonary blood flow is also low—such as in patients without a pulmonary ventricle who have undergone cavopulmonary anastomosis (bidirectional Glenn shunt). Second, some children may have increased pulmonary blood flow (i.e., intraventricular shunt with left-to-right flow) resulting in an mPAP of 25 mm Hg or higher but not have increased PVR from pulmonary vascular disease (PVD).
Deleterious histologic changes are often seen in pediatric patients with PH. Patients with PH may have a deficiency of vasodilator pathways as evidenced by less endogenous prostacyclin, reduced expression of nitrogen oxide synthase and vasoactive intestinal peptide in the lungs, and increased levels of circulating endothelin-1and serotonin. These conditions may ultimately result in migration of smooth muscle cells into peripheral arteries that are normally nonmuscularized, to medial hypertrophy in the muscular arteries, impaired microvascular growth, progressive loss of arterioles, and a decrease in arterial density. There is general consensus that the early changes in this evolution of pathophysiology may be remodeled toward normal microvascular infrastructure over time but that the later and more advanced vascular wall changes may become irreversible.
Challenges Specific to Congenital Heart Disease
PH may substantially contribute to morbidity and mortality in infants and children with CHD. Pathogenesis of PH in many children with CHD may be linked to three types of aberrations of pulmonary circulatory dynamics: excessive pulmonary blood flow, fixed obstruction of the right ventricular (RV) outflow tract or the main or branch pulmonary arteries, or obstruction of pulmonary venous return ( Table 71.1 ). Conditions involving either intracardiac (i.e., ventricular septal defect [VSD], complete atrioventricular [AV] canal) or extracardiac (i.e., aortopulmonary window, patent ductus arteriosus [PDA], truncus arteriosus) communication between the systemic and pulmonary circulation can result in increased blood flow in the developing pulmonary circulation. The expected response to this increase in pulmonary blood flow is a decrease in the vascular resistance, to maintain normal pulmonary pressure. In this situation it is considered abnormal when PVR remains normal or is increased. Thus when PVR does increase, this suggests that progressive pulmonary vascular remodeling has occurred with increased vascular smooth muscle. When PVR fails to appropriately decrease, it may be termed hyperkinetic pulmonary hypertension. This is one reason that children with CHD should undergo repair early in life to reduce the occurrence of PVD.
Pathogenesis of RV Hypertension | Cardiac Lesion(s) | Perioperative Management Priorities |
---|---|---|
Excessive pulmonary blood flow | VSD ASD PDA Truncus arteriosus Aortopulmonary window TGA |
|
Fixed right ventricular outflow tract or pulmonary artery obstruction | TOF with or without hypoplastic PAs |
|
Obstructed pulmonary venous return | Pulmonary vein obstruction with or without TAPVR Mitral stenosis/atresia LV diastolic dysfunction |
|
In the fetus, PVR is very high, and pulmonary blood flow is approximately 30 to 40 mL/kg/min, or approximately 8% of combined ventricular output. With the initiation of breathing at birth, there is an initial rapid decrease in PVR, allowing pulmonary blood flow to increase to approximately 350 to 400 mL/kg/min. This is followed by a slower, progressive decline in PVR (and therefore pulmonary pressure) over the next 6 to 8 weeks after birth. However, in some newborns high PVR persists after birth, and the expected increase in pulmonary blood flow is never observed. If no underlying congenital heart lesion is present, this is referred to as persistent pulmonary hypertension of the newborn (PPHN). Elevated PVR may cause right-to-left shunting of blood and subsequent hypoxemia in the presence of a persistent foramen ovale and/or PDA.
In contrast to PPHN the development PVD in the presence of CHD is a usually a consequence of the underlying cardiac lesion, rather than the primary disorder. The evolution of PVD in CHD depends not only on the location and size of the shunt but also on the hemodynamic burden it imposes on the pulmonary vasculature. Cardiac lesions that shunt left to right and result in hyperkinetic pulmonary circulation include septal defects, aortopulmonary connection, and PDA. When small, these lesions pose minimal risk for the development of PVD. Some patients do not develop symptoms of congestive heart failure or pulmonary vascular congestion even when the defect is large, but this should not prevent a child with a large, unrestrictive defect from undergoing percutaneous or surgical closure early in life to subvert the development of PH. In the first 2 years of life, closure of a VSD (even in the presence of markedly elevated PVR) may result in normal or near-normal PVR following repair (despite a 50% risk of preoperative PH at 2 years of age), with the likelihood of favorable pulmonary vascular remodeling being even better if the operation is performed in the first year of life. A PDA may also cause PVD, but the occurrence of irreversible PVD seldom occurs before 2 years of age. On the other hand, older children who undergo surgical repair may not have a substantial drop in PVR postoperatively. Thus the approach to the older patient with PH and CHD involves careful evaluation and perioperative planning. Factors that raise concern for elevated PVR include late presentation, lack of pulmonary congestion, bidirectional shunting, systemic oxygen desaturation, and presence of underlying genetic syndromes. (Down syndrome, DiGeorge syndrome, and Noonan syndrome are some more common examples.)
In contrast, prolonged exposure to increased pulmonary blood flow from an atrial septal defect (ASD) causes PVD in children less commonly, although large ASDs may lead to moderate or severe PH in up to 59% of patients by adulthood and may shorten the life expectancy by 50% if repaired beyond the fourth decade of life.
If PH occurs in the presence of a small atrial-level shunt, investigation for a possible underlying genetic predisposition to developing PVD is needed. Genetics consultation has a growing and vital role in the management of PH in CHD. For example, transforming growth factor beta receptors have a pivotal role in cardiac embryogenesis and vascular development; therefore gene mutations in these receptors, especially BMPR2 (bone morphogenetic protein receptor 2) and in ALK-1 (activin A receptor-like kinase type 1—present in hereditary hemorrhagic telangiectasia), are of critical interest.
Infants with transposition of the great arteries (TGA) with VSD who are not repaired within the early neonatal period may develop severe PVD during the first year of life. This is likely is due to in utero preconditioning of the pulmonary vasculature. Pulmonary arteries normally carry low oxygen content from the right ventricle, whereas the oxygen-rich blood from the placenta is preferentially shunted from the inferior vena cava across the foramen ovale to the left heart by the eustachian valve and then is ejected out the left ventricle into the aorta. In d-TGA, oxygen-rich blood from the placenta is instead delivered to the pulmonary arteries, and the pulmonary vessels dilate in utero, leading to stretch injury of the vascular intima with secondary vasoconstriction. This may explain why some children with d-TGA (with or without a VSD) may develop PVD despite a successful early neonatal arterial switch operation.
Truncus arteriosus, aortic origin of a pulmonary artery, complete AV canal defects, and double-outlet right ventricle with VSD may all be associated with rapid evolution of PVD. These lesions all result in excessive pulmonary-to-systemic flow ratios (Q p :Q s ) as PVR decreases after birth, and many of these infants are at risk of death in the first year without surgical correction. These lesions therefore warrant consideration for complete repair in early infancy.
In infants with unobstructed total anomalous pulmonary venous connection (TAPVC), the added pulmonary venous return to the right heart causes RV volume overload and increased pulmonary blood flow. This may induce muscularization of the pulmonary vascular bed and irreversible PH. Partial anomalous pulmonary venous connection with more than half of the pulmonary veins draining anomalously and an associated ASD can also cause right heart failure with PH, but this usually evolves later in life. If the anomalous veins are obstructed, elevation of pulmonary pressures occurs from pulmonary venous hypertension rather than from increased pulmonary blood flow.
Shunt-mediated PH is usually reversible if the surgical correction is performed early. In one study involving 280 autopsies in infants under 1 year of age with CHD, advanced degrees of pulmonary hypertensive arteriopathy (grade IV or more) occurred in only 6 infants. Rabinovitch et al. performed lung biopsy on 74 patients with congenital heart defects who had either PH or high-risk lesions, including VSD, d-TGA, and complete AV canal. One year after repair, mPAP and/or PVR were normal in all patients whose conditions were corrected surgically before 9 months of age, regardless of the severity of the pulmonary vascular changes on biopsy at the time of the operation. Furthermore, pulmonary arterial pressure and PVR were increased in all patients whose conditions were repaired after 2 years of age with grade C morphometric findings and severely elevated in children with Heath-Edwards grade III biopsy.
Elevated pulmonary arterial pressures may also occur secondary to postcapillary PH (i.e., secondary to left heart dysfunction and/or pulmonary venous obstruction). This occurs because the elevated left heart pressure (high left atrial pressure) is passively transmitted back toward the pulmonary veins and arteries, leading to reflex changes in pulmonary artery pressure. Hemodynamic measurements will initially show an elevated pulmonary capillary wedge pressure (PCWP) with normal PVR of less than 3 Wood units and transpulmonary gradient (mean PA pressure − PCWP) of approximately 12 mm Hg. In patients in whom pulmonary vascular remodeling has occurred, the PA pressure will increase to a much higher degree than the left atrial pressure (LAP), resulting in an elevated transpulmonary gradient above 12 mm Hg. In older children and adults, left heart lesions (i.e., mitral valve stenosis, aortic valve stenosis, cor triatriatum) and both systolic and diastolic ventricular dysfunction can cause PH. In newborns, postcapillary PH is more commonly due to obstructed TAPVC, in which elevated LAP is absent because the obstruction is proximal to the left atrium. The pulmonary venous obstruction can quickly lead to subsequent pulmonary vascular changes. Pulmonary artery vasoconstriction and alveolar capillary remodeling occur as a compensatory mechanism protecting against the development of pulmonary edema that occurs from transudation of fluid into the alveolar space in the setting of downstream obstruction. Prolonged, severe pulmonary venous hypertension promotes hypertrophy of the pulmonary arterioles and arterialization of the pulmonary venules. Autopsies of infants with obstructed TAPVC demonstrated increased arterial medial thickness, intimal proliferation in periacinar veins, and abnormally small and thick-walled extrapulmonary veins. It is therefore no surprise that neonates with obstructed TAPVC are extraordinarily vulnerable to PAH in the perioperative period despite a successful repair.
Perioperative Risk in Patients With PH
Children with PH have increased perioperative risk, and those with underlying congenital cardiac disease are more likely to have cardiac arrest. Preoperative planning and risk assessment are guided by a child’s preoperative functional status, current medical therapies, coexisting medical conditions, the type of procedure (e.g., emergency versus elective, open versus laparoscopic, intrathoracic versus intraabdominal), the planned anesthetic approach (sedation versus general anesthetic, use of a neuroaxial or regional nerve block), and postoperative disposition (need for postoperative mechanical ventilation).
Baseline perioperative risk assessment encompasses clinical symptoms, biomarker data, echocardiographic findings, and hemodynamic parameters from cardiac catheterization ( Fig. 71.1 ). The most substantial risk factors for adverse perioperative events include suprasystemic PA pressures, decreased RV function, young age, home use of supplemental oxygen, new PH diagnosis before therapy, and lack of a stable therapeutic regimen. Children with idiopathic or heritable PH may also be at higher perioperative risk. Finally, patients with chronic or long-standing PH may develop RV hypertrophy and be at risk for RV ischemia due to increased resistance to coronary flow and lowered systemic vascular resistance (SVR) with general anesthesia.

One single-center retrospective study of 156 children undergoing noncardiac surgical procedures and cardiac catheterizations from 1999 to 2004 found that suprasystemic PA pressure was a predictor of major perioperative complications (odds ratio [OR], 9.1; P = .02), including cardiac arrest (with overall prevalence of 1.17%) and pulmonary hypertensive crisis. Another study of 97 cardiac catheterization procedures in children with known PH, between 2009 and 2014, showed 3% incidence of systemic hypotension and zero incidence of cardiac arrest and death. All patients who had an adverse event had suprasystemic pulmonary artery pressures and moderate to severe RV dysfunction. In yet another study of 284 non–cardiopulmonary bypass surgical cases, suprasystemic PAH, young age, and home oxygen use were significant risk factors for complications, whereas the cause of PAH was not associated with an increased risk. Van der Griend et al. evaluated 101,885 anesthetics of pediatric patients and found that 50% of all deaths occurred in patients with PH. The deaths encompassed the entire perioperative period—induction of anesthesia, maintenance of anesthesia, and in the postoperative care unit. Another large study evaluating 43,391 pediatric patients undergoing anesthesia for surgery in Spain showed that the presence of PAH made death more likely if a cardiac arrest occurred.
Comorbid conditions augment perioperative risk for children with PH. Sleep-disordered breathing, reactive airway disease, chronic lung disease, chronic aspiration, obesity, sickle cell disease, neuromuscular disease, and congenital or acquired cardiac disease can each decrease cardiopulmonary or airway reserve and increase risk of decompensation. In a retrospective multicenter study of infants with bronchopulmonary dysplasia who underwent surgical repair of CHD, 25% of the deaths were attributed to PH. Chi and Krovetz reported that children with Down syndrome, who may have underlying upper airway obstruction and pulmonary alveolar hypoplasia, have an unusually high PVR and a propensity for early development of severe PVD.
Finally, anesthetic and surgical factors can increase the risk for morbidity and mortality in patients with PH. These include emergency surgical procedure, ASA III or poorer status, presence of a difficult airway (which may potentially lead to hypoxemia or hypercarbia either while attempting to secure the airway or immediately after extubation), prolonged procedure times (which can cause atelectasis and necessitate increased fluid administration), intraoperative hemodynamic lability, procedures that can generate compressive forces on the heart and lung (intrathoracic and abdominal surgery), interventions that cause a systemic inflammatory response (e.g., abdominal washout for abscess, bowel resection), and surgery that may provoke major fluid shifts (e.g., exploratory laparotomy, orthopedic trauma). Of special consideration are procedures in the cardiac catheterization laboratory because they are associated with high risk of cardiac arrest, especially during pulmonary angioplasty and stenting, in which direct stimuli to the pulmonary arterial tree is applied.
Given the high-risk nature of this patient population, communication among all providers, including nurses, surgeons, anesthesiologists, intensive care unit (ICU) team, PH specialists, and the primary physician, must be standard of practice and must encompass the following checklist: (1) medical optimization before elective surgical cases without interruption in the administration of pulmonary vasodilators; (2) scheduling procedures early in the day to avoid prolonged fasting and dehydration; (3) assignment of an experienced pediatric anesthesiology team that is skilled in acute PH management; (4) availability of postoperative care in a pediatric intensive care unit as needed; (5) minimization of anesthetic exposure; and (6) immediate availability of pediatric subspecialists, nurses, and pharmacologists who are skilled in the care of children with PH. Management priorities in specific subtypes of CHD are outlined in Table 71.1 .
Noninvasive Assessment of Pulmonary Hypertension
Echocardiography for Pulmonary Hypertension
Echocardiography has been a first-line test in the evaluation of the intensive care patient with PH. The benefits include bedside availability, minimal coordination of staff, relatively low cost, and the fact that it is noninvasive. Although echocardiography is a very useful tool in the assessment and ongoing care of these patients, the child with PH presents unique challenges to echocardiographic assessment. These include limited acoustic windows due to hyperinflation accorded by often concomitant lung disease, ventilator modes such as high-frequency ventilator oscillation that challenge logistics and compromise image quality, and the risks inherent to sedating pediatric patients with PH. Additionally, the right ventricle is a major imaging target for PH, and its proximity to the airways as an anterior cardiac structure presents a challenge.
Quantification of right heart pressure can be achieved noninvasively in several ways but depends on the presence of right-sided valve regurgitation or cardiac shunts. Right ventricular systolic pressure (RVSP) is most often estimated by interrogating tricuspid regurgitation (TR) velocity by continuous wave Doppler evaluation ( Fig. 71.2 ; ). RVSP is equivalent to pulmonary artery systolic pressure when pulmonary stenosis and RV outflow obstruction have both been excluded—determination that must be made on initial imaging. TR velocity (meters/s) estimates the RVSP (mm Hg) using the modified Bernoulli equation but requires either direct catheter measurement or estimation of right atrial (RA) pressure (mm Hg):
RVSP = 4 × ( TR velocity ) 2 + RA pressure

Pulmonary regurgitation velocity should also be interrogated, when present, and this may provide an estimate of pulmonary artery end-diastolic pressure (PAEDP):
PAEDP = PR gradient at end diastole + RV diastolic pressure
RV diastolic pressure is approximated by RA pressure in the absence of tricuspid valve stenosis. When cardiac shunts exist, specifically a PDA ( ) or VSD, these velocities should be estimated, and the Bernoulli equation should be applied. Subtracting the resulting gradient from a simultaneous systemic systolic blood pressure will yield an estimate of pulmonary artery systolic pressure or an RV systolic pressure estimate—depending on the location of the shunt. When more than one of these shunts and tricuspid valve regurgitation are available, it is useful to use multiple methods to cross-reference the validity of the estimated right heart pressure.
Disappointingly, over 50% of patients do not have enough TR to estimate RV pressure and do not have any shunts. Indirect methods are then used to determine RV pressure. Specifically, this entails evaluation of the ventricular septal geometry in systole. When flattened ( Fig. 71.3 ; ) in systole, the RV pressure is greater than 50% systemic.

Serial assessment of RV function may also be necessary in the care of the ICU patient with PH. The RV function is known to be adversely affected (at rest) when more than 50% of the pulmonary resistance vessels are dysfunctional ( ), and preservation of RV function is a critical prognostic factor in management of PH in adults. Barriers to quantification of RV systolic function include RV geometry, as well as image quality. Several methods exist to assess RV function, but their use widely varies between centers. Tricuspid Annular Planar Systolic Excursion (TAPSE) is perhaps the most widely used, and normal values exist for children, showing a positive correlation to age (see Fig. 71.3 ). This method measures the longitudinal displacement of the tricuspid valve annulus in systole, recognizing that the RV fiber orientation is more longitudinal than that of the left ventricle. It is measured using the M-mode of echocardiography along the lateral aspect of the tricuspid annulus in the apical four-chamber view, evaluating the maximum movement of the annulus from high in the atrium during diastole to down into the right ventricle during systole. Decreased values have been associated with poor prognosis in adults with PH and heart failure. Limitations of this method include the fact that it is dependent upon load, angle, and orientation of the heart within the chest. Additionally, the focus is limited to a small part of the RV myocardium—the basolateral wall, and it is affected by the overall motion of the heart. RV shortening fraction is another method for assessment of RV systolic function but is dependent on loading conditions, and there are currently no reference values for RV shortening fraction in children. Pulmonary artery acceleration time is a relatively new method proposed for RV functional assessment that inversely correlates with right heart catheterization-based pulmonary hemodynamics, including mPAP and PVR. However, this method is also dependent upon heart rate, and normal values are available only for older children.
Two other methods that bear mentioning include RV speckle tracking and RV Tei index (sum of RV isovolumetric contraction time + RV isovolumetric relaxation time, divided by the RV ejection time) because both have been studied in adults with PH. Unfortunately, the RV Tei index has not been found to be helpful in assessing the RV function during the newborn transition period, which is often the age and stage of one of the most common pediatric ICU patient types with CDH and PH.
In summary, bedside echocardiography will continue to be the mainstay method for evaluation of right heart pressure and RV function in children with PH in the ICU, with some promising new techniques for its application on the horizon.
Biomarkers for Pulmonary Hypertension
Serum biomarkers of disease severity and response to treatment in PH are highly valued tools that are under active investigation and development. The biomarker that is currently used for pediatric PH is the amino-terminal fragment of the pro-form of the brain natriuretic peptide (BNP)—NTproBNP. This is an inactive byproduct of enzymatic cleavage of BNP that is triggered by wall stretch in the right atrium and in the right ventricle. NTproBNP levels have been shown to correspond well to changes in PVR and to echocardiographic measurements of PH severity in CHD, and it is the current standard for periodic assessment of children with an active PH course. More specific markers are under development due to a higher range of normal NTproBNP values in pediatrics and more variability in some clinical settings—particularly those with active noncardiac systemic disease. Promising candidates for development as additional biomarkers with potential for higher specificity include hepatoma-derived growth factor and microRNA’s miR-208b and miR-199a.
Cardiac Catheterization in Pulmonary Hypertension
Indications
Cardiac catheterization can provide definitive and extensive information about hemodynamics, responsiveness to pulmonary vasodilators, and cardiovascular anatomy in PH patients. Furthermore, cardiac catheterization allows interventions to be performed, which can alleviate or palliate PH or otherwise facilitate PH management.
Various recommendations have been made regarding which children with PH should undergo cardiac catheterization and when. In one series of formerly premature infants with bronchopulmonary dysplasia and PH, 31% underwent cardiac catheterization. Mourani et al. in 2008 emphasized the inability of echocardiography to reliably assess PH severity in a significant proportion of young patients. Bobhate et al. in 2015 proposed catheterization for most children with PH with the exception of those in overt right heart failure or requiring mechanical ventilation and inotropic support. Guidelines from the American Heart Association and the American Thoracic Society recommend catheterization before initiation of therapy, except for critically ill patients requiring immediate treatment. Guidelines recently published by the Pediatric and Congenital Heart Disease Task Forces of the Pulmonary Vascular Research Institute specify that nearly all children with PH should undergo diagnostic cardiac catheterization with acute vasoreactivity testing (AVT) at least once. In general, catheterization should be undertaken when the cause, severity, and/or course of PH is to be assessed; when acute responsiveness to pulmonary vasodilators is to be determined; when severe PH is not satisfactorily responding to therapy; when transcatheter or surgical therapy may be indicated; to assess operability; and to evaluate candidacy for heart or heart-lung transplantation.
Catheterization Procedure
Catheterization for pediatric patients with PH often requires general anesthesia. Some centers have developed strategies to avoid or minimize general anesthesia for selected patients. Anesthesia and catheter manipulations do entail risk. An earlier study reported resuscitation or death in as many as 6% of patients. However, a later registry study showed that cardiac catheterization for pediatric patients with PH can be performed with few adverse events and zero mortality. Zuckerman et al. in 2013 reported a 5.7% complication rate, a 1.2% major complication rate, and a 0.2% mortality rate associated with cardiac catheterization for children and adults with PH. A recent study from another single center reported a 6% rate of complications but no deaths. Due to potential for complications to occur during cardiac catheterization, pediatric patients with PH should undergo catheterization in tertiary care centers where cardiology and anesthesiology teams with requisite expertise and experience are available. Comprehensive guidelines for cardiac catheterization of pediatric patients with PH have recently been published by the Pulmonary Vascular Research Institute, Pediatric and Congenital Heart Disease Task Forces. Chest computed tomography angiography (CTA) should be considered in advance of catheterization to identify large airway, pulmonary parenchymal, and vascular anomalies and to guide catheterization planning. Furthermore, CTA may also identify patients with pulmonary venoocclusive disease or pulmonary vein stenosis in whom acute pulmonary vasoreactivity testing may lead to severe pulmonary edema ( Fig. 71.4 ).

Diagnostic criteria for PH are mPAP of 25 mm Hg or higher, mean PCWP of less than 15 mm Hg, and PVR of 3 Wood units or higher. Although these adult criteria are applied to children, it is necessary to be mindful that systemic blood pressure is typically lower in children, and therefore the adult criteria may be too strict for some children.
Cardiac catheterization allows informed decision making by quantitatively assessing acute responses to pulmonary vasodilators. Typically, baseline hemodynamic measurements (pressure, oxygen saturation, blood gas determinations, oxygen consumption measurements, and thermodilution measurements of cardiac output) are made while the patient is breathing room air or the patient’s baseline fraction of inspired oxygen (FiO 2 ) with supplemental oxygen. The FiO 2 is then increased to between 0.4 and 1.0 (depending on a given practitioner or center’s usual practice), and the hemodynamic measurements are repeated with this new condition. Nitric oxide in concentrations ranging between 20 and 80 ppm (depending on a given practitioner or center’s practice) may then be added to the inspired oxygen, and hemodynamic measurements are repeated. Responses to other agents such as intravenous or inspired prostacyclin analogues or enteral sildenafil may be assessed in similar fashion. Important derived hemodynamic results include pulmonary and systemic blood flow indexed to body surface area and pulmonary and systemic vascular resistances also indexed to body surface area. Responsiveness to pulmonary vasodilators in pediatric patients has been defined as a decrease in mPAP of more than 20% in the setting of increased or unchanged cardiac output. In addition, responsiveness is defined as a decrease in the ratio between PVR and systemic vascular resistance. This measured responsiveness is used to identify patients who are likely to be successfully treated with calcium channel blockers (CCBs) or more selective pulmonary vasodilators. Responsiveness has also been defined as decrease in mPAP by 10 to 40 mm Hg, together with an increase or no change in cardiac output. These latter criteria, however, are not applicable to all children whose baseline mPAP is less than 40 mm Hg despite the presence of severe PH. Some investigators have found other measures such as pulmonary artery capacitance index to be useful in predicting survival.
Beyond the assessment of the severity of PH and its responsiveness to pulmonary vasodilators, additional diagnostic information may be obtained from cardiac catheterization. First, contributions to PH by shunt lesions such as persistent foramen ovale or ASDs, VSDs, PDA, and aortopulmonary collateral arteries can be evaluated. Second, hemodynamic measurements and angiography can provide definitive information about the presence and significance of any pulmonary arterial and/or venous narrowings. Third, the presence and degree of left ventricular (LV) diastolic dysfunction can be assessed to guide further medical management. Fourth, inconclusive hemodynamic and angiographic findings can point to rare disorders such as alveolar-capillary dysplasia, pulmonary venoocclusive disease, or surfactant deficiency. Finally, serial cardiac catheterization enables assessment of progression of disease and response to therapy.
Catheter-Based Interventions for Pulmonary Hypertension
Balloon angioplasty and stent implantation are established procedures for treating pulmonary artery narrowings to improve distribution of pulmonary blood flow and reduce RSVP. More daunting, however, is the management of pulmonary venous stenosis ( Fig. 71.5 ). Although balloon angioplasty and stent implantation are regularly used as alternatives to open surgical intervention, outcomes have been mixed, with a significant proportion of patients experiencing disease progression and death despite intervention.

A variety of shunting lesions contribute to increased pulmonary artery pressure and PVR. These include ASDs, VSDs, PDAs, and aortopulmonary collateral arteries. Many, although not all, of these lesions can be closed during cardiac catheterization procedures.
In patients in whom these shunt lesions are associated with severe PH, closure can potentially exacerbate the patient’s clinical situation by removing an avenue for relief of suprasystemic pulmonary artery pressure via right-to-left shunting. Thus careful case selection of patients for intervention is necessary. For some shunts it is possible to perform temporary balloon occlusion by a balloon catheter, which then allows rapid assessment of the acute physiologic effects that closure may have before proceeding with permanent closure, either by transcatheter or surgical intervention.
Atrial septostomy is an established palliative intervention that can decompress the right ventricle and increase LV preload and cardiac output in the setting of elevated right heart pressure. Although systemic deoxygenation is a consequence of this intervention due to right-to-left shunting, the overall result may be favorable with improved clinical status, echocardiographic findings, 6-minute walk distance, functional class, and survival.
Compared with atrial septostomy, which causes deoxygenation of blood within the left heart from the right-to-left shunt, a pulmonary artery-to-descending aorta shunt may be advantageous because it limits the supply of deoxygenated blood to the descending aorta. Thus, blood supplying the coronary and cerebral circulations typically remains well saturated. Surgical and more recently transcatheter creation of left pulmonary artery-to-descending aorta shunts (Potts shunts) has been used to decompress the pulmonary circulation. Although one small study showed a mortality of 25% to 50%, outcomes in survivors are encouraging. In analogous fashion a small PDA may be enlarged with a stent to create a pulmonary artery-to-descending-aorta shunt.
Some children with hemoptysis due to PH will benefit from cardiac catheterization to embolize pulmonary arterial or aortopulmonary collateral sources of bleeding. In addition, when children with PH undergo cardiac catheterization with general anesthesia and remain hemodynamically stable, there can be an opportunity to perform procedures such as placement of a central venous catheter (percutaneous or tunneled); flexible bronchoscopy to evaluate for tracheobronchomalacia, look for signs of chronic aspiration, or remove mucous secretions; open lung biopsy for tissue diagnosis in suspected lung disease; or placement of a chest tube to drain a pleural effusion.
Emerging Interventions and Alternatives
Implantable hemodynamic monitoring devices that allow for ambulatory pulmonary pressure monitoring, such as the CardioMEMS HF System (St. Jude Medical, St. Paul, MN), require transcatheter placement. Such devices allow pulmonary artery pressures to be assessed in real time, and trends may be evaluated to guide management.
It is recognized that sympathetic overactivation occurs in patients with PH and that increased pulmonary artery pressure is associated with pulmonary nerve stimulation. Limited experimental and clinical evidence has been published to suggest that transcatheter pulmonary artery denervation to treat PH may be beneficial.
Electrical and mechanical ventricular dyssynchrony is common in pediatric patients with PH. RV pacing in adult patients with PH can lead to improved diastolic relaxation and increased stroke volume. Resynchronization therapy for RV failure in PH has yet to become established as a standard strategy of treatment for PH.
In conclusion, it is clear that cardiac catheterization remains an important diagnostic tool for the management of pediatric PH. It is often a valuable therapeutic tool as well. Emerging technologies will both modify the use of and increase the utility of cardiac catheterization in the care of children with PH.
Treating Children With Pulmonary Hypertension in the Intensive Care Unit
Pulmonary Hypertensive Crisis Management
Pulmonary hypertensive crisis may be defined as two or more of the following changes that occur acutely over a period of 10 minutes or less: a decline in systemic blood pressure by greater than 20% from baseline (the average blood pressure for the hour before the change); a decline in oxygen saturation to less than 90% in acyanotic patients or a decline of more than 10% in cyanotic patients in the absence of any other obvious cause; an increase in central venous pressure (CVP) by more than 20%; and an increase or decrease in heart rate by 20% (excluding patients with epicardial pacing). Progressive worsening of PH crisis frequency and severity may be presaged by elevation of NTproBNP, but this may not be the case in an isolated episode.
The ICU management of a PH crisis requires an integrative approach that is focused on the treatment of increased PVR and its impact on RV function. Initial treatment should be the administration of oxygen due to its ease of administration, quick onset of action, and selective pulmonary vasodilation. In situations in which controlled ventilation can be performed, minute ventilation should be increased to reduce the effect of hypercarbia on PVR, while avoiding excessive positive end-expiratory pressure, high peak pressures, and long inspiratory times that may cause lung overdistention and reduce preload to a challenged RV. Furthermore, if the patient is ventilated, it is prudent to use sedation strategies to attenuate sympathetic outflow that can increase PVR. Bolus doses of sedatives are often helpful—narcotics and dexmedetomidine offer hemodynamic stability, whereas benzodiazepines and barbiturates are more likely to have adverse effects. In addition, neuromuscular blockade can facilitate patient-ventilator synchrony and reduce PA pressure. Coexistent metabolic acidosis should be corrected because PVR is also directly related to H + concentration. When such interventions do not result in resolution of the PH crisis, pharmaceutical interventions targeting PVR are necessary ( Fig. 71.6 ). As detailed earlier, agents such as prostacyclin analogues, nitric oxide (NO), and phosphodiesterase (PDE) inhibitors provide avenues for modulating PVR. When RV dysfunction persists during a PH crisis, low cardiac output may result due to decreased left heart filling and septal bowing to the left that reduces LV ejection. Ensuring adequate preload and administering inotropic support with either dopamine or epinephrine promotes forward flow of blood across the pulmonary vasculature to the left heart. Although milrinone is useful for patients with ventricular dysfunction due to PH, its long onset of action and propensity to cause hypotension limit its usefulness in an acute PH crisis. Furthermore, vasopressor support to maintain SVR and enhance coronary perfusion may be necessary to avoid RV ischemia. Vasopressin is often chosen because it increases SVR with a minimal effect on increasing PVR. If cardiac output continues to decline and/or hypoxemia persists, extracorporeal membrane oxygenation (ECMO) should be considered.

Directed Pharmacotherapy for Pulmonary Hypertension
Overall therapeutic goals in pediatric PH are to dilate and reverse the abnormal pulmonary vascular bed, restore endothelial function, and prevent PH crisis. The most common agents used to achieve this are directed at the NO, prostacyclin, and endothelin pathways. A summary of current pharmacotherapies for children with PH is shown in Table 71.2 , and mechanisms of action are shown in Fig. 71.6 . A discussion of each category of pulmonary vasodilators and other agents follows here.
Pharmacologic Pathway | Medication Class | Mechanism of Action | Therapeutic Agents | Route of Administration | Adverse Effects |
---|---|---|---|---|---|
34 | Mean (SD), 38.3 (1.7) weeks’ GA | Comparator: MgSO 4 (serum level 7-11 mg/dl) | 200 mg/kg IV LD then 20 mg/ kg/h to max 100 mg/kg/h; tapered and stopped in 1d when OI <15 and PAP <20 | ||
cGMP augmentation | iNO | Via diffusion across the alveolar-capillary membrane →↑cGMP in pulmonary smooth muscle vasculature → smooth muscle relaxation | iNO | Inhaled | Methemoglobin Formation of NO 2 |
l -Arginine | Increases endogenous NO synthesis | l -Arginine | Oral Intravenous | –––– | |
PDE-5 inhibitors | Via PDE-5 inhibition →↑cGMP in pulmonary smooth muscle vasculature → pulmonary vasodilation and inhibition of vascular remodeling | Sildenafil | Oral Intravenous | Headache Flushing Dizziness Hypotension Priapism | |
Tadalafil | Oral | ||||
Vardenafil | Oral | ||||
cAMP augmentation | Prostacyclin analogues | Via cell-surface G-protein receptors on pulmonary endothelial cells, or platelets → ↑cAMP → pulmonary and systemic vasodilation, inhibition of vascular remodeling and inhibition of platelet aggregation | Epoprostenol | Intravenous Inhaled | Flushing Headache Hypotension Jaw pain Thrombocytopenia Bronchospasm (Iloprost) |
Treprostinil | Intravenous Oral Subcutaneous | ||||
Iloprost | Intravenous Inhaled | ||||
Beraprost | Oral | ||||
PDE-3 inhibitors | Via PDE-3 inhibition →↑cAMP in arterial smooth muscle cells and cardiac myocytes → pulmonary and systemic vasodilation and inotropy | Milrinone | Intravenous Inhaled | Hypotension Thrombocytopenia | |
Endothelin receptor blockade | ET-1 antagonists | Via specific competitive dual endothelin receptor A and B blockade → pulmonary vasodilation and inhibition of vascular remodeling | Bosentan | Oral | Liver toxicity Anemia Headache Flushing Nasal congestion Peripheral edema Extremity pain Teratogenicity |
ET-A antagonists | Via selective endothelin receptor A blockade → pulmonary vasodilation, antiproliferation and ET-1 clearance | Ambrisentan Sitaxentan | Oral | ||
Vasoactive intestinal peptide | Blocks pulmonary vasoconstriction caused by ET-1 | Vasoactive intestinal peptide | –––– | Does not cause increased airway resistance often seen with other ET-1 antagonists | |
Calcium channel blockers | Calcium channel antagonists | Reduce calcium influx into vascular smooth muscle and cardiac myocytes | Nifedipine Diltiazem Amlodipine | Oral Intravenous | Negative inotropic effect Caution <1 year of age |
Anticoagulation | –––– | Varying depending on anticoagulant selected | Heparin Coumadin Lovenox | Oral Intravenous Subcutaneous | Bleeding Unclear indications |
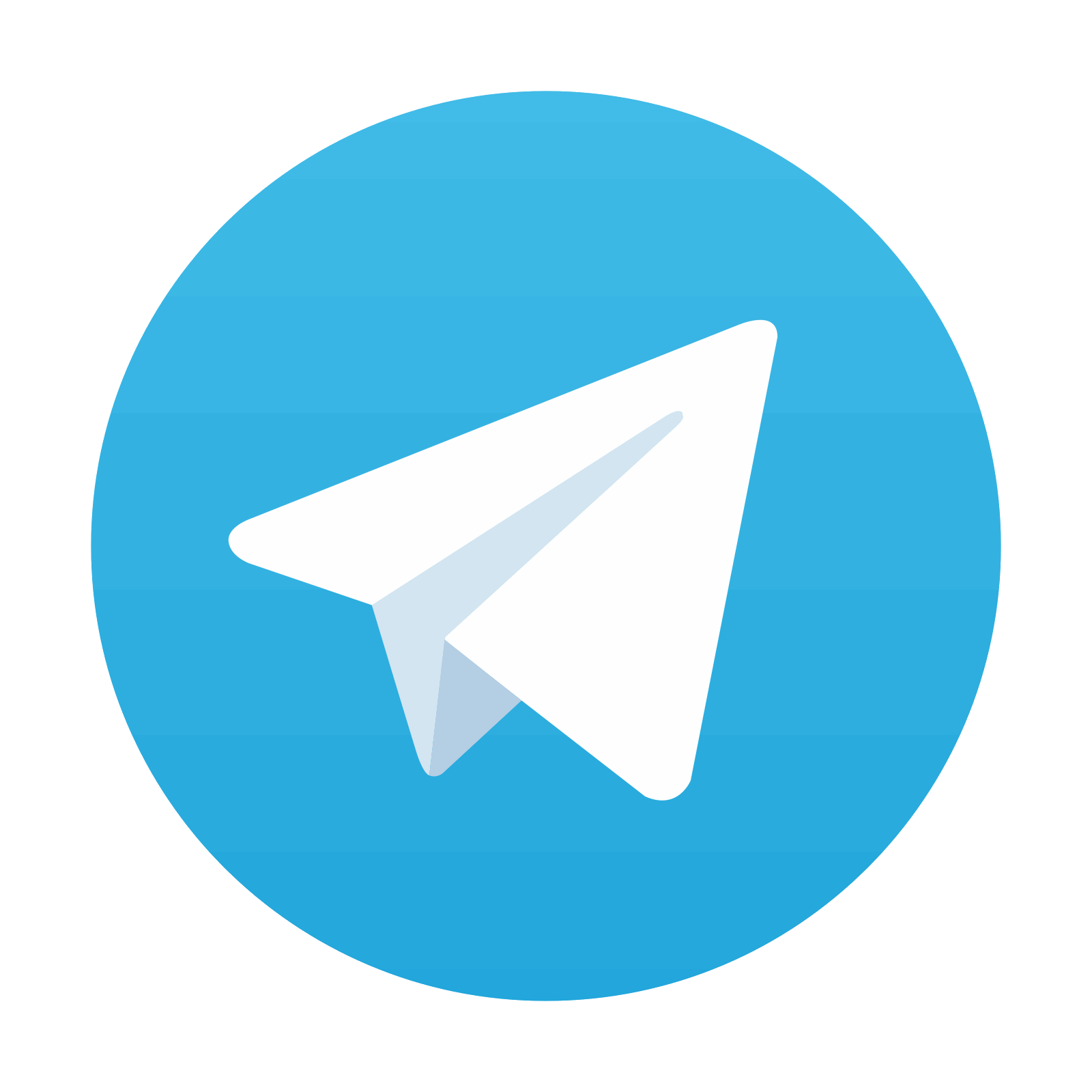
Stay updated, free articles. Join our Telegram channel

Full access? Get Clinical Tree
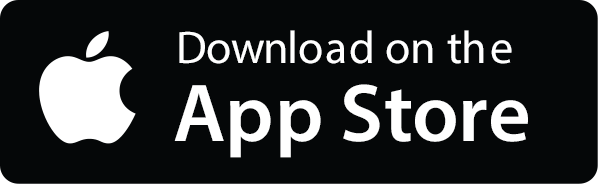
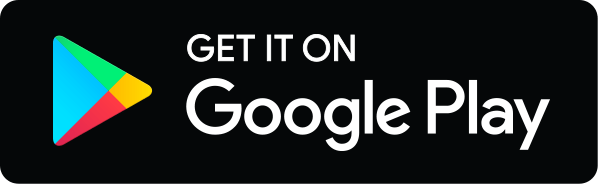
