Pulmonary embolism (PE) is defined as a thrombosis that arises in the venous system and subsequently embolizes to the pulmonary arterial circulation. Despite the advent of modern medical therapies, PE remains a commonly occurring condition associated with significant morbidity and mortality. There is a well-established decreased incidence of PE with appropriate prophylaxis and a significant improvement in patient outcome associated with the prompt institution of appropriate therapy; hence, rigorous screening, early diagnosis, effective thromboprophylaxis, and early therapy are warranted.1 In the United States, it is estimated that 600,000 cases of PE occur each year2 with a mortality rate in the first 3 months after diagnosis of greater than 15%.3 Despite our increased knowledge about the condition and ever-increasing technologic sophistication, mortality rates associated with PE remain high and relatively unchanged over the past half century.4 Additionally, autopsy studies continue to demonstrate that the diagnosis of PE is made less than 50% of the time before death.5 Although specific risk factors have been identified for the occurrence of PE and numerous effective prophylactic regimens have been demonstrated, recent studies show that appropriate prophylactic regimens continue to be significantly underused.6
PE results from the detachment and migration of thrombi fragments that lodge within and obstruct blood flow to a single or multiple areas of the pulmonary vascular bed (Figures 21-1 and 21-2). Pulmonary emboli generally origin from the detachment of deep venous thrombi (DVTs) of the proximal lower extremities accounting for up to 50% of PE.7 Less commonly, deep pelvic veins or proximal upper extremity DVTs associated with central venous catheters are the source of pulmonary emboli.8 PE is, therefore, part of the continuum of venothromboembolism (VTE).
A cascade of pathophysiologic mechanisms ensues with the pulmonary embolic event that may lead to hypoxemia and cardiovascular compromise. After traveling to the lung, large thrombi may lodge at the bifurcation of the main pulmonary artery or the lobar branches and cause hemodynamic compromise. The anatomical obstruction is the primary insult resulting from the PE. The reduction of the cross-sectional area of the pulmonary arterial bed caused by the embolism has been shown to correlate with the degree of physiologic impairment. Pulmonary arterial pressure generally begins to increase with a 25% to 30% occlusion of the pulmonary vascular bed in otherwise normal heart and lungs9; greater than 50% obstruction is generally present before a clinically significant elevation occurs. Increased right ventricular afterload can cause right ventricular dilatation, hypokinesis, tricuspid regurgitation, and eventual right heart failure.10 A normal right ventricle can fail with acute increases of the pulmonary arterial pressure to 40 to 50 mm Hg when obstruction of the pulmonary vascular bed approaches 75%. PE in patients with preexisting cardiopulmonary disease may experience more severe physiologic impairment with lesser degrees of pulmonary vascular obstruction.11 The increase in pulmonary vascular resistance that occurs with PE can impede cardiac right ventricular outflow and diminish left ventricular preload to cause an acute decrease in forward cardiac output and, ultimately, hemodynamic collapse. Hypoxic pulmonary vascular vasoconstriction and the release of neurohumoral factors such as serotonin incited by the embolic event are additional factors that can contribute to increases in pulmonary vascular resistance and the resultant adverse hemodynamic consequences.12
Hypoxemia results from pulmonary emboli by a number of mechanisms. PE creates a redistribution of pulmonary blood flow to alter ventilation/perfusion (V/Q) characteristics in the lung, thereby decreasing the efficiency of alveolar gas exchange.13 Atelectasis may also occur and contribute to altered V/Q relationships and gas-exchange impairment.10 A right-to-left shunt may develop with PE by the intrapulmonary passage of blood through unventilated gas exchange units or intracardiac shunting of blood through a patent foramen ovale associated with increases in right atrial pressure occurring with PE.12 The inability to correct arterial hypoxemia with supplemental oxygen suggests the existence of intrapulmonary or intracardiac right-to-left shunting of blood. Additionally, decreased cardiac output and hypoxemia can lead to a low venous blood oxygen tension, which may amplify gas exchange impairment through lung gas exchange units with poor V/Q characteristics.
Although arterial hypoxemia and an increased alveolar–arterial oxygen gradient are the most common abnormalities of gas exchange, hyperventilation is common with PE and can cause hypocapnia and respiratory alkalosis. However, PE can increase both anatomic and physiologic dead space, thus impairing carbon dioxide removal. Hypercapnia associated with PE suggests underlying obstructive airway disease or a massive clot burden.14 Patients with underlying cardiopulmonary disease experience more substantial deterioration in cardiac output than do normal individuals. In addition, right ventricular failure after PE is more common in patients who have coexistent coronary artery disease.
General risk factors for VTE include older age, immobility, surgical procedures, congestive heart failure, stroke and paralysis, trauma, malignancy, and hypercoagulable states. However, numerous clinical circumstances associated with an increased risk for the development of VTE have been identified. Most patients with PE have more than one risk factor.15 Various degrees of risk have been defined for individual risk factors, with some patient populations having a greater than 50% incidence of VTE.16 Knowledge of these risk factors and a thorough patient history investigation for these factors are needed to develop an efficient and rational approach to the evaluation, diagnosis, and treatment of PE for the individual patient, particularly because presenting clinical signs and symptoms are insensitive and nonspecific for the diagnosis of PE. Certain acquired risk factors (i.e., total hip replacement, knee replacement) increase the likelihood of acute DVT and thus PE. In patients with conditions such as cancer and the thrombophilias, acquired risk and genetic predisposition may overlap (Table 21-1).
Prolonged air travel Obesity Cigarette smoking Immobility Increasing age Medical conditions Hypertension Prior PE or DVT Cancer Congestive heart failure Diabetes mellitus Inflammatory bowel disease Paresis Nursing home confinement Varicose veins Foreign bodies or particles Indwelling central venous catheter Permanent pacemaker Internal cardiac defibrillator IV air Amniotic fluid IV fat IV cement Women’s health Oral contraceptives Pregnancy Hormone replacement Surgical Trauma Orthopedic: especially high incidence with total hip replacement, hip fracture repair, and total knee replacement General surgery: especially high incidence with cancer surgery Gynecologic: especially high incidence with cancer surgery Neurosurgery: especially high incidence with brain tumor Hematologic Thrombophilia Factor V Leiden mutation Prothrombin gene mutation Hyperhomocysteinemia Antiphospholipid syndrome Antithrombin III, protein C, protein S deficiencies Dysfibrinogenemia Plasminogen deficiency MTHFR gene mutation |
Symptoms and physical examination findings have been consistently shown to be insensitive and nonspecific for the diagnosis of VTE.17,18,19,20 One or more elements of Virchow’s triad (stasis, hypercoagulability, and intimal injury) are present in the vast majority of patients with VTE.15 Classic clinical findings of DVT include pain and swelling of the affected extremity, warmth and superficial venous dilatation, a thrombosed venous cord, and pain upon passive dorsiflexion of the foot (Homan’s sign). Patients with PE frequently present with symptoms of dyspnea, cough, pleuritic chest pain, agitation, symptoms of DVT, hemoptysis, syncope, and palpitations. The signs include tachypnea, tachycardia, fever, increased P2, signs of DVT, increased jugular venous pressure, shock, friction rub, and wheezing.7 Lightheadedness, syncope, and hypotension may occur and may indicate a greater clot burden. Patients with massive PE may present with hemodynamic abnormalities, including shock, refractory hypoxemia, or cardiopulmonary arrest.21 Patients with underlying cardiopulmonary disease may develop more severe pathophysiologic consequences of PE with less clot burden.22
The initial laboratory evaluation has also been shown to be insensitive and nonspecific for the diagnosis of PE.23 Most patients with PE have chest radiograph findings that are abnormal but nonspecific.24 Platelike atelectasis, pleural effusions, pulmonary infiltrates, and hemidiaphragm elevation have been commonly found on chest radiographs in patients presenting with PE, but these findings are also observed with numerous other conditions. Hampton’s hump and Westermark’s sign are considered to be suggestive of PE, although they are present infrequently. A normal chest radiograph in a patient with dyspnea and hypoxemia without bronchospasm or evidence of anatomic cardiac shunt suggests a high likelihood of PE.25
Arterial blood gas analysis, as well, cannot confirm or exclude the diagnosis of PE. As discussed, arterial blood gas abnormalities commonly found in the setting of PE include arterial hypoxemia, increased alveolar–arterial oxygen gradient, hypocapnia, and respiratory alkalosis. Hypercapnia and respiratory acidosis are signs of underlying cardiopulmonary disease or a massive PE. A normal arterial oxygen pressure and, less commonly, a normal alveolar–arterial oxygen gradient may be present, particularly in patients who are young with otherwise normal lungs.26 Although arterial blood gas analysis has been shown to be neither sensitive nor specific for the diagnosis of PE, correlation has been demonstrated between the extent of the clot burden and the degree of the alveolar–arterial oxygen gradient.23
Electrocardiogram (ECG) abnormalities are seen in up to 80% to 90% of cases of PE; however, a wide variety of abnormalities can be observed, and these findings are not sensitive or specific for the diagnosis of PE.27 A major limitation of studies on ECG abnormalities and PE is that most studies have been done on patients after the diagnosis of PE has been confirmed. Sinus tachycardia and incomplete right bundle branch block have been found more often in confirmed than suspected cases.28 SIQIIITIII is a nonspecific and infrequent finding that may suggest right heart strain from a massive or submassive PE. Thus, ECG analysis may be most useful to identify or exclude diagnoses other than PE.
As reviewed above, patients with PE can present with a variety of symptoms, signs, and initial diagnostic studies, although it is unusual to be able to confirm or exclude the diagnosis of PE based on these findings. Initial evaluation based on these data, therefore, is generally most useful in assigning a probability of PE in a particular patient. Additionally, specific quantitation of this probability cannot be determined because of differences in patient populations and discrepancies in study results. However, a qualitative assessment of the probability of PE can usually be determined on the basis of the initial presentation data, which can then be used to guide the subsequent approach to further diagnostic evaluation and therapy. The initial presenting data should be used to determine a qualitative pretest probability for further diagnostic testing, as well as to consider when evaluating the potential risks and benefits of therapeutic options.
D-dimer is a fibrin degradation product present in the circulation that is produced by endogenous fibrinolysis of cross-linked fibrin. A number of different methods have been developed as assays for D-dimer. Enzyme-linked immunosorbent assay (ELISA), enzyme-linked fluorescent assay (ELFA), and newer generation latex agglutination assays have been shown to have a high sensitivity for PE. When used in conjunction with a clinical scoring system such as Wells Criteria,29 Geneva Score,30 or Charlotte Rule,31 these tests have been demonstrated in some studies to have sufficient negative predictive value to rule out the diagnosis of PE in the emergency department setting32 (Table 21-2). The specificity of these assays, however, is poor, because D-dimer levels have been found to be elevated with many conditions.33 Additionally, D-dimer assays have been found to be less accurate in hospitalized patients.34 Therefore, D-dimer assays are not useful in confirming the diagnosis of PE or in ruling out the diagnosis of PE for hospitalized patients. Other biomarkers, such as serum troponin and brain natriuretic peptide, may become useful to diagnosis or further characterize PE.7 However, sufficient studies have not yet been completed to clearly define the role of these biomarkers at this time.
Canadian (Wells) prediction score |
Variable and score DVT symptoms and signs—3.0 PE as likely as or more likely than alternative diagnosis—3.0† Heart rate>100 beats/min—1.5 Immobilization or surgery in previous 4 wk—1.5 Previous DVT or PE—1.5 Hemoptysis—1.0 Cancer—1.0 Total score‡ <2.0—low pretest probability 2.0 to 6.0—moderate pretest probability >6.0—high pretest probability Dichotomized Wells score§ ≤4—PE unlikely >4—PE likely |
Original Geneva (Wicki) score¶ |
Variable and score Age 60–79 yr—1 ≥80 yr—2 Previous DVT or PE—2 Recent surgery—3 Heart rate>100 beats/min—1 PaCO2 <36.2 mm Hg (<4.8 kPa)—2 36.2–38.9 mm Hg (4.8–5.19 kPa)—1 PaO2 <48.8 mm Hg (<6.5 kPa)—4 48.8–59.9 mm Hg (6.5–7.99 kPa)—3 60–71.2 mm Hg (8.0–9.49 kPa)—2 71.3–82.4 mm Hg (9.5–10.99)—1 Chest radiograph Platelike atelectasis—1 Elevation of hemidiaphragm—1 |
Revised Geneva score|| |
Variable and score Age>65 yr—1 Previous DVT or PE—3 Surgery or lower limb fracture in previous wk—2 Active cancer—2 Unilateral lower limb pain—3 Hemoptysis—2 Heart rate 75–94 beats/min–3 ≥95 beats/min—5 Pain on leg palpation or unilateral edema—4 |
V/Q scanning has been used frequently as a diagnostic study for the evaluation of acute PE and, under the appropriate clinical circumstances, may yield results that the clinician can use to confirm or exclude the diagnosis of PE. However, V/Q scanning is nondiagnostic for PE in the majority of cases.35,36 The collaborative Prospective Investigation of Pulmonary Embolism Diagnosis (PIOPED) trial helped shape consensus on the interpretation of V/Q scans. Important information determined from this investigation included the finding that the degree of pretest clinical suspicion is crucial in determining the probability of PE when V/Q scanning is used. It was found that a low-probability V/Q scan was associated with PE in 40% of patients with a high clinical suspicion of PE and that a high-probability scan was associated with only a 56% incidence of PE in patients with a low clinical suspicion of PE. However, a normal or near-normal perfusion scan was found to be associated with a low incidence of PE in all clinical risk groups studied, and a low-probability V/Q scan for patients with a low clinical probability was associated with a very low incidence of PE (4%). Additionally, a high-probability scan in patients with a high clinical suspicion was associated with a 96% incidence of PE.37 V/Q scan can be considered as an appropriate pulmonary imaging study for patients in whom angiography may be disadvantageous.38
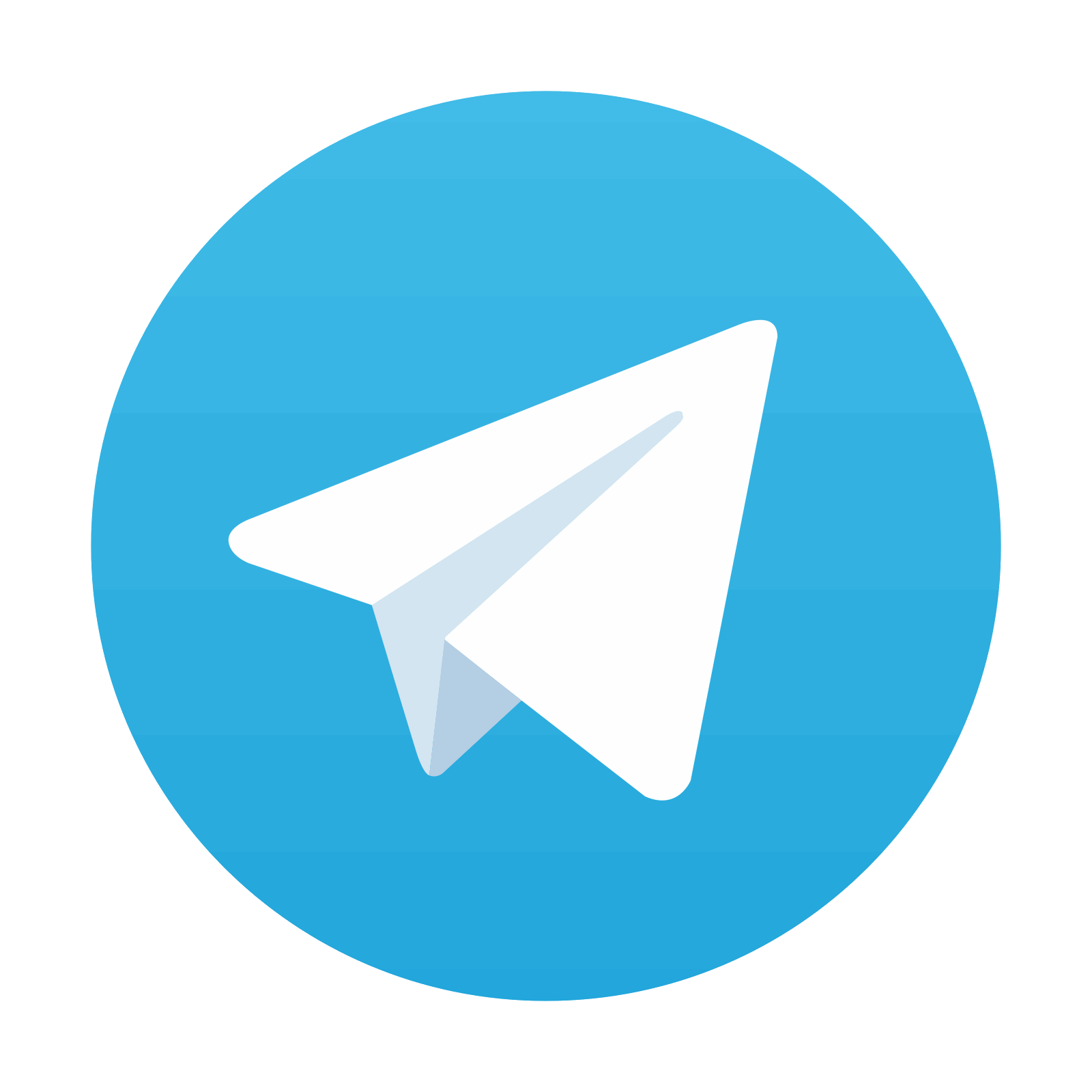
Stay updated, free articles. Join our Telegram channel

Full access? Get Clinical Tree
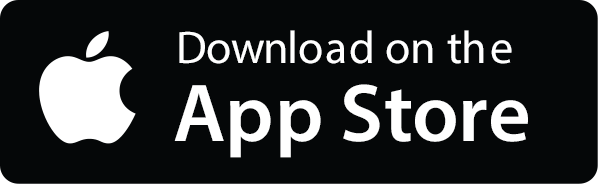
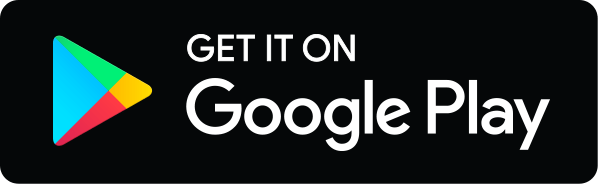