Background
Pulmonary artery acceleration time (PAAT) is a noninvasive method to assess pulmonary hemodynamics, but it lacks validity in children. The aim of this study was to evaluate the accuracy of Doppler echocardiography–derived PAAT in predicting right heart catheterization (RHC)–derived pulmonary artery pressure (PAP), pulmonary vascular resistance (PVR), and compliance in children.
Methods
Prospectively acquired and retrospectively measured Doppler echocardiography–derived PAAT and RHC-derived systolic PAP, mean PAP (mPAP), indexed PVR (PVRi), and compliance were compared using regression analysis in a derivation cohort of 75 children (median age, 5.3 years; interquartile range, 1.3–12.6 years) with wide ranges of pulmonary hemodynamics. To account for heart rate variability, PAAT was adjusted for right ventricular ejection time and corrected by the RR interval. Regression equations incorporating PAAT and PAAT/right ventricular ejection time from the derivation cohort were then evaluated for the accuracy of their predictive values for invasive pulmonary hemodynamics in a validation cohort of 50 age- and weight-matched children with elevated PAP and PVR.
Results
There were significant inverse correlations between PAAT and RHC-derived mPAP ( r = −0.82) and PVRi ( r = −0.78) and a direct correlation ( r = 0.78) between PAAT and pulmonary compliance in the derivation cohort. For detection of pulmonary hypertension (PRVi > 3 Wood units · m 2 and mPAP > 25 mm Hg), PAAT < 90 msec and PAAT/right ventricular ejection time < 0.31 resulted in sensitivity of 97% and specificity of 95%. In the derivation cohort, the regression equations relating PAAT with mPAP and PVRi were mPAP = 48 − 0.28 × PAAT and PVRi = 9 − 0.07 × PAAT. These PAAT-integrated equations predicted RHC-measured pulmonary hemodynamics in the validation cohort with good correlations ( r = 0.88 and r = 0.83, respectively), small biases (<10%), and minimal coefficients of variation (<8%).
Conclusions
PAAT inversely correlates with RHC-measured pulmonary hemodynamics and directly correlates with pulmonary arterial compliance in children. The study established PAAT-based regression equations in children to accurately predict RHC-derived PAP and PVR.
Attention ASE Members:
The ASE has gone green! Visit www.aseuniversity.org to earn free continuing medical education credit through an online activity related to this article. Certificates are available for immediate access upon successful completion of the activity. Nonmembers will need to join the ASE to access this great member benefit!
Methods
Study Design
In this study, we performed pulmonary hemodynamic assessment by DE and RHC in two cohorts of pediatric patients: (1) a derivation cohort to assess the correlations between DE-measured PAAT and RHC-measured PAP for the establishment of PAAT-based regression equations and (2) a validation cohort to substantiate these PAAT-based regression equation for their accuracy in predicting invasive measured pulmonary hemodynamics. In each cohort, DE and RHC were performed both simultaneously under the same loading and sedation conditions and nonsimultaneously to represent typical clinical condition in both cohorts.
Derivation Cohort
The derivation cohort consisted of 75 children (ages 1–18 years) who presented to the St. Louis Children’s Hospital cardiac catheterization laboratory for clinically indicated RHC between November 2011 and December 2013. DE and RHC were performed simultaneously ( n = 50 children) or within 6 hours ( n = 25 children) of each other (DE followed by RHC). The data were prospectively acquired and retrospectively analyzed. The 50 children who underwent simultaneous DE and RHC were recruited from a previous study reported by our group, in whom invasive pulmonary hemodynamics and PAAT were not reported. Children included in the study had a wide range of right heart pressures and diagnoses, including pulmonary arterial hypertension due to idiopathic, heritable, or connective tissue disease etiology, lesions with left-to-right shunt, and orthotopic heart transplantation, representing the spectrum of cardiopulmonary diseases seen in clinical practice ( Table 1 ). Children with single-ventricle physiology, mechanical tricuspid valves, RV outflow tract obstruction, left heart diseases, arrhythmias, or pulmonary insufficiency or on pulmonary vasodilators were excluded. Informed, written parental consent was obtained for all subjects and assent was obtained for all subjects >12 years of age. The institutional review board at Washington University School of Medicine approved all components of the study.
Variable | Study cohort ( n = 75) | Validation cohort ( n = 50) |
---|---|---|
Age (yrs) | 6.0 (1.4–14.2) | 8.0 (1.2–14.3) |
Women | 46 (61%) | 27 (54%) |
Caucasian | 84% | 80% |
Weight (kg) | 20 (11.4–48.2) | 23 (13.9–65) |
Body surface area (kg/m 2 ) | 0.70 (0.43–1.35) | 0.83 (0.55–1.18) |
Hemoglobin (mg/dL) | 11.1 ± 1.6 | 11.0 ± 1.9 |
Heart rate (beats/min) | 104 ± 22 (53–155) | 104 ± 19 (60–144) |
Systolic blood pressure (mm Hg) ∗ | 83 ± 15 (60–128) | 84 ± 22 (54–141) |
Diastolic blood pressure (mm Hg) ∗ | 52 ± 11 (35–87) | 55 ± 14 (30–90) |
Mean arterial blood pressure (mm Hg) ∗ | 63 ± 12 (44–103) | 67 ± 15 (60–144) |
Diagnosis | ||
Orthotopic heart transplantation | 22 | 13 |
Atrial septal defect | 10 | 3 |
Pulmonary artery hypertension | 32 | 28 |
Others | 11 | 6 |
Reason for RHC | ||
Diagnostic | 43 | 43 |
RV biopsy | 22 | 5 |
Atrial septal defect device closure | 6 | 1 |
Others | 4 | 1 |
∗ Simultaneous systemic blood pressure was obtained by arterial catheterization in patients in whom arterial access was clinically indicated or by upper extremity noninvasive cuff measurement.
RHC
Fifty children underwent simultaneous DE and RHC in the cardiac catheterization laboratory under the same sedation and loading conditions, and 25 children underwent DE first without sedation, followed by sedated RHC within 6 hours. RHC-measured mean PAP (mPAP) and systolic PAP (sPAP) using a standard fluid-filled catheter. An interventional cardiologist, blinded to the patients’ diagnoses and echocardiographic data, analyzed pressure tracings to evaluate pulmonary hemodynamics. Cardiac output and pulmonary blood flow were calculated using the standard Fick method and indexed to body surface area. Pulmonary artery compliance (mL/mm Hg/m 2 ) was calculated as the ratio of stroke volume (indexed pulmonary blood flow divided by heart rate) to pulmonary artery pulse pressure. PVR (Wood units [WU]) indexed to body surface area (PVRi; WU · m 2 ) was derived using pulmonary capillary wedge pressure from the following equation: (mPAP − pulmonary capillary wedge pressure)/indexed pulmonary blood flow. Oxygen consumption was assumed using the standard LaFarge methodology.
Echocardiography
We acquired two-dimensional and color Doppler echocardiographic images of the parasternal and apical standard views with the patient in the supine position per the American Society of Echocardiography guidelines. We developed a protocol for PAAT image acquisition and postprocessing data analysis ( Appendix 1 ) and tested for variability using reproducibility statistical analysis. A spectral Doppler image was obtained by placing a pulsed Doppler sample volume at the pulmonary valve annulus in the parasternal short-axis view ( Figure 1 ). Maximal alignment of Doppler interrogation with blood flow direction was achieved with the placement of the sample volume at the annulus of the pulmonary valve and not more proximally in the RV outflow tract. PAAT was calculated from a spectral Doppler envelope as the time interval between the onset of systolic pulmonary arterial flow (onset of ejection) and peak flow velocity ( Figure 1 ).

Confounding Influences
The variables confounding the PAAT measures include imaging acquisition techniques and postprocessing analysis, heart rate variability, influence of RV mechanics and function, and pulmonary compliance. We identified and quantified the image acquisition and postprocessing analysis uncertainties with the development of a protocol ( Appendix 1 ). To account for the potential impact of heart rate variability, PAAT was corrected for the R-R interval and also adjusted for RV ejection time (RVET) with the ratio of PAAT to RVET. The mean PAAT/RVET ratio from three cardiac cycles was used for data analysis and further corrected by the square root of the R-R interval. PAAT/RVET and the R-R interval were compared using a linear regression. We measured RV preejection time, RVET, and the ratio of RV preejection time to RVET to assess RV mechanics. Preejection time was defined as the interval between the onset of QRS complex to the onset of RV ejection, whereas RVET was defined as the interval between the onset of RV ejection to the cessation of systolic pulmonary arterial flow in the same cardiac cycle ( Figure 1 ).
We assessed RV function using two-dimensional speckle-tracking echocardiography–derived global longitudinal systolic strain, a sensitive and reliable measure with published normative values in children. One sonographer measured RV global longitudinal strain in the children who underwent simultaneous DE and RHC using a protocol previously validated by our group and others.
PAAT-Based Regression Equations and Cutoff Values
In the derivation cohort, PAAT and PAAT/RVET were compared with RHC-derived mPAP, sPAP, PVR, and PVRi using both linear and logarithmic regression analysis ( P values < .01 were considered to indicate statistical significance). A receiver operating characteristic (ROC) curve was constructed to determine cutoff valueS for PAAT and PAAT/RVET with the best sensitivity to predict pulmonary hypertension, defined as elevated PVR values (PVR > 2 WU and PVRi > 3 WU · m 2 ), elevated sPAP (>30 mm Hg), and mPAP > 25 mm Hg. The statistical analysis was performed using SPSS version 14.0 (SPSS, Chicago, IL).
Validation Cohort
The regression equations using PAAT and PAAT/RVET that were generated in the derivation cohort to predict PAP and PVR were subsequently validated in two separate validation subcohorts: (1) 25 age- and gender-matched pediatric patients with a wide range of pulmonary hemodynamics who were prospectively recruited and underwent simultaneous RHC and DE between January 2014 and December 2014 and (2) 25 age- and gender-matched pediatric patients with pulmonary arterial hypertension who underwent DE and RHC within 7 days of each other identified from a retrospective search of the database for the period between November 2011 and December 2013. We included this retrospective validation subcohort to demonstrate the applicability of the PAAT-based regression equations in routine clinical practice under no sedation. We assessed the predictive value of the PAAT-based regression equations to estimate PAP and PVR in both validation subcohorts by comparing with the RHC-derived pulmonary hemodynamics using (1) Bland-Altman plot analysis (percentage bias and 95% limits of agreement), (2) intraclass correlation, and (3) coefficient of variation. Bias < 10%, narrow 95% limits of agreement, a coefficient of variation <10%, and an intraclass correlation coefficient >0.8 were recognized as measures of minimal variability and positive reliability.
Reproducibility Analysis
Reproducibility of PAAT measurements was assessed using intra- and interobserver variability in 60% of the images from the derivation and validation cohorts. Three observers (P.T.L., M.D.P., and G.K.S.) performed offline analysis using the same measurement protocol and were blinded to the patients’ diagnoses, RHC data, and one another ( Appendix 1 ).
Methods
Study Design
In this study, we performed pulmonary hemodynamic assessment by DE and RHC in two cohorts of pediatric patients: (1) a derivation cohort to assess the correlations between DE-measured PAAT and RHC-measured PAP for the establishment of PAAT-based regression equations and (2) a validation cohort to substantiate these PAAT-based regression equation for their accuracy in predicting invasive measured pulmonary hemodynamics. In each cohort, DE and RHC were performed both simultaneously under the same loading and sedation conditions and nonsimultaneously to represent typical clinical condition in both cohorts.
Derivation Cohort
The derivation cohort consisted of 75 children (ages 1–18 years) who presented to the St. Louis Children’s Hospital cardiac catheterization laboratory for clinically indicated RHC between November 2011 and December 2013. DE and RHC were performed simultaneously ( n = 50 children) or within 6 hours ( n = 25 children) of each other (DE followed by RHC). The data were prospectively acquired and retrospectively analyzed. The 50 children who underwent simultaneous DE and RHC were recruited from a previous study reported by our group, in whom invasive pulmonary hemodynamics and PAAT were not reported. Children included in the study had a wide range of right heart pressures and diagnoses, including pulmonary arterial hypertension due to idiopathic, heritable, or connective tissue disease etiology, lesions with left-to-right shunt, and orthotopic heart transplantation, representing the spectrum of cardiopulmonary diseases seen in clinical practice ( Table 1 ). Children with single-ventricle physiology, mechanical tricuspid valves, RV outflow tract obstruction, left heart diseases, arrhythmias, or pulmonary insufficiency or on pulmonary vasodilators were excluded. Informed, written parental consent was obtained for all subjects and assent was obtained for all subjects >12 years of age. The institutional review board at Washington University School of Medicine approved all components of the study.
Variable | Study cohort ( n = 75) | Validation cohort ( n = 50) |
---|---|---|
Age (yrs) | 6.0 (1.4–14.2) | 8.0 (1.2–14.3) |
Women | 46 (61%) | 27 (54%) |
Caucasian | 84% | 80% |
Weight (kg) | 20 (11.4–48.2) | 23 (13.9–65) |
Body surface area (kg/m 2 ) | 0.70 (0.43–1.35) | 0.83 (0.55–1.18) |
Hemoglobin (mg/dL) | 11.1 ± 1.6 | 11.0 ± 1.9 |
Heart rate (beats/min) | 104 ± 22 (53–155) | 104 ± 19 (60–144) |
Systolic blood pressure (mm Hg) ∗ | 83 ± 15 (60–128) | 84 ± 22 (54–141) |
Diastolic blood pressure (mm Hg) ∗ | 52 ± 11 (35–87) | 55 ± 14 (30–90) |
Mean arterial blood pressure (mm Hg) ∗ | 63 ± 12 (44–103) | 67 ± 15 (60–144) |
Diagnosis | ||
Orthotopic heart transplantation | 22 | 13 |
Atrial septal defect | 10 | 3 |
Pulmonary artery hypertension | 32 | 28 |
Others | 11 | 6 |
Reason for RHC | ||
Diagnostic | 43 | 43 |
RV biopsy | 22 | 5 |
Atrial septal defect device closure | 6 | 1 |
Others | 4 | 1 |
∗ Simultaneous systemic blood pressure was obtained by arterial catheterization in patients in whom arterial access was clinically indicated or by upper extremity noninvasive cuff measurement.
RHC
Fifty children underwent simultaneous DE and RHC in the cardiac catheterization laboratory under the same sedation and loading conditions, and 25 children underwent DE first without sedation, followed by sedated RHC within 6 hours. RHC-measured mean PAP (mPAP) and systolic PAP (sPAP) using a standard fluid-filled catheter. An interventional cardiologist, blinded to the patients’ diagnoses and echocardiographic data, analyzed pressure tracings to evaluate pulmonary hemodynamics. Cardiac output and pulmonary blood flow were calculated using the standard Fick method and indexed to body surface area. Pulmonary artery compliance (mL/mm Hg/m 2 ) was calculated as the ratio of stroke volume (indexed pulmonary blood flow divided by heart rate) to pulmonary artery pulse pressure. PVR (Wood units [WU]) indexed to body surface area (PVRi; WU · m 2 ) was derived using pulmonary capillary wedge pressure from the following equation: (mPAP − pulmonary capillary wedge pressure)/indexed pulmonary blood flow. Oxygen consumption was assumed using the standard LaFarge methodology.
Echocardiography
We acquired two-dimensional and color Doppler echocardiographic images of the parasternal and apical standard views with the patient in the supine position per the American Society of Echocardiography guidelines. We developed a protocol for PAAT image acquisition and postprocessing data analysis ( Appendix 1 ) and tested for variability using reproducibility statistical analysis. A spectral Doppler image was obtained by placing a pulsed Doppler sample volume at the pulmonary valve annulus in the parasternal short-axis view ( Figure 1 ). Maximal alignment of Doppler interrogation with blood flow direction was achieved with the placement of the sample volume at the annulus of the pulmonary valve and not more proximally in the RV outflow tract. PAAT was calculated from a spectral Doppler envelope as the time interval between the onset of systolic pulmonary arterial flow (onset of ejection) and peak flow velocity ( Figure 1 ).
Confounding Influences
The variables confounding the PAAT measures include imaging acquisition techniques and postprocessing analysis, heart rate variability, influence of RV mechanics and function, and pulmonary compliance. We identified and quantified the image acquisition and postprocessing analysis uncertainties with the development of a protocol ( Appendix 1 ). To account for the potential impact of heart rate variability, PAAT was corrected for the R-R interval and also adjusted for RV ejection time (RVET) with the ratio of PAAT to RVET. The mean PAAT/RVET ratio from three cardiac cycles was used for data analysis and further corrected by the square root of the R-R interval. PAAT/RVET and the R-R interval were compared using a linear regression. We measured RV preejection time, RVET, and the ratio of RV preejection time to RVET to assess RV mechanics. Preejection time was defined as the interval between the onset of QRS complex to the onset of RV ejection, whereas RVET was defined as the interval between the onset of RV ejection to the cessation of systolic pulmonary arterial flow in the same cardiac cycle ( Figure 1 ).
We assessed RV function using two-dimensional speckle-tracking echocardiography–derived global longitudinal systolic strain, a sensitive and reliable measure with published normative values in children. One sonographer measured RV global longitudinal strain in the children who underwent simultaneous DE and RHC using a protocol previously validated by our group and others.
PAAT-Based Regression Equations and Cutoff Values
In the derivation cohort, PAAT and PAAT/RVET were compared with RHC-derived mPAP, sPAP, PVR, and PVRi using both linear and logarithmic regression analysis ( P values < .01 were considered to indicate statistical significance). A receiver operating characteristic (ROC) curve was constructed to determine cutoff valueS for PAAT and PAAT/RVET with the best sensitivity to predict pulmonary hypertension, defined as elevated PVR values (PVR > 2 WU and PVRi > 3 WU · m 2 ), elevated sPAP (>30 mm Hg), and mPAP > 25 mm Hg. The statistical analysis was performed using SPSS version 14.0 (SPSS, Chicago, IL).
Validation Cohort
The regression equations using PAAT and PAAT/RVET that were generated in the derivation cohort to predict PAP and PVR were subsequently validated in two separate validation subcohorts: (1) 25 age- and gender-matched pediatric patients with a wide range of pulmonary hemodynamics who were prospectively recruited and underwent simultaneous RHC and DE between January 2014 and December 2014 and (2) 25 age- and gender-matched pediatric patients with pulmonary arterial hypertension who underwent DE and RHC within 7 days of each other identified from a retrospective search of the database for the period between November 2011 and December 2013. We included this retrospective validation subcohort to demonstrate the applicability of the PAAT-based regression equations in routine clinical practice under no sedation. We assessed the predictive value of the PAAT-based regression equations to estimate PAP and PVR in both validation subcohorts by comparing with the RHC-derived pulmonary hemodynamics using (1) Bland-Altman plot analysis (percentage bias and 95% limits of agreement), (2) intraclass correlation, and (3) coefficient of variation. Bias < 10%, narrow 95% limits of agreement, a coefficient of variation <10%, and an intraclass correlation coefficient >0.8 were recognized as measures of minimal variability and positive reliability.
Reproducibility Analysis
Reproducibility of PAAT measurements was assessed using intra- and interobserver variability in 60% of the images from the derivation and validation cohorts. Three observers (P.T.L., M.D.P., and G.K.S.) performed offline analysis using the same measurement protocol and were blinded to the patients’ diagnoses, RHC data, and one another ( Appendix 1 ).
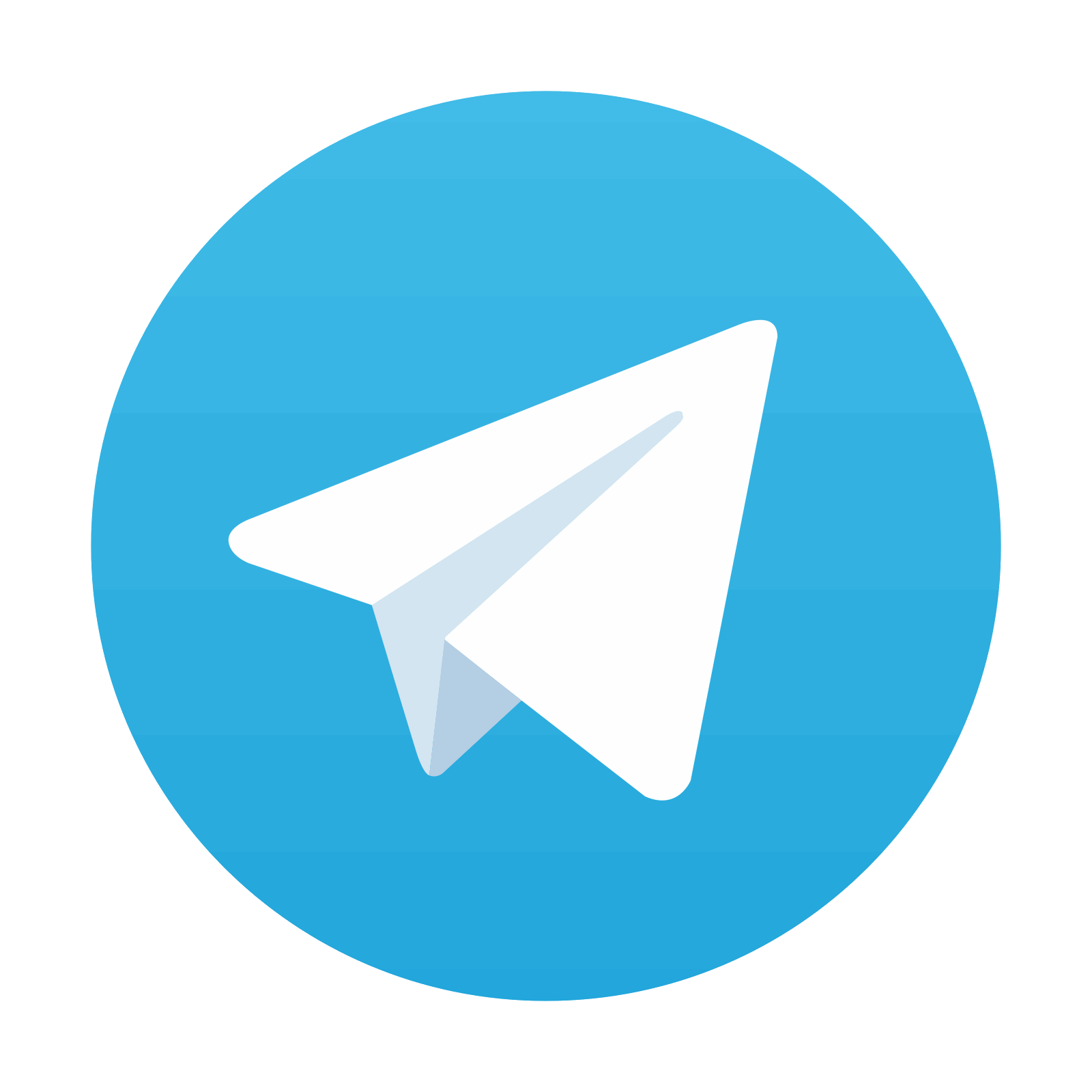
Stay updated, free articles. Join our Telegram channel

Full access? Get Clinical Tree
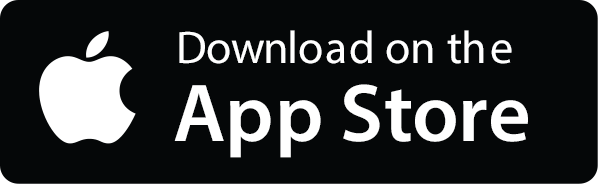
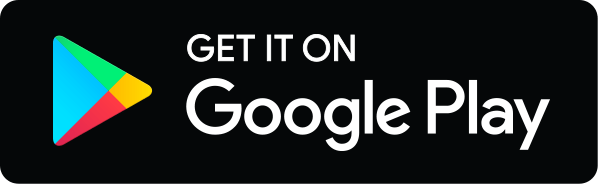