Summary
Pulmonary arterial hypertension is a progressive syndrome based on diverse aetiologies, which is characterized by a persistent increase in pulmonary vascular resistance and overload of the right ventricle, leading to heart failure and death. Currently, none of the available treatments is able to cure pulmonary arterial hypertension; additional research is therefore needed to unravel the associated pathophysiological mechanisms. This review summarizes current knowledge related to this disorder, and the several experimental animal models that can mimic pulmonary arterial hypertension and are available for translational research.
Résumé
L’hypertension artérielle pulmonaire (HTAP) est un syndrome progressif caractérisé par une augmentation persistante de la résistance vasculaire pulmonaire et par une surcharge du ventricule droit, ce qui conduit à une insuffisance cardiaque et la mort. À l’heure actuelle, l’HTAP est incurable et, par conséquent, davantage de recherche est nécessaire pour comprendre les mécanismes physiopathologiques associés. Cette revue résume les connaissances actuelles liées à ce trouble et les différents modèles animaux de l’HTAP disponibles pour la recherche translationnelle.
Background
Pulmonary arterial hypertension (PAH) is a syndrome based on diverse aetiologies and pathogenesis, potentially leading to right heart failure and death. PAH is characterized by excessive pulmonary vascular remodelling, pulmonary arterial obstruction and elevated pulmonary vascular resistance (PVR), which result in a marked increase in right ventricle (RV) afterload. Eventually, the RV is unable to cope with the increase in load and heart failure develops .
PAH is defined by a mean pulmonary artery pressure (mPAP) ≥ 25 mmHg at rest, and is haemodynamically characterized by the presence of precapillary pulmonary hypertension (PH), which implies a normal pulmonary capillary wedge pressure or left ventricular end-diastolic pressure ≤ 15 mmHg, with a PVR > 3 Wood Units . So far, there is insufficient evidence to add an exercise criterion to this definition .
Pathophysiology
Histopathology
PAH is considered a vasculopathy and, in general, all PAH subgroups (i.e. idiopathic, heritable, drug or toxin-induced, or associated with connective tissue disease, human immunodeficiency virus [HIV] infection, portal hypertension, congenital heart disease or schistosomiasis) and other forms of PH (i.e. PH resulting from lung disease and/or hypoxia) exhibit several arterial abnormalities that are mainly present in the small pulmonary arteries and arterioles . The most common pathological features in PH are medial hypertrophy, local dilation and intimal atheromas, and because they are present in all forms of PH, they are of poor diagnostic value. However, PAH is characterized by constrictive lesions, which include medial hypertrophy and intimal and adventitial thickening, and by complex lesions that include plexiform and dilation lesions, as well as arteritis .
Medial hypertrophy is defined by an increase in the diameter of the medial layer, measured between the internal and external elastic lamina, exceeding 10% of the cross-sectional diameter of the arteries. This abnormality appears in all PAH subgroups, and occurs as a result of pulmonary arterial smooth muscle cell (PASMC) proliferation and/or recruitment to the tunica media. This lesion is considered an early event in PAH pathogenesis, but it is usually regarded as reversible . Intimal and adventitial thickening occurs as a result of the proliferation and recruitment of connective tissue cells and, consequently, by the interstitial deposition of collagen, leading to fibrosis. This thickening can be uniform (concentric) or focal (eccentric); the former is often associated with thrombotic events . The presence of plexiform lesions in the vascular compartments is very characteristic of PAH , and is a consequence of local and excessive pulmonary arterial endothelial cell proliferation, which leads to the formation of capillary-like channels within the arterial lumen . These lesions are responsible for the expansion and destruction of the arterial wall, as they tend to enlarge into the perivascular space. Fibrin, thrombi and platelets are frequently encountered in these lesions, as well as dilation lesions, which are thin-walled vein-like vessels that are a potential cause of haemorrhages and subsequent fibrosis. The artery wall may also accumulate necrotic and fibrotic tissue and/or be infiltrated with inflammatory cells, leading to arteritis .
Cellular factors
The main mechanisms responsible for pulmonary vascular dysfunction are the abnormal proliferation of PASMCs and pulmonary arterial endothelial cells, infiltration of inflammatory cells and fibrosis . However, PAH is not only associated with cell proliferation, but also with apoptotic processes, as the imbalance between these two events is the major cause of the narrowing of the pulmonary arteries in PAH .
All forms of PAH have in common the migration and proliferation of PASMCs, which in general is accompanied by the migration of fibroblasts and the formation of an extracellular matrix layer. The uncontrolled proliferation of PASMCs ultimately leads to medial hypertrophy, also contributing to the thickening of the intima and adventitia layers of the pulmonary vessels . The formation of an extracellular matrix and myofibroblasts between the endothelium and internal elastic lamina is termed neointima. Another feature characteristic of PAH is the increase in vasa vasorum neovascularization, which mainly affects the adventitia, and can expand to the media .
Beyond the intrinsic dysfunction present in both pulmonary arterial endothelial cells and PASMCs in PAH, recent evidence suggests that crosstalk between these cells may contribute to further impairment. Eddahibi et al. demonstrated that pulmonary arterial endothelial cells constitutively produce and release growth factors that act on PASMCs, which appears to be critical for pulmonary vascular remodelling . Although the majority of mechanisms governing this crosstalk remain to be elucidated, Humbert et al. have discussed whether some of the pathways already known to be involved in PAH pathophysiology are also involved in the crosstalk between pulmonary arterial endothelial cells and PASMCs .
In response to growth factors, pulmonary arterial endothelial cell and PASMC can grow, proliferate, migrate or differentiate, and therefore these substances assume a very important role in vascular remodelling processes. One of the most studied growth factors in the scope of PAH is vascular endothelial growth factor, which has been identified as an important mediator in the hypoxia-inducible factor-1α signalling pathway under hypoxic conditions, which eventually culminates in endothelial proliferation and the formation of new blood vessels. Another growth factor that also regulates blood vessel development is fibroblast growth factor-2, which, like vascular endothelial growth factor, is also able to promote the formation of new blood vessels in the presence of low levels of oxygen. Furthermore, platelet-derived growth factor and epidermal growth factor have also been implicated in PAH pathophysiology because of their ability to stimulate PASMC proliferation and survival, contributing to the abnormal PASMC phenotype observed in the distal pulmonary arteries of PAH patients . In PAH, if the pulmonary endothelium becomes damaged, these vascular growth factors mentioned above may mediate an exaggerated or persistent response to injury, leading to new vessels or hyperproliferation of the endothelium.
In response to shear stress, hypoxia, inflammation and/or other stimuli, endothelial cells proliferate excessively and generate plexiform lesions . In response to these stimuli, endothelial cells develop an imbalance between proliferative and apoptotic processes, as well as changes at the functional level. Endothelial dysfunction eventually results in a clear imbalance between the production and release of vasoconstrictors/vasodilators, activator/inhibitory growth factors, prothrombotic/antithrombotic mediators and proinflammatory/anti-inflammatory signals .
In certain forms of PAH (i.e. PAH associated with autoimmune diseases), the inflammatory response plays an important role, as some patients improved both clinically and haemodynamically when administered with immunosuppressant therapy. Indeed, several markers of inflammation, such as interleukin-1b, interleukin-6 and tumour necrosis factor-α, are highly expressed in both PAH patients and animal models , while demonstrating a positive correlation with disease severity and poor outcome. At high concentrations, these cytokines may contribute to the exaggerated contractility and proliferation of vascular cells, thereby promoting the adverse remodelling seen in PAH . Moreover, some inflammatory cells, such as lymphocytes and macrophages, can also be found in plexiform lesions .
Some PAH patients exhibit elevated plasma concentrations of fibrinopeptides, along with von-Willebrand factor and plasminogen activator inhibitor type 1, reflecting an abnormal coagulation process and endothelial dysfunction, respectively. Both events are very important in PAH development because they can generate or aggravate in situ thrombosis. Platelets also participate in vasoconstriction and vascular remodelling, as they are able to produce prothrombotic, vasoactive and mitogenic factors .
Molecular abnormalities and therapeutic targets
The molecular abnormalities seen in PAH patients are normally associated with increased endothelin (ET)-1 concentrations and decreased nitric oxide (NO) and prostacyclin concentrations, as these factors influence vascular homeostasis, cell survival and proliferation, among other processes, and are key therapeutic targets for the management of PAH.
Prostanoids
Prostacyclin (or prostaglandin I2) and thromboxane A 2 belong to the prostanoid family, and are produced from arachidonic acid metabolites. The former is a potent vasodilator and inhibitor of platelet activation, while thromboxane A 2 has opposite effects . Prostacyclin is produced in vascular endothelial cells, and acts on both systemic and pulmonary vascular smooth muscle cells, as well as on circulating platelets and other cells, via the cyclic adenosine monophosphate pathway . Prostacyclin plays an important role in antiproliferative, antithrombotic, antimitogenic and immunomodulatory activity . In PAH patients, the expression of prostacyclin synthase in the pulmonary arteries is reduced, and therefore the production of prostacyclin in endothelial cells is decreased . In fact, patients with PAH have reduced endogenous prostacyclin . Therefore, as shown in Fig. 1 , prostacyclin analogues are an established PAH therapy, which mimic the prostacyclin signalling pathway and are able to induce vasodilation while inhibiting platelet activation .

Endothelin-1
This 21-amino acid vasoactive peptide is expressed in several mammalian tissues in different types of cells, and is responsible for the regulation of vascular tone. ET-1 exerts its effects through interaction with two types of receptors: ET receptor type A (ET A ) and ET receptor type B (ET B ), which belong to the G-protein-coupled receptor family. In PASMCs, when activated, both receptors have a vasoconstrictor effect, while in pulmonary arterial endothelial cells, ET A is not expressed, and the activation of ET B leads to vasodilatation . Endothelial cell dysfunction usually leads to ET-1 overexpression, which results in vasoconstriction and reduced synthesis of NO and prostacyclin, worsening the vasoconstrictor response. The upregulation of ET-1 is also involved in inflammatory responses and increased fibrosis. In PAH patients, ET-1 clearance by the pulmonary vasculature is reduced, and ET-1 plasma concentrations are elevated and correlate with PAH severity and prognosis . Therefore, ET receptor antagonists are used as treatment for PAH ( Fig. 2 ). So far, several types of ET receptor antagonists have been identified, which differ in their selectivity for ET A and ET B receptors . Therapies targeting this pathway can be dual, if they block both receptors, or selective if they only block ET A .

Endothelial nitric oxide
NO is a 30 Da lipophilic gaseous molecule that can be synthesized in mammalian tissues via activation of one of the three NO synthase isoforms, which have the ability to catalyse the formation of NO from L-arginine in a two-step reaction. NO is a vasodilator that modulates several physiological processes, and is also capable of inhibiting leukocyte adhesion, platelet aggregation, thrombus formation and vascular proliferation . Endothelial NO synthase can be activated either by G-protein-coupled receptor signal transduction, which increases intracellular calcium concentrations and, subsequently, concentrations of calcium-calmodulin, Akt signalling, vascular endothelial growth factor and hormonal stimuli (e.g. oestrogen and insulin) . In both animal models and humans with PH, decreased pulmonary vascular endothelial NO synthase activity is observed, along with loss of NO bioavailability, which is linked to impaired endothelium-dependent and -independent vasodilatation, increased PASMC mitogenesis and platelet aggregation .
Soluble guanylate cyclase stimulators were recently approved for the treatment of PAH, as they are able to restore NO levels (which are decreased in PAH) and the associated beneficial properties , such as vasodilatation and reduced proliferation ( Fig. 3 ).

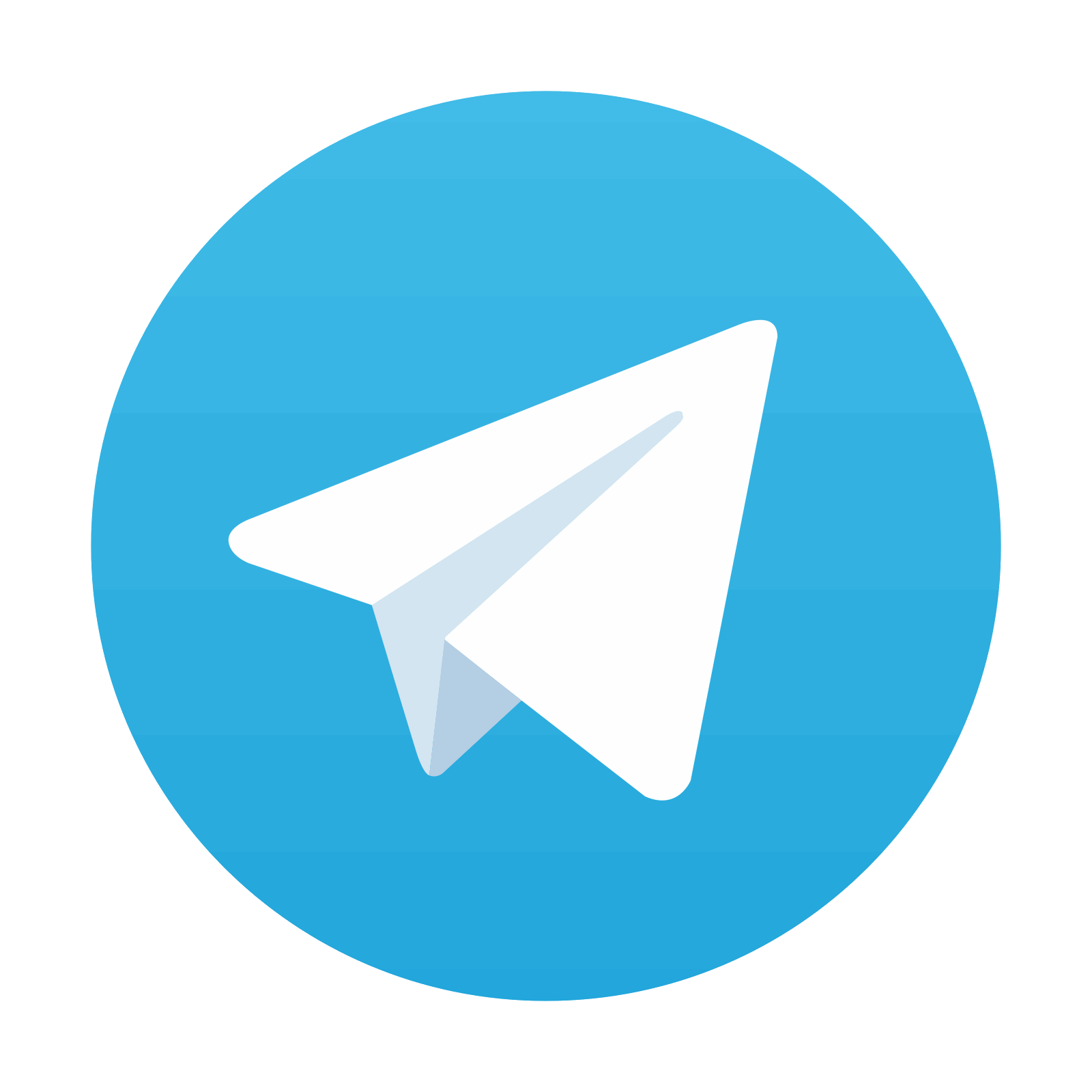
Stay updated, free articles. Join our Telegram channel

Full access? Get Clinical Tree
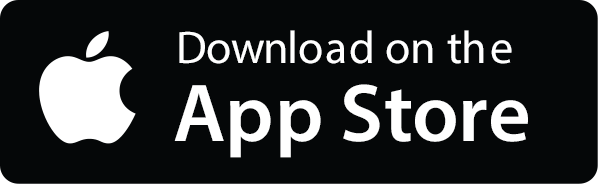
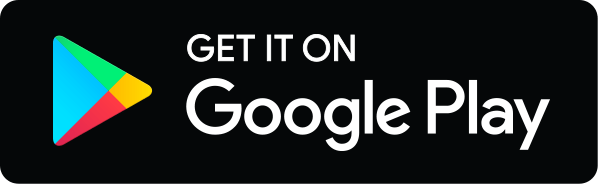
