Fig. 16.1
This schematic places pulmonary vascular cell apoptosis, in particular endothelial cell apoptosis, in the center of the lung vascular remodeling process which results in exuberant wound healing. Autophagy is portrayed as a mechanism to protect against apoptosis. Efferocytosis, the removal of apoptotic bodies after engulfment by professional phagocytes or neighboring cells, if inhibited or ineffective, prolongs inflammation and sets the stage for autoimmunity. Chronic hypoxia activates via HIF 1 alpha inflammatory mechanisms, and via VEGF, mobilizes precursor cells from the bone marrow. Within the injured vascular wall, vascular niche precursor cells divide and give rise to cells which obliterate the lumen [With permission from Voelkel (2013)]
Because of the reported phenotype switch of exuberantly proliferating pulmonary vascular endothelial cells in severe PAH (Cool et al. 1999; Tuder et al. 1994; Tu et al. 2011), we had hypothetically applied the concept of the cancer paradigm as outlined by Hanahan and Weinberg (2011) in order to explain the roles of “misguided angiogenesis” and the evolution of quasi-malignant, that is, apoptosis-resistant, proliferating endothelial cell phenotypes in the pathobiology of severe PAH (Voelkel et al. 2012; Rai et al. 2008).
In the following chapter, we will examine a role for stem cells and precursor cells (Majka et al. 2005; Chow et al. 2013; Spees et al. 2008) in the development of exuberant pulmonary vascular endothelial cell growth (Tuder et al. 1994) borrowing from models of wound healing and from cancer stem cell concepts. We will focus on stem cell differentiation and its potentially critical role in the development and maintenance of severe angio-obliterative PAH and will advance the argument for a stem cell-directed therapy of PAH.
16.2 Evidence for a Participation of Stem and Precursor Cells in Severe PAH
Remarkably, several recent reviews covering the topic of the pathology (Stacher et al. 2012) or pathobiology of severe PAH (Rabinovitch 2012; Tuder et al. 2013) have not anticipated or considered a mechanistic role of precursor or stem cells. This is surprising in view of the amount of clinical and experimental data which demonstrate that circulating precursor cells and vessel precursor cells have been described in various forms of PAH (Table 16.1). The majority of these studies report that such cells are present in the blood or in the lungs; however, evidence based on strict mechanistic criteria of stemness is largely lacking. Nevertheless, at this moment in time it is not premature to sketch a stem cell picture of hypertensive pulmonary vascular remodeling and highlight likely mechanistically involved bone marrow-derived and resident lung precursor and stem cells.
Table 16.1
Precursor and stem cells in pulmonary hypertension
Circulating precursor cells in human disease | |
Increased endothelial cells in PAH patients | Bull et al. (2003) |
Increased bone marrow-derived CD34+/CD133+ cells in IPAH | Aosingh et al. (2008) |
Increased CD34+/CD133+ cells in PAH patients | Smadja et al. (2010) |
Increased CD34+/CD133+, CD34+/KDR+, CD34+/CD133+/KDR+ cells in PAH | Diller et al. (2008) |
Lung tissue precursor cells | |
c-Kit+ cells | |
In plexiform lesions from IPAH patients | |
Mesenchymal precursor cells | |
In thrombendarterectomized tissue | Firth et al. (2010a) |
Endothelial cell precursor cells | |
In thrombendarterectomized tissue | Yao et al. (2009) |
In pulmonary arteries from PAH patients | Farha et al. (2011) |
Oct-4+ cells | |
In vascular smooth muscle cells from patients with IPAH | Firth et al. (2010b) |
CD44+ multipotent stem cells in plexiform lesions in IPAH | Ohta-Ogo et al.(2012) |
Precursor cells in animal models of PAH | |
Circulating precursor cells | |
Decrease in endothelial cell precursors in rat monocrotaline model | Liu et al. (2013) |
Lung tissue precursor cells | |
Perivascular accumulation of c-kit+ cells in hypoxic mice | |
Increased c-kit expression in endothelial and vascular | Farkas et al. (2014) |
Smooth muscle cells in the rat Su/Hx model | |
Increased number of c-kit+ cells in hypoxic calves | Davie et al. (2004) |
Mesenchymal precursors in hypoxic calves | Frid et al. (2006) |
We may be biased but believe that the first statement which could be interpreted as a veiled reference to precursor cells in PAH can be found in a study describing the electron microscopic features of plexiform lesions (Smith and Heath 1979) as elaborated in (Tuder et al. 1994): “the lesions appeared to contain cells of an undetermined nature” and the authors go on to suggest that “these cells might represent primitive angioformative cells committed to differentiate into endothelial cells” (Smith and Heath 1979). The term “angioformative” was later replaced with “angiogenic,” and the cells that had been described as “committed”, i.e. not differentiated, are large and have plump nuclei and prominent nucleoli (Fig. 16.2).
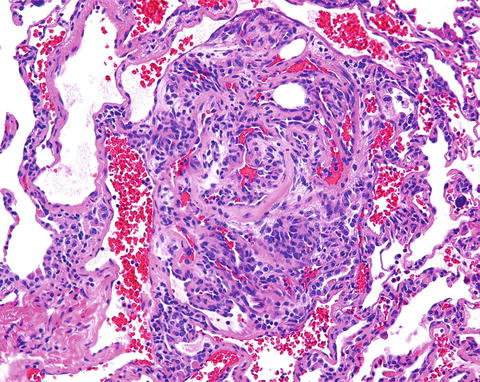
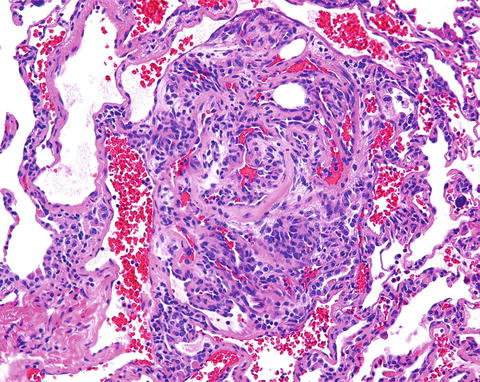
Fig. 16.2
Complex pulmonary vascular lesion in the lung from a patient with severe pulmonary arterial hypertension. The vessel lumen is obliterated by phenotypically altered cells. The cells are large, plump, and have large nuclei and nucleoli. There are many lumina within this lesion
Years later, circulating precursor cells were identified in the blood from patients with severe PAH. Bull et al. were the first to describe circulating CD133+ cells and to speculate that these cells might be derived from the PAH patients’ bone marrow (Bull et al. 2003). While the original goal of this particular study had been to identify precursor cells which were thought to be released from the “sick lung circulation” (Ghofrani et al. 2010) and can perhaps be used as surrogates for the abnormal, phenotypically altered lung vessel endothelial cells (Cool et al. 1999; Rai et al. 2008), at the time, only a limited number of cell membrane protein markers was known and available for precursor cell characterization.
16.3 The Cancer Paradigm of PAH
It is now clear and accepted that PAH is not one homogeneous disease, but that the term rather defines a group of disorders which share a severe pulmonary microangiopathy that is usually progressive and driven by proliferation of apoptosis-resistant vascular cells. This definition does not contradict the presently used WHO nomenclature, but it emphasizes “severe and angio-obliterative” disease features. This microangiopathy defines histologically the abnormal appearance and function of the precapillary arterioles in the lungs of patients with systemic sclerosis, the various forms of hereditary IPAH, HIV infection, and congenital intracardiac shunts. Several, not necessarily mechanistically related, factors and descriptors of PAH prompted us to consider the comparison with malignancies (Hanahan and Weinberg 2011).
First, like many cancers, IPAH is refractory to therapy; that is the vascular lesions do not regress with the treatment of PH by standard pulmonary hypertension drugs or after chemotherapy in the few cases where this has been attempted, as recently documented by the PAH imatinib treatment trial (Ghofrani et al. 2010).
Second, there are several reports of patients developing PAH years after radiation and chemotherapy for Hodgkin’s lymphoma, and it is well-appreciated that patients suffering from myelodysplastic disorders can develop PAH (Dingli et al. 2001). However, it remains unclear whether the late occurrence of severe PAH following therapy for Hodgkin’s lymphoma is related to the lymphoma or to the chemotherapy. Perhaps the recent occurrence of PAH following treatment of leukemia with dasatinib (Groeneveldt et al. 2013) may suggest a chemotherapy-related mechanism.
In PAH, the complex vascular lesions, like many tumors, are multicellular and the cells are characterized by various degrees of differentiation and proliferation and, again like tumors which contain cancer stem cells, these lesions contain stem cells (Fig. 16.3). Cancer stem cells were initially implicated in hematopoietic malignancies and later also identified in solid tumors (Hanahan and Weinberg 2011). Some of the plexiform lesions in idiopathic PAH are composed of clonally expanded endothelial cells (Lee et al. 1998), which carry mutations (Yeager et al. 2001). In tumors, normal tissue stem cells may undergo oncogenic transformation or progenitor cells may undergo this transformation (Hanahan and Weinberg 2011). Signals that trigger endothelial cell mesenchymal transformation (EnMT) can be released by inflammatory stroma and may also be implicated in stem cell formation and maintenance (Hanahan and Weinberg 2011). Hanahan and Weinberg in their 2011 review of “The Hallmarks of Cancer” describe solid tumors as an assemblage of cells (Hanahan and Weinberg 2011), which contain stem cells, fibroblasts, endothelial cells, immune/inflammatory cells, and pericytes. All of these cell types, prominently including apoptosis-resistant cells, are also found in the vascular lesions of patients with severe PAH. However, one important difference between malignant tumors and the pulmonary vascular lesions in severe PAH is the non-invasiveness of the pulmonary vascular lesions; the lesions do not break through the vessel wall and do not invade alveolar spaces.
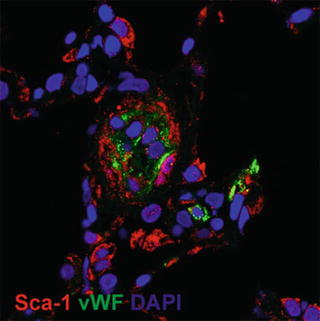
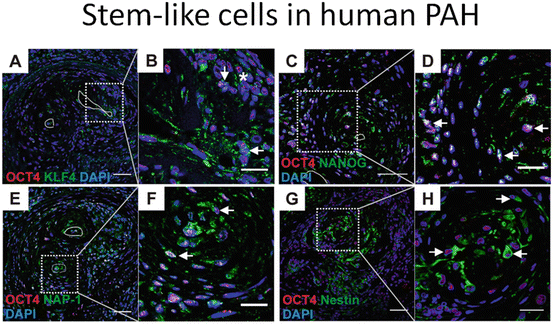
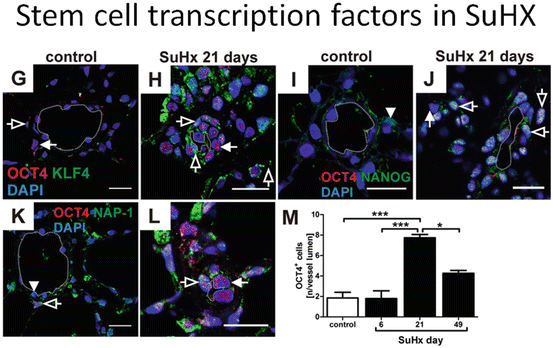
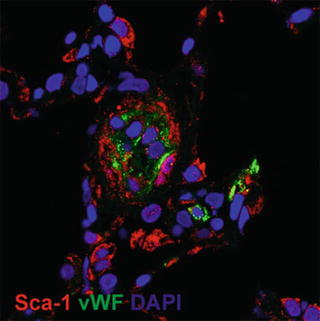
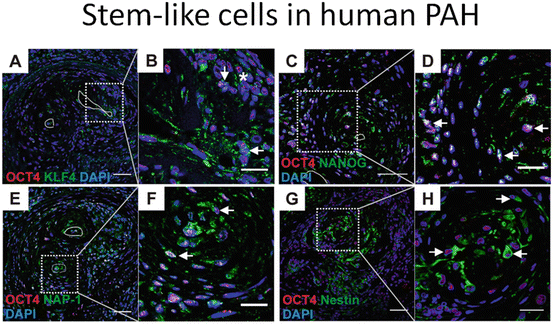
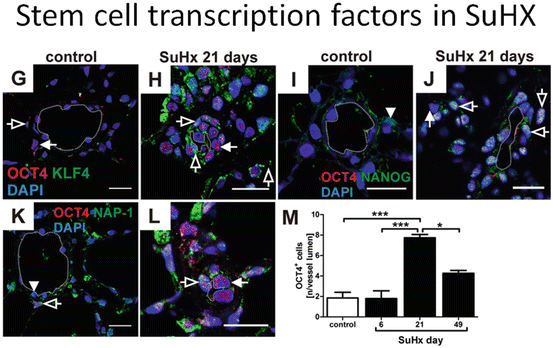
Fig. 16.3
Immunohistochemistry of vascular lesions in a rat lung which develop after a single subcutaneous injection of the VEGF receptor blocker Sugen 5416 when combined with 3 weeks of chronic hypoxia. There are multiple Sca+ cells (a) and cells staining positive for the stem cell markers Oct4, Nanog, KLF4, and the marker protein NAP 1 (nucleosome assembly protein 1, a histone chaperone) (b). Immunohistochemistry of a human plexiform lesion staining positive for the markers Oct4, Nanog, Nestin, and KLF4 (c)
16.4 Vascular Stem Cell Niches
Due to the fact that in patients with angioproliferative PAH, there is evidence for circulating, bone marrow-derived precursor cells (see above), and because precursors are found in the vessel wall and in the perivascular spaces, we assume for the purpose of this review that both bone marrow-derived and resident vascular precursor cells participate in the process of pulmonary vascular remodeling (Voelkel et al. 2012; Rai et al. 2008).
There is an increasing number of reports concerned with vascular biology that describe several different precursor and stem cells residing in the vessel wall (Ergun et al. 2011; Pasquinelli et al. 2007; Ingram et al. 2005; Tigges et al. 2013; Majesky et al. 2012; Klein et al. 2011; Zengin et al. 2006). As pointed out above, in human PAH investigations the presence of precursor and stem-like cells has been documented by several groups; however, short of lineage-tracing studies, which cannot be performed in patients, it will be difficult to prove that these cells actually function as stem cells, or to demonstrate differentiation or de-differentiation. We are not aware of lineage-tracing studies in any animal model of PAH conducted to demonstrate which of the different precursor cells divide, give rise to apoptosis-resistant cells, and participate in the process of pulmonary vascular remodeling. However, it is likely that in PAH the adventitial stem cell niche is a reservoir for precursor cells, as has already been shown in systemic vessels. Rare chondrocytes and adipocytes have been found in the complex vascular lesions from patients with IPAH (Farkas et al. 2013), suggesting the presence of pluri- or multi-potent precursors in these lesions. Of particular interest are pericytes in the adventitia of the remodeled lung vessels (Ergun et al. 2011; Klein et al. 2011).
Klein et al. (2011) showed that CD44+ multipotent stem cells, which are present in plexiform lesions (Ohta-Ogo et al. 2012), can differentiate into pericytes and smooth muscle cells, and that Hox genes are involved in the differentiation into smooth muscle cells. Interestingly, Golpon et al. had shown an altered expression pattern of Hox genes in lungs from idiopathic PAH patients (Golpon et al. 2001); strikingly, the differentially expressed HoxC6 and HoxC8 genes in IPAH lungs are also differentially expressed in the multipotent vessel wall CD44+ stem cells (Klein et al. 2011) and also in cancers (Moon et al. 2012; Du et al. 2014).
There are different subsets of pericytes which can be morphologically distinguished. Lung vessel pericytes can transdifferentiate into contractile smooth muscle cells under chronic hypoxic conditions (Meyrick et al. 1981). Pericytes express NG2, PDGF receptor beta, desmin, and CD146; they can activate ADAMTS1 which cleaves the vessel basement membrane, such that pericytes can directly interact with endothelial cells. Pericytes can express a repertoire of functional phenotypes as shown by culture studies of human pluripotent stem cells (Wanjare et al. 2014); they also synthesize and release VEGF, yet little is known about a precursor role of pericytes in angioproliferative PAH.
As has been pointed out by Ingram et al. (2005), the pulmonary small vessel endothelial cell layer is probably another vessel wall stem cell reservoir or niche, and we speculate that resident endothelial stem cells may account for the EnMT (endothelial cell mesenchymal transition (Medici and Kalluri 2012; Rokavec et al. 2014)) that contributes to hemodynamic stress-induced muscularization of pulmonary arterioles and also to the proliferation of phenotypically altered intima layer cells (Cool et al. 1999).
16.5 The Sugen 5416/Hypoxia Rat Model of Severe Angioproliferative PAH
This rat model of severe PAH was first reported in 2001 (Taraseveciene-Stewart et al. 2001). Briefly, rats are subcutaneously injected with a single dose of the VEGF receptor blocker Sugen 5416 and then exposed to chronic hypoxia for 4 weeks (Taraseveciene-Stewart et al. 2002). After this 4-week period of exposure to hypoxia, the animals are removed from the hypoxic environment and they continue to present with severe angio-obliterative PAH and right heart failure (Oka et al. 2007).
This model is of interest, because the wild-type Sprague Dawley rats used for these experiments do not carry any mutations and the developed disease is refractory to treatment of the animals with pulmonary vasodilator drugs and other drugs (Taraseveciene-Stewart et al. 2006). However, and of mechanistic importance, the disease can be prevented by the inhibition of apoptosis using a pan caspase inhibitor (Taraseveciene-Stewart et al. 2001, 2002). This model also demonstrated for the first time that the degree of pulmonary hypertension was highly correlated with the number of obliterated lung vessels (Oka et al. 2007).
Variants of this model are the combination of Sugen 5416 (VEGFR blockade) and left-sided pneumonectomy, Sugen 5416 treatment of athymic immunocompromised rats, or the immunization of rats with ovalbumin combined with VEGFR blockade. Each of these combinations produces severe and frequently fatal angio-obliterative PAH (Nicolls et al. 2012). Thus, “two hits” are required in this model because Sugen 5416 by itself generates pulmonary emphysema and only a mild degree of PAH (Kasahara et al. 2000). We have recently attempted to elucidate this apparent paradox: that an antiangiogenic drug in combination with a second challenge causes angio-obliterative PAH (Gomez-Arroyo and Voelkel 2014). As shown by Sakao et al. (2007), induction of lung endothelial cell apoptosis by (Sugen 5416-induced) VEGFR blockade is followed by the emergence of apoptosis-resistant cells, and importantly, a fraction of the initial apoptosis surviving cells are precursor or stem cells. This finding has been recently confirmed by Das et al. (2014); the Sugen 5416/hypoxia rat model of severe PAH is now being interrogated to assess the contribution of precursor/stem cells to pulmonary vascular remodeling (Farkas et al. 2013; Das et al. 2014).
Immunohistochemistry of lung tissue samples from Sugen/chronic hypoxia (Su/Hx) rats with established severe PAH demonstrates the expression of Oct-4, KLF-4, NANOG, Nestin, Ly6A (Sca-1), and c-kit proteins in vascular lesion cells and in the cells occupying the perivascular spaces (Figs. 16.3a, b). Similar aggregates can be shown in the complex pulmonary vascular lesions of patients with severe angio-obliterative PAH (Fig. 16.3c). In the aggregate, these findings suggest that cells expressing precursor and stem cell markers are present in the pulmonary vascular lesion cells. As already pointed out, the presence of cells expressing stem cell markers does not necessarily imply that these cells actually function as stem cells. However, in the context of a vascular stem cell niche and borrowing heavily from David Scadden’s “Neighborhood” concept of stem cell niches (Scadden 2014), it is reasonable to consider a stem cell-centric, or stem cell-anchored hypothesis of severe PAH vascular remodeling.
16.6 The Role of the Vascular Stem Cell Niche
For most known multicellular organisms, their relatively constant outward appearance is underscored by an incessant inner transformation in which cells lost to normal wear and tear (turnover) are replaced by the progeny of dividing cells. If the normal fate of cells in adult tissues is plastic and environmental changes such as growth-promoting conditions can switch their development from a program of terminal differentiation to one of active clonogenicity, we need to invest more effort in studying how stemness arises (Sanchez Alvarado and Yamanaka 2014).
In the following we will not depart from the quasi-malignant neoplastic concept of the pathobiology of severe angio-obliterative PAH, but we will redefine this concept by introducing the complementary hypothesis that progenitor/stem-like cells, which are present in the normal vascular wall (Ergun et al. 2011; Pasquinelli et al. 2007; Ingram et al. 2005; Tigges et al. 2013; Majesky et al. 2012; Klein et al. 2011; Zengin et al. 2006), proliferate and transdifferentiate, as the vessel wall responds to vascular injury. The concept of a homeostatic lung vessel structure maintenance program (Taraseveciene-Stewart and Voelkel 2008) accommodates the postulate that in severe PAH, as part of the abnormal wound healing process, the first law of vascular biology, “the law of the endothelial cell monolayer,” is being broken (Rai et al. 2008). Thus, if we think about the pathobiology of severe PAH as driven by a wound-healing process (cancer is the wound that never heals) carried out in a cancer-like stem cell niche, we arrive at a concept of innate cell plasticity of the pulmonary vessel wall. For short, in severe PAH, some vessel wall cells, when exposed to severe stress, change their phenotype, and the stress may induce stemness in normally present multipotent progenitors (Sanchez Alvarado and Yamanaka 2014).
Such a conceptual model of the pathobiology of severe angioproliferative PAH would explain why endothelial cells can transdifferentiate into smooth muscle cells (Sakao et al. 2007; Frid et al. 2002) (see above), why pericytes may influence the growth of endothelial cells, and why cobblestone (flat) endothelial monolayer cells change their phenotype and turn into large cells that cluster and proliferate (Cool et al. 1999; Tuder et al. 1994; Rai et al. 2008). Such a disease model would also point out the limits of single cell type culture studies and focus our attention on the investigation of multi-cellular niches, where neighboring cells exchange information with the goal of maintaining structural and functional homeostasis. The biological principle of homeostasis and the response to the stress- or injury-induced evolutionary selection pressure, apparently require cellular plasticity.
Apparently, there are hierarchical signaling pathways and topographical blueprints which organize the ongoing conversation between dying and growing cells in all three layers of the vascular wall (Sanchez Alvarado and Yamanaka 2014). It is somewhat intuitive, and this concept suggests how difficult it will be to “reprogram” the altered vessel wall and perivascular cells in the setting of angio-obliterative PAH, to de-remodel by differentiation, in particular when the hemodynamic stress (high pressure or high flow) persists. While in the rodent models of chronic hypoxia-induced PAH there occurs generally successful de-remodeling after several weeks after the chronic hypoxic stress has been withdrawn, this is not the case in the Sugen/hypoxia rat model (see above). In the Sugen/hypoxia model of severe angio-obliterative PAH simvastatin treatment caused a partial reduction of the PAH (Taraseveciene-Stewart et al. 2006), and treatment of rats with established angio-obliterative PAH with a copper chelator reopened most of the obliterated vessels without affecting the muscularization of the pulmonary arterioles. Unfortunately, this Cu2+-chelator-induced de-remodeling is transient as the vessel obliteration returns once the copper chelator treatment has been discontinued (Bogaard et al. 2012). Treatment of the rats with the copper chelator not only reopened vessels and reduced the pulmonary hypertension, but also the number of c-kit + cells in and around the diseased arterioles. If we postulate that progenitor/stem cells drive angioproliferative PAH in the Sugen/hypoxia model, the antiangiogenic copper chelator may have caused pulmonary arteriolar remodeling by influencing the behavior of stem cells, or their crosstalk with the injured vessel wall cells.
There are presently only a few publications which support the concept that copper chelation affects stem cell behavior (Zaker et al. 2013; Jain et al. 2013; Suh et al. 2014). Interestingly, copper chelation does reduce the number of circulating endothelial progenitor cells in women with breast cancer (Jain et al. 2013) and copper chelation-affected neuronal differentiation in induced pluripotent stem cells (iPSC) (Suh et al. 2014).
16.7 The Role of Hypoxia
Chronic hypoxia in rodents causes PAH typically associated with muscularization of the pulmonary arterioles, and this form of vascular remodeling is generally reversible (de Raaf et al. 2014). The putative role of stem cells in this mechanism of hypoxia-induced remodeling is being investigated (see Table 16.1). Because Sugen 5416 alone does not cause significant PAH, we wonder whether chronic hypoxia awakens multilineage differentiating stress enduring (Muse) cells; such mesenchymal Muse cells express Oct-4, Nanog, Sox2, and also CXCL2, a chemokine receptor involved in cell homing (Heneidi et al. 2013).
A growing body of data indicates that most human cancers are hypoxic and that hypoxia-inducible factors (HIFs) aid in the acquisition of more malignant phenotypes of cancer-initiating cells (Mimeault and Batra 2013). For example, it has been suggested that the hypoxic tumor microenvironment maintains glioblastoma stem cells and promotes reprogramming towards a cancer stem cell phenotype (Heddleston et al. 2009). During severe chronic hypoxia, micro-regions of the lung may become severely oxygen-deprived and ischemia-reperfusion may occur (Voelkel et al. 2013); however, exuberant endothelial cell proliferation (Tuder et al. 1994) may not occur without substantial damage to the pulmonary vascular endothelium, as indeed is the case in the Sugen/hypoxia rat model (Taraseveciene-Stewart et al. 2001; de Raaf et al. 2014). As hypoxia, via HIF-1alpha and VEGF, mobilizes bone marrow cells, it is likely that bone marrow-derived precursors home to the lung and participate in pulmonary vascular remodeling; perhaps these cells arrive in the vascular wall via the vasa vasorum (Nijmeh et al. 2014). The process of hypoxia-induced pulmonary arteriolar media hypertrophy may be initiated by EnMT and thus the endothelial cells may be the first responders, quickly providing myofibroblasts, and in doing so, providing the early defense against hemodynamic (shear) stress. Possibly, this EnMT-driven “adequate” adjustment of the resistance vessels to increased shear stress also protects against angio-obliteration (unpublished own data).
16.8 The Role of the Immune System
As stated, the vascular lesions in severe PAH are multicellular and complex and the presence of inflammatory cells in and around these lesions has been appreciated for many years (Wagenvoort and Wagenvoort 1977; Tuder et al. 1994; Hassoun et al. 2009). Here it is important for us to examine whether immune dysregulation can influence progenitor and stem cell behavior during the development of PAH.
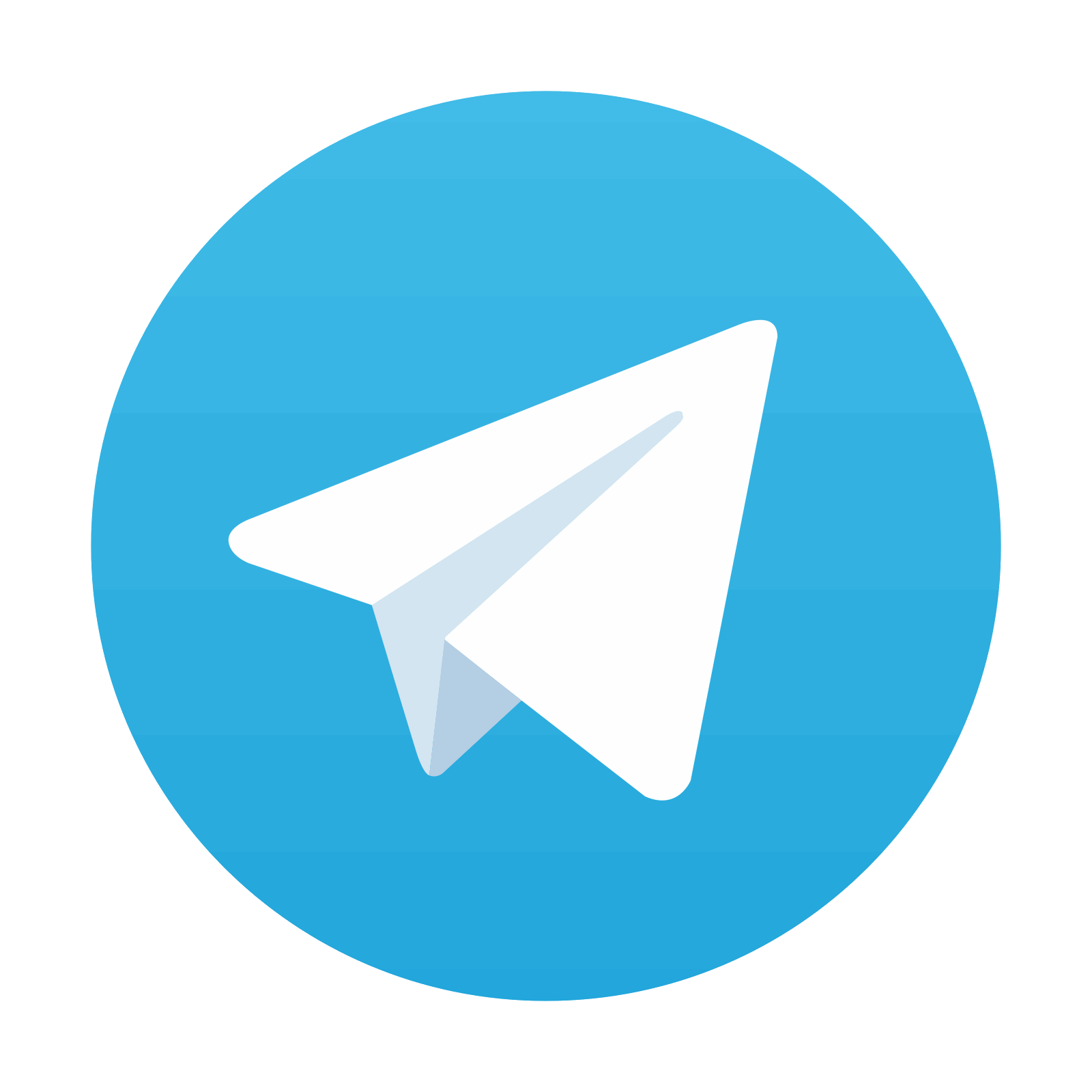
Stay updated, free articles. Join our Telegram channel

Full access? Get Clinical Tree
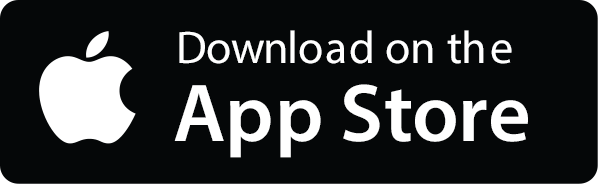
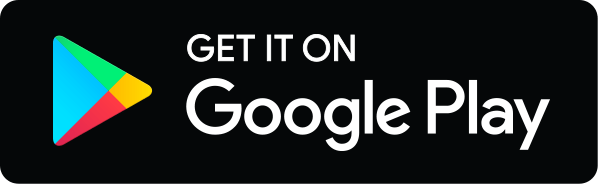