13
Prosthetic Valves
Echocardiographic evaluation of prosthetic valves is similar, in many respects, to the evaluation of native valve disease. However, there are some important differences. First, there are several types of prosthetic valves with differing fluid dynamics for each basic design and differing flow velocities for each valve size. Second, the mechanisms of valve dysfunction are somewhat different from those for native valve disease. Third, the technical aspects of imaging artificial devices—specifically, the problem of acoustic shadowing—significantly affect the diagnostic approach when prosthetic valve dysfunction is suspected (Table 13-1).
TABLE 13-1
Prosthetic Valves: Clinical Echocardiographic Correlates
AF, atrial fibrillation; AR, aortic regurgitation; MR, mitral regurgitation.
Echocardiographers increasingly are asked to evaluate prosthetic valve function because of the increasing number of prosthetic valves implanted annually and the greater longevity of patients with prosthetic valves. Both an understanding of the basic approach to echocardiographic evaluation (as outlined in this chapter) and detailed knowledge of the specific flow dynamic for the size and type of prosthesis in an individual patient (see Suggested Readings 2 and 3) are needed for appropriate patient management.
Basic Principles
The three basic types of surgical prosthetic valves (Figs. 13-1 and 13-2) are:
Figure 13–1 Examples of mechanical valve types.
A, A bileaflet valve (St. Jude Medical Regent valve) and (B) a tilting-disk valve (Medtronic-Hall valve). Images of other specific valve types can be found using a web search. (A, Copyright St. Jude Medical Inc., St Paul, Minnesota. B, Copyright Medtronic, Inc., Minneapolis, Minn.)
Figure 13–2 Examples of surgical tissue valve prostheses.
Carpentier-Edwards Perimount aortic valve (A), St. Jude Trifecta valve (B), and Medtronic stentless freestyle valve (C) (A, Copyright Edwards Lifesciences LLC, Irving, Calif. B, Copyright St. Jude Medical Inc., St Paul, Minn. C, Copyright Medtronic Inc., Minneapolis, Minn.)
Bioprosthetic Valves
Tissue valves are composed of three biologic leaflets with an anatomic structure similar to that of the native aortic valve. With stented prosthetic valves, the leaflets (typically porcine), or pericardium (usually bovine or equine) shaped to mimic normal leaflets, are mounted on a cloth-covered rigid support that functions as the crown-shaped aortic annulus with a raised “stent” at each of the three commissures (Fig. 13-3). Variations in the support structure and leaflet types abound in commercially available valves; some include anticalcification treatments. Current generation stented tissue valves include the Edwards Magna, St. Jude Trifecta and the Medtronic Mosaic valve. Older examples of stented heterografts include Carpentier-Edwards porcine valves, Hancock porcine valves, and Ionescu-Shiley bovine pericardial valves. “Stentless” tissue valves also have been developed that use a flexible cuff of fabric or tissue, instead of rigid stents, to support the valve leaflets. Stentless valves often are implanted as part of a composite tissue valve and aortic root, for example, the Medtronic freestyle valve and root.
Figure 13–3 Bioprosthetic mitral valve.
Parasternal long-axis view of a stented mitral bioprosthetic valve. A, The valve struts (arrow) protrude into the ventricular chamber, with antegrade flow seen with color Doppler (B) directed toward the ventricular septum.
In the past, bioprosthetic valves were only implanted surgically using cardiopulmonary bypass to support the circulation while the valve is implanted. Newer approaches to aortic and pulmonic valve implantation include a transapical surgical approach and a nonsurgical transcatheter approach. The bioprosthetic valves used for catheter implantation are mounted on a compressible stent (see Suggested Readings 20 to 24).
Mechanical Valves
A bileaflet valve in which two semicircular disks hinge open to form two large lateral orifices and a smaller central orifice (Figs. 13-4 and 13-5)
Figure 13–4 Bileaflet mitral valve prosthesis.
TEE images show (A) the sewing ring and two parallel open leaflets (arrows) in diastole. B, In systole, the two leaflets close with a slightly obtuse closure angle with reverberations from the leaflets and shadowing from the sewing ring (arrows) obscuring the LV side of the valve.
Figure 13–5 Normal Doppler color flow patterns with a bileaflet mitral valve prosthesis.
In diastole (A), antegrade flow is seen across the valve with two large lateral orifices (arrows) and a small central orifice, which appears red because of local flow acceleration. In systole (B), two normal jets of regurgitation (arrows) are seen.
A tilting-disk valve in which a single circular disk opens at an angle to the annulus plane, being constrained in its motion by a smaller “cage,” a central strut, or a slanted slot in the valve ring
Mechanisms of Prosthetic Valve Dysfunction
Thromboembolic Complications
Prosthetic valves, particularly mechanical valves, are prone to thrombus formation which may result in systemic embolic events or valve dysfunction. Echocardiographic evaluation for prosthetic valve thrombus is limited, except with very large masses, because of shadowing and reverberations. In addition, clinical events may be associated with clots smaller than the limits of clinical ultrasound resolution. Thus, echocardiography cannot exclude the possibility of thrombus on a prosthetic valve; in patients with embolic events, the prosthetic valve itself is potential source of embolus.
Technical Aspects of Echo Evaluation
There are two major challenges in evaluating prosthetic valves by echocardiography. The normal fluid dynamics of the prosthetic valve must be distinguished from prosthetic valve dysfunction. However, the most technically limiting aspect of the echocardiographic evaluation of prosthetic valves is the problem of acoustic shadowing. The sewing rings of both bioprosthetic and mechanical valves and the occluders of mechanical valves are strong echo reflectors, resulting in acoustic shadows and reverberations (Fig. 13-6). These reverberations and shadows obscure the motion of the valve structures themselves and block detection of imaging and Doppler abnormalities in the acoustic shadow region. During the examination, considerable effort is directed toward utilizing windows and views that avoid these imaging artifacts. Transesophageal echocardiography (TEE) is particularly useful in the evaluation of prosthetic mitral valves because it provides acoustic access from the left atrial (LA) side of the valve. Three-dimensional (3D) imaging often is helpful, although acoustic shadowing and reverberations still limit optimal valve visualization.
Figure 13–6 Acoustic shadowing on TTE and TEE with prosthetic valves.
Effect of mechanical prosthetic valve position and echocardiographic imaging view on shadowing and masking of a regurgitation jet by Doppler. A higher effect from TTE imaging is seen on prostheses in the mitral position compared to the aortic position. (Modified from Zoghbi WA, Chambers JB, Dumesnil JG, et al: Recommendations for evaluation of prosthetic valves with echocardiography and Doppler ultrasound. J Am Soc Echocardiogr 22[9]:975-1014, 2009.)
Echocardiographic Approach
Bioprosthetic Valves
Stented tissue prosthetic valves have a trileaflet structure similar to that of a native aortic valve. An M-mode recording through the leaflets shows the typical “boxlike” opening in systole (for the aortic position) or diastole (for the mitral position) as is seen with a normal native aortic valve. However, with conventional valve designs, the echogenic sewing ring and struts may limit visualization of the leaflets with the specific ultrasound appearance of the supporting structures depending on the specific model (Fig. 13-7). Because there is marked variability in surgeons’ valve preferences, it is helpful to look online at photographs of the valves most commonly encountered at your institution. Stentless bioprosthetic valves have an echocardiographic appearance very similar to a native aortic valve, other than increased echogenicity in the aortic root in the early postoperative period. This valve is best identified by reviewing the chart or asking the patient about any cardiac surgical procedures before beginning the study. Percutaneous valves (Fig. 13-8) appear similar to a native valve with increased paraannular thickness due to the supporting expanded stent.
Figure 13–7 Bioprosthetic aortic valve.
A, Parasternal long-axis view of a normal stented aortic valve replacement (AVR). The orifice (double arrow) between the thin open leaflets is seen with the struts of the stent (single arrows) seen within the sinuses. B, In the short-axis view, the triangular opening of the three leaflets is seen with the three struts (arrows) located in the position of the native valve commissures.
Figure 13–8 Transcatheter aortic valve bioprosthesis.
Parasternal long-axis view zoomed to show calcific aortic stenosis (AS) (A), followed by percutaneous placement of an aortic valve prosthesis (B). The valve cage (arrows) is seen corresponding to the photograph of the Edwards SAPEIN transcatheter heart valve (C). (C, Copyright Edwards Lifesciences LLC, Irvine, Calif.)
Improved images of prosthetic tissue valves can be obtained from a TEE approach, particularly for valves in the mitral position, because the ultrasound beam has a perpendicular orientation to the leaflets with no intervening structures from this approach. With aortic valve prostheses, TEE imaging is less rewarding because the posterior part of the sewing ring shadows the valve leaflets. When images of the leaflets themselves are suboptimal, Doppler data can provide valuable information.
Mechanical Valves
Ultrasound imaging of mechanical valves from a transthoracic echocardiographic (TTE) approach is frustrating because of severe reverberations and acoustic shadowing. While imaging may provide clues as to the type of valve prostheses (e.g., “low-profile” bileaflet or tilting-disk valve versus “high-profile” ball-cage valve), obviously it is simpler to ascertain the exact valve type and size from the patient’s medical record or valve identification card. Assessing motion of the valve occluder often is difficult. For example, the leading edge of a tilting-disk valve results in a strong reverberation across the image obscuring motion of the disk itself. In addition, an oblique image plane often is obtained relative to the prosthetic valve because orientation of the prosthesis within the annulus is not standard. With a tomographic plane perpendicular to the open bileaflet valve, the two leaflets can be identified clearly; this is an image plane that is best identified on multiplane TEE imaging or using 3D volumetric imaging (Fig. 13-9).
Figure 13–9 3D imaging of a bileaflet mechanical mitral prosthesis.
On this diastolic TEE image, looking from the LA side of the valve, the two open valve occluders are well seen.
Normal Doppler Findings
The motion of the occluder of a mechanical valve (or the tissue leaflets of a biologic valve) creates a brief, intense Doppler signal that appears as a dark narrow band of short duration on the spectral display (Fig. 13-10). Audibly, this signal is similar to the valve “click” appreciated on auscultation. However, unlike auscultation, usually both opening and closing valve clicks are seen on spectral Doppler analysis. The Doppler signals associated with valve opening and closing are similar to those seen with native valves but are of greater intensity. The motion of the occluder also may result in color flow artifacts, with color signals covering large areas of the image that are inconsistent from beat to beat.
Antegrade Flow Patterns and Velocities
Flow profiles of different mechanical valves vary substantially, and none is analogous to flow across a normal native valve. Bileaflet mechanical valves have complex fluid dynamics that affect the Doppler echocardiographic evaluation of these valves. With the leaflets open, there are two large lateral valve orifices with a small narrow central slit-like orifice. The flow velocity profile shows three peaks corresponding to these three orifices, with higher velocities in the center of each orifice. The local acceleration forces within the narrow central orifice result in localized high-pressure gradients in this region of the valve, which often are substantially higher than the overall pressure gradient across the valve (Fig. 13-11).
Figure 13–11 Antegrade velocity across a normal aortic valve replacement.
The antegrade velocity is slightly increased compared to that of a native valve, but the triangular shape indicates a normal flow pattern. Prominent valve clicks are seen. The flow in diastole is transmitral flow, not aortic regurgitation, based on timing, velocity, and shape of the diastolic signal. This patient also has a mechanical mitral valve (note the two valve clinks close together on the second beat).
With a ball-cage valve in the open position, blood flows across the sewing ring and around the ball occluder on all sides. When the valve closes, a small amount of regurgitation is seen circumferentially around the ball as it seats in the sewing ring.
Prosthetic valve normal velocities, pressure gradients, and valve areas depend on the valve type, size, and position. However, compared to a normal native valve, all prosthetic valves are inherently stenotic to some extent. Specifically, the expected antegrade velocities and pressure gradients across a normally functioning prosthetic valve are higher than the corresponding values for a native valve. Similarly, the effective orifice area of a prosthetic valve is smaller than the orifice area of a normal native valve. While manufacturers have data on in vitro flow characteristics for each valve, in vivo echocardiographic data are sparse owing to the large number of valve types and sizes. Even in a large study, only a few patients have the same valve type, position, and size. In the available Doppler studies of normal prosthetic valves, data often are presented in various ways. Some studies report the mean ± 1 standard deviation for each variable; others include the range as well. Expected normal velocities, pressure gradients, and valve areas for several commonly seen prosthetic valves are shown in Tables 13-2 and 13-3.
TABLE 13-2
Normal Doppler Parameters for Selected Mechanical and Bioprosthetic Aortic Valves
Data from Rosenhek R, Binder T, Maurer G, et al: Normal values for Doppler echocardiographic assessment of heart valve prostheses. J Am Soc Echocardiogr 16:1116–1127, 2003.
TABLE 13-3
Normal Doppler Parameters for Selected Mechanical and Bioprosthetic Mitral Valves
Data from Rosenhek R, Binder T, Maurer G, et al: Normal values for Doppler echocardiographic assessment of heart valve prostheses. J Am Soc Echocardiogr 16:1116–1127, 2003.
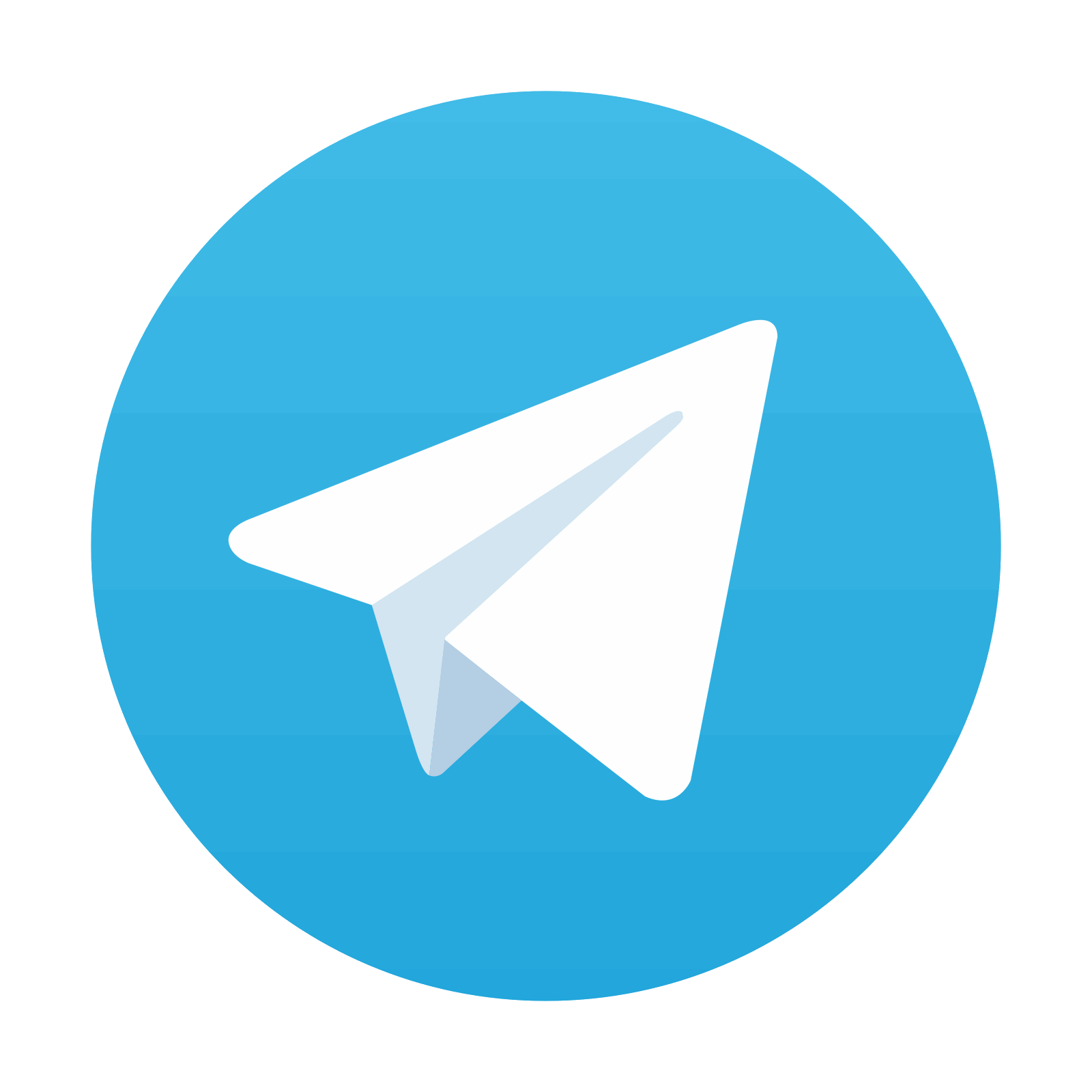
Stay updated, free articles. Join our Telegram channel

Full access? Get Clinical Tree
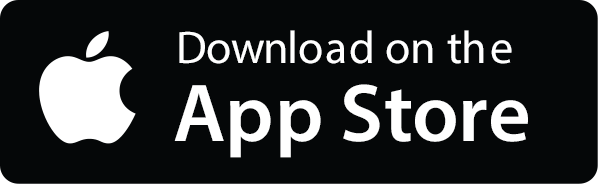
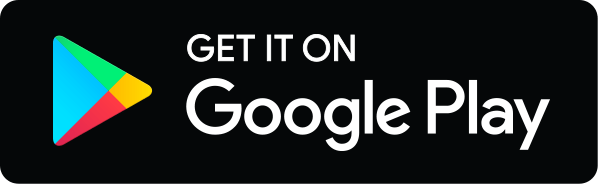