Background
Coronary and microvascular blood flow reserve have been established as important predictors of prognosis in patients with cardiovascular disease. The aim of this study was to assess the value of coronary flow velocity reserve (CFVR) and real-time myocardial perfusion echocardiography (RTMPE) for predicting events in patients with nonischemic dilated cardiomyopathy.
Methods
One hundred ninety-five patients (mean age 54 ± 12 years; 66% men) with dilated cardiomyopathy (left ventricular ejection fraction < 35% and no obstructive coronary disease on invasive angiography or multidetector computed tomography) who underwent dipyridamole stress (0.84 mg/kg over 10 min) RTMPE were prospectively studied. CFVR was calculated as the ratio of hyperemic to baseline peak diastolic velocities in the distal left anterior coronary artery. The replenishment velocity (β), plateau of acoustic intensity ( A N ), and myocardial blood flow reserve were obtained from RTMPE.
Results
Mean CFVR was 2.07 ± 0.52, mean A N reserve was 1.05 ± 0.09, mean β reserve was 2.05 ± 0.39, and mean myocardial blood flow reserve ( A N × β) was 2.15 ± 0.48. During a median follow-up period of 29 months, 45 patients had events (43 deaths and two urgent transplantations). Independent predictors of events were left atrial diameter (relative risk, 1.16; 95% confidence interval, 1.08–1.26; P < .001) and β reserve ≤ 2.0 (relative risk, 3.22; 95% confidence interval, 1.18–8.79; P < .001). After adjustment for β reserve, CFVR and myocardial blood flow reserve no longer had predictive value. Left atrial diameter added prognostic value over clinical factors and left ventricular ejection fraction (χ 2 = 36.8–58.5, P < .001). Beta reserve added additional power to the model (χ 2 = 70.2, P < .001).
Conclusions
Increased left atrial diameter and depressed β reserve were independent predictors of cardiac death and transplantation in patients with nonischemic dilated cardiomyopathy. Beta reserve by RTMPE provided incremental predictive value beyond that provided by current known prognostic clinical and echocardiographic factors.
Dilated cardiomyopathy (DCM) is a prevalent cardiovascular condition associated with left ventricular dysfunction and poor prognosis. The reliable selection of patients at higher risk for hard events is of great importance for their management, especially considering current available therapeutic approaches, such as implantable cardioverter-defibrillators, and limited resources for health care. Moreover, outcomes have been improved for most high-risk patients, which have resulted in a shift in the selection process for patients referred for heart transplantation. Multivariate analysis of clinical and echocardiographic variables has helped identify significant predictors of survival, and prognostic models have been developed and validated.
The incorporation of new advances in cardiovascular medicine may have a potential prognostic impact in patients with DCM. In this context, impairment of coronary flow velocity reserve (CFVR) by Doppler echocardiography in the left anterior descending coronary artery (LAD) has been associated with higher mortality and progression of heart failure. Neglia et al. also demonstrated that severely depressed myocardial blood flow analyzed by positron emission tomography is a predictor of poor prognosis in patients with idiopathic left ventricular dysfunction, independent of the degree of left ventricular functional impairment and the presence of overt heart failure. Quantitative contrast echocardiography has been shown to be useful for assessing global and regional myocardial blood flow reserve (MBFR) using different pharmacologic stimuli. This technique seems feasible for the assessment of mechanistic insights at a microvascular level and has been applied for evaluating the effects of therapy and predicting mortality in patients with heart failure.
In this study, we sought to determine the value of CFVR and parameters of flow reserve obtained by quantitative myocardial contrast echocardiography for predicting cardiac death and heart transplantation in patients with nonischemic DCM. In addition, we investigated whether these parameters added incremental prognostic value over clinical and resting echocardiographic data in this specific group of patients.
Methods
Patients
From October 2005 to July 2011, we prospectively studied 201 consecutive patients with nonischemic DCM who underwent dipyridamole stress with measurement of CFVR by Doppler echocardiography in the LAD and quantitative analysis of myocardial blood flow by real-time myocardial perfusion echocardiography (RTMPE). All patients were on standard medical treatment for ≥1 year, including angiotensin-converting enzyme inhibitors or angiotensin II receptor blockers, β-blockers, diuretics, and digoxin. The doses of these medications were stable for ≥3 months. Inclusion criteria were age >18 years, left ventricular systolic dysfunction, defined as left ventricular ejection fraction (LVEF) < 35% by Simpson’s rule, and no obstructive coronary artery disease by invasive angiography or multidetector computed tomography. Exclusion criteria were decompensated heart failure (defined as New York Heart Association [NYHA] functional class IV or need for intravenous inotropic drugs) within 2 weeks of stress testing, valvular heart disease, serious ventricular arrhythmias, atrial fibrillation, previous myocardial infarction, previous percutaneous coronary intervention, severe obstructive pulmonary disease, second-degree or third-degree atrioventricular block, allergy to echocardiographic contrast, presence of any intracavitary shunt, pregnancy, and breast feeding. Patients who did not complete ≥6 months of follow-up without events were also excluded. All clinical management decisions were taken by physicians who were unaware of the results of RTMPE. Patients who underwent cardiac resynchronization therapy during follow-up were censored at the time of the procedure. Informed consent was obtained from each patient, and the study protocol conformed to the ethical guidelines of the Declaration of Helsinki, as reflected in a priori approval by the ethics committee of the Heart Institute at the University of São Paulo Medical School.
Study Protocol
Before stress testing, patients were requested to abstain from xanthine-containing food and drinks for ≥24 hours. Patients underwent complete resting two-dimensional echocardiography, followed by measurement of LAD velocities and RTMPE using a commercially available ultrasound system equipped with myocardial contrast echocardiographic software (iE33; Philips Medical Systems, Bothell, WA).
Coronary blood flow in the distal LAD was detected using color Doppler, first obtaining a modified foreshortened two-chamber view or, if distal LAD flow was not feasible, using a low parasternal short-axis view of the base of the heart. Pulsed-wave sample volume positioning in the distal LAD was guided by contrast-enhanced color Doppler imaging.
Myocardial perfusion imaging was performed using a low–mechanical index real-time pulse sequence scheme (power modulation) using a mechanical index of 0.2, a frame rate of 25 to 30 Hz, and continuous intravenous infusion of either lipid-encapsulated microbubbles (Definity; Lantheus Medical Imaging, North Billerica, MA) or perfluorocarbon-exposed sonicated dextrose albumin. The formulation of perfluorocarbon-exposed sonicated dextrose albumin has been described elsewhere.
The contrast infusion rate was adjusted for complete left ventricular cavity opacification, and shadowing in the mid left atrial (LA) cavity was obtained. Once stable myocardial enhancement was reached, the contrast infusion rate was kept constant, Doppler recordings in the distal LAD were performed, and sequences of low-power perfusion images in the apical two-chamber, three-chamber, and four-chamber views were acquired at baseline. To assess the replenishment kinetics, microbubble destruction in the myocardium was induced by a manually delivered flash containing five consecutive high–mechanical index (1.4) impulses and acquired sequences contained ≥15 cardiac cycles. After the completion of resting perfusion sequences, contrast infusion was stopped, and hyperemia was induced by dipyridamole infusion through a parallel port at dose of 0.84 mg/kg over 10 min. At the end of dipyridamole infusion, contrast infusion was restarted at the same rate as in the baseline study, and Doppler recordings in the distal LAD and perfusion sequences were acquired for hyperemic measurements. Blood pressure and cardiac rhythm were continuously monitored before, during, and up to 20 min after dipyridamole infusion. Twelve-lead electrocardiograms were obtained at baseline and at 2-min intervals during dipyridamole infusion.
Quantitative Analysis of RTMPE
Cardiac measurements and hemodynamic evaluations were obtained as recommended by the American Society of Echocardiography. End-diastolic and end-systolic volumes and LVEF were calculated using a modified Simpson’s biplane method. Mitral regurgitation was classified as absent, mild, moderate, or severe using qualitative analysis by Doppler color flow mapping and quantitative parameters, including vena contracta width, regurgitant volume and fraction, and effective regurgitant orifice area. Diastolic dysfunction was classified as degree I (abnormal relaxation), II (pseudonormal pattern), III (reversible restrictive pattern), or IV (fixed restrictive pattern). CFVR was calculated as the ratio of hyperemic to baseline peak diastolic velocities in the distal LAD. The average of three consecutive cardiac cycles with the best spectral curve was considered for analysis.
Real-time myocardial perfusion echocardiographic quantification was performed using commercially available software (QLAB version 7.0; Philips Medical Systems). Only end-systolic frames were selected for analysis. The left ventricle was divided into 17 segments. Basal segments of the left ventricle were excluded from the quantitative analysis because of well-known ultrasound attenuation. Regions of interest were traced manually within the myocardial segments and in the adjacent left ventricular cavity. Myocardial intensity data were corrected for noncontrast signals arising from the tissue by subtracting the signal intensity of the first frame after manual bubble destruction. Myocardial plateau ( A M ) and adjacent left ventricular plateau ( A LV ) signal intensities and the signal intensity exchange rate (β), which is proportional to myocardial blood flow velocity, were calculated automatically by the software. Normalized myocardial acoustic intensity ( A N ) was defined as the ratio of A M to A LV , representing the relative myocardial blood volume. To originate an index of myocardial blood flow, the product of A N and β was calculated. For each study subject, A N reserve, β reserve, and MBFR ( A N × β) were calculated as the arithmetic means of values obtained individually in the 11–myocardial segment regions of interest. The cutoff value of 2.0 has been previously reported in patients with nonischemic DCM and was used in this study to differentiate normal reserve (>2.0) and depressed reserve (≤2.0). The intraobserver variability for the measurements of β reserve and MBFR in our laboratory has been previously reported as 2.1% ( r = 0.99) and 7.4% ( r = 0.95), respectively. The interobserver variability for the same parameters was 6.8% ( r = 0.98) and 5.5% ( r = 0.97), respectively. All echocardiographic analyses were performed by one experienced observer who was blinded to prognostic data.
Follow-Up
Follow-up was obtained by review of the patients’ hospital charts and electronic records and by telephone interviews with the patients. The study end points were cardiac death and refractory heart failure requiring heart transplantation. Cardiac death was defined as death associated with known or suspected myocardial infarction, life-threatening arrhythmia, or pulmonary edema. Sudden unexpected death occurring without another explanation was included as cardiac death. Patients without events were followed for ≥6 months.
Statistical Analysis
Data are expressed as mean ± SD for continuous variables and as absolute (number) or relative (percentage) frequencies for discrete variables. Discrete variables were analyzed using χ 2 or Fisher’s exact tests, if necessary. Continuous variables among groups were analyzed using Student’s t tests or analysis of variance (two or three groups, respectively, for variables with normal distribution) and Mann-Whitney or Kruskal-Wallis tests (two or three groups, respectively, for variables with distribution not considered normal). Reserves obtained by Doppler imaging (CFVR) and quantitative RTMPE ( A N , β, and MBFR) were analyzed as qualitative variables and defined as normal (>2.0) or depressed (≤2.0). Kaplan-Meier curves were used to estimate the distribution of time to cardiac death and transplantation. A Cox model was used to estimate the relative risk of events for each variable. Differences between time-to-event curves were compared using log-rank tests. The incremental value of flow reserve obtained by RTMPE over clinical and echocardiographic data was assessed in four modeling steps. The first step consisted of fitting a multivariate model of only clinical data. Baseline LVEF was then added to this model, followed by LA diameter and β reserve obtained by dipyridamole stress. The significance of adding additional variables to previous modeling steps was based on the change in model-based likelihood statistics, with degrees of freedom equal to the number of additional variables. Two-tailed P values < .05 were considered statistically significant. Statistical tests were performed using SPSS version 13.0 for Windows (SPSS, Inc., Chicago, IL).
Results
Among 201 patients initially enrolled, five were excluded because they did not complete RTMPE (symptomatic hypotension in three, ventricular tachycardia in one, and atrioventricular block in one). One additional patient was excluded because of loss to follow-up. The final study population consisted of 195 patients with nonischemic DCM who underwent RTMPE and were followed for ≥6 months without events. There were 130 men (66%), and the mean age was 54 ± 12 years. The etiology of DCM was hypertensive in 87 patients (45%), Chagas disease in 38 (19%), idiopathic in 41 (21%), alcoholic in 17 (9%), and others in 12 (6%). Forty-seven patients (24%) were in NYHA functional class I, 95 (49%) were in class II, and 53 (27%) were in class III. A total of 10 patients (5%) had diabetes. One hundred eighty-seven patients (96%) were taking angiotensin-converting enzyme inhibitors or angiotensin II receptor blockers, 188 (96%) were taking β-blockers, 173 (89%) were taking diuretics, 16 (39%) were taking digoxin, and 16 (8%) were taking amiodarone.
The complete protocol of performing measurement of LAD velocities and RTMPE at rest and during dipyridamole stress lasted approximately 20 min. Real-time myocardial perfusion echocardiographic dipyridamole stress resulted in significant side effects in five patients (excluded from the study) and was well tolerated in the remaining patients. Overall, during dipyridamole stress, heart rate increased compared with baseline (71 ± 14 vs 86 ± 13 beats/min, P < .001), systolic blood pressure was maintained (116 ± 20 vs 114 ± 19 mm Hg, P = .091), and diastolic blood pressure decreased (73 ± 13 vs 66 ± 13 mm Hg, P < .001). The contrast agent Definity was used in 133 patients (68%), and perfluorocarbon-exposed sonicated dextrose albumin was used in 62 (32%). Mean LVEF was 26 ± 7% at rest, and no patient had worsening of segmental wall motion during vasodilator stress.
CFVR measurement by Doppler echocardiography was feasible in 97% of patients and MBFR and β reserve in 99% of patients. The overall feasibility of performing the analysis of wall motion, CFVR, and quantitative RTMPE was 97%. Values of LAD velocities and parameters from myocardial blood flow at rest and during dipyridamole, with respective reserves, are listed in Table 1 . Hyperemia resulted in increases of all parameters ( P < .001 vs baseline values). Mean CFVR obtained by Doppler was 2.07 ± 0.52. By quantitative RTMPE, mean A N reserve (a parameter of myocardial blood volume) was 1.05 ± 0.09, mean β reserve (a parameter of myocardial blood flow velocity) was 2.05 ± 0.39, and mean MBFR ( A N × β) was 2.15 ± 0.48. There was no difference between CFVR and β reserve (2.07 ± 0.52 vs 2.05 ± 0.39, P = NS), with a good correlation between the measurements of both parameters ( r = 0.80, P < .001). A N reserve and MBFR were statistically different from other reserves ( P < .001 by analysis of variance).
Parameters | Rest | Hyperemia | P |
---|---|---|---|
A N (dB) | 0.48 ± 0.09 | 0.49 ± 0.09 | <.001 |
β velocity (sec −1 ) | 0.94 ± 0.18 | 1.86 ± 0.38 | <.001 |
Myocardial blood flow (dB · sec −1 ) | 0.44 ± 0.15 | 0.89 ± 0.29 | <.001 |
LAD diastolic velocity (cm · sec −1 ) | 39.43 ± 11.56 | 78.83 ± 21.04 | <.001 |
When analyzing flow reserve obtained by RTMPE, no difference was observed in the territories supplied by the LAD, right coronary artery, or left circumflex coronary artery for A N reserve (1.05 ± 0.15, 1.03 ± 0.14, and 1.06 ± 0.16, respectively, P = .741), β reserve (2.08 ± 0.25, 2.03 ± 0.05, and 2.04 ± 0.94, respectively, P = .581), or MBFR (2.20 ± 0.19, 2.10 ± 0.10, and 2.15 ± 0.11, respectively, P = .085). Table 2 demonstrates the number of patients with normal (>2.0) and depressed (≤2.0) CFVR, β reserve, and MBFR.
CFVR | Total | ||
---|---|---|---|
Normal | Depressed | ||
β Reserve | |||
Normal | 100 | 8 | 108 |
Depressed | 9 | 78 | 87 |
Total | 109 | 86 | 195 |
MBFR | |||
Normal | 101 | 12 | 113 |
Depressed | 8 | 74 | 82 |
Total | 109 | 86 | 195 |
Predictors of Cardiac Death or Urgent Heart Transplantation
Patients were followed for a median of 29 months (range, 6–69 months). A total of 45 patients (23%) had events during the follow-up period (43 cardiac deaths and two urgent heart transplantations). Clinical and echocardiographic characteristics of patients with and without subsequent events are presented in Tables 3 and 4 . Patients with events during follow-up had higher frequencies of depressed CFVR, MBFR, and β reserve than patients without events ( Table 4 ). On the other hand, patients with and without events had similar frequency of abnormal A N reserve.
Variable | With events ( n = 45) | Without events ( n = 150) |
---|---|---|
Age (y) | 55.3 ± 13.8 | 53.4 ± 11.7 |
Men | 28 (62%) | 102 (68%) |
NYHA functional class | ||
I | 6 (13.3%) | 41 (27.3%) ∗ |
II | 19 (42.2%) | 76 (50.7%) |
III | 20 (44.4%) | 33 (22.0%) ∗ |
Etiology | ||
Hypertension | 19 (42.2%) | 68 (45.3%) |
Chagas disease | 15 (33.3%) | 23 (15.3%) ∗ |
Idiopathic | 8 (17.8%) | 33 (22.0%) |
Medications | ||
ACE inhibitors/ARBs | 40 (88.9%) | 147 (98.0%) ∗ |
β-blockers | 43 (95.6%) | 145 (96.7%) |
Digoxin | 20 (44.4%) | 56 (37.3%) |
Diuretics | 44 (97.8%) | 129 (86.0%) |
Vasodilator | 5 (11.1%) | 13 (8.7%) |
Calcium channel blockers | 3 (6.7%) | 12 (8.0%) |
Nitrates | 4 (8.9%) | 6 (4.0%) |
Amiodarone | 6 (13.3%) | 10 (6.7%) |
∗ P < .05 by log-rank test (see Table 4 for details).
Variable | With events ( n = 45) | Without events ( n = 150) |
---|---|---|
LVEDD (mm) | 71.4 ± 8.5 | 66.7 ± 7.3 ∗ |
LVESD (mm) | 63.0 ± 8.8 | 57.5 ± 8.1 ∗ |
LVEDV (mL) | 264.5 ± 84.1 | 213.9 ± 69.1 ∗ |
LVESV (mL) | 204.4 ± 69.2 | 156.4 ± 58.0 ∗ |
LVEF | 0.22 ± 0.6 | 0.28 ± 0.7 ∗ |
LVMI (g/m 2 ) | 150.6 ± 37.3 | 141.6 ± 44.1 |
LA diameter (mm) | 50.4 ± 5.9 | 43.1 ± 6.1 ∗ |
dP/dt (mm Hg/sec) | 517.8 ± 19 | 594.6 ± 174.3 ∗ |
MPI | 0.918 ± 0.215 | 0.904 ± 0.276 |
Mitral regurgitation | ||
Absent | 0 (0%) | 29 (19.3%) |
Mild | 19 (42.2%) | 91 (60.7%) ∗ |
Moderate | 15 (33.3%) | 25 (16.7%) ∗ |
Severe | 11 (24.4%) | 5 (3.3%) ∗ |
Diastolic dysfunction | ||
Absent | 1 (2.3%) | 26 (17.4%) |
Grade I | 10 (23.3%) | 72 (48.3%) ∗ |
Grade II | 19 (44.2%) | 30 (20.1%) ∗ |
Grade III | 10 (23.3%) | 14 (9.4%) ∗ |
Grade IV | 3 (7.0%) | 7 (4.7%) ∗ |
Depressed CFVR | 31 (73.8%) | 55 (37.2%) ∗ |
Depressed A N reserve | 44 (97.8%) | 146 (98.0%) |
Depressed MBFR | 35 (77.8%) | 47 (31.5%) ∗ |
Depressed β reserve | 37 (82.2%) | 50 (33.6%) ∗ |
The predictors of cardiac death and transplantation by univariate analysis were NYHA functional (patients in class III were at higher risk than those in class I), angiotensin-converting enzyme inhibitor or angiotensin II receptor blocker use (patients with no use were at higher risk than those with long-term use), left ventricular diameters and volumes and LA diameter (increased risk with higher values), LVEF (increased risk with lower values), dP/dt (increased risk with lower values), degree of mitral regurgitation and diastolic dysfunction (increased risk with higher degrees), and CFVR, MBFR, and β reserve (patients with depressed reserves were at higher risk than those with normal values) ( Table 5 ).
Variables | Univariate | Multivariate | ||
---|---|---|---|---|
RR (95% CI) | P | RR (95% CI) | P | |
Age | 1.03 (0.98–1.04) | .369 | NS | |
Male gender | 1.29 (0.64–2.58) | .471 | NS | |
NYHA functional class (III × I) | 4.14 (1.49–11.49) | .006 | NS | |
Hypertension | 0.88 (0.45–1.73) | .713 | NS | |
Chagas disease | 2.76 (1.29–5.92) | .009 | NS | |
Idiopathic | 0.76 (0.326–1.805) | .543 | NS | |
ACE inhibitors/ARBs | 0.14 (0.04–0.71) | .016 | NS | |
β-blockers | 0.74 (0.149–3.966) | .726 | NS | |
Diuretics | 7.16 (0.94–54.81) | .06 | NS | |
Vasodilators | 1.32 (0.44–3.92) | .620 | NS | |
Calcium channel blockers | 0.82 (0.77–0.82) | .769 | NS | |
Nitrates | 2.34 (0.63–8.69) | .204 | NS | |
Amiodarone | 2.15 (0.74–6.29) | .161 | NS | |
LVEDD ∗ | 1.08 (1.03–1.13) | .001 | NS | |
LVESD ∗ | 1.08 (1.03–1.13) | <.001 | NS | |
LVEDV ∗ | 1.01 (1.00–1.01) | <.001 | NS | |
LVESV ∗ | 1.01 (1.01–1.02) | <.001 | NS | |
dP/dt ∗ | 0.99 (0.99–1.00) | .033 | NS | |
LA diameter ∗ | 1.21 (1.13–1.30) | <.001 | 1.16 (1.08–1.26) | <.001 |
LVEF ∗ | 0.87 (0.84–0.93) | <.001 | NS | |
MPI | 1.23 (0.31–4.87) | .768 | NS | |
LVMI | 1.00 (0.99–1.01) | .217 | NS | |
Mitral regurgitation ∗ | 3.67 (2.25–5.96) | <.001 | NS | |
Diastolic dysfunction ∗ | 1.94 (1.39–2.72) | <.001 | NS | |
Depressed CFVR (≤2.0) | 4.76 (2.22–10.23) | <.001 | NS | |
Depressed MBFR (≤2.0) | 7.60 (3.47–16.62) | <.001 | NS | |
Depressed β reserve (≤2.0) | 9.16 (3.97–21.14) | <.001 | 3.22 (1.18–8.79) | <.001 |
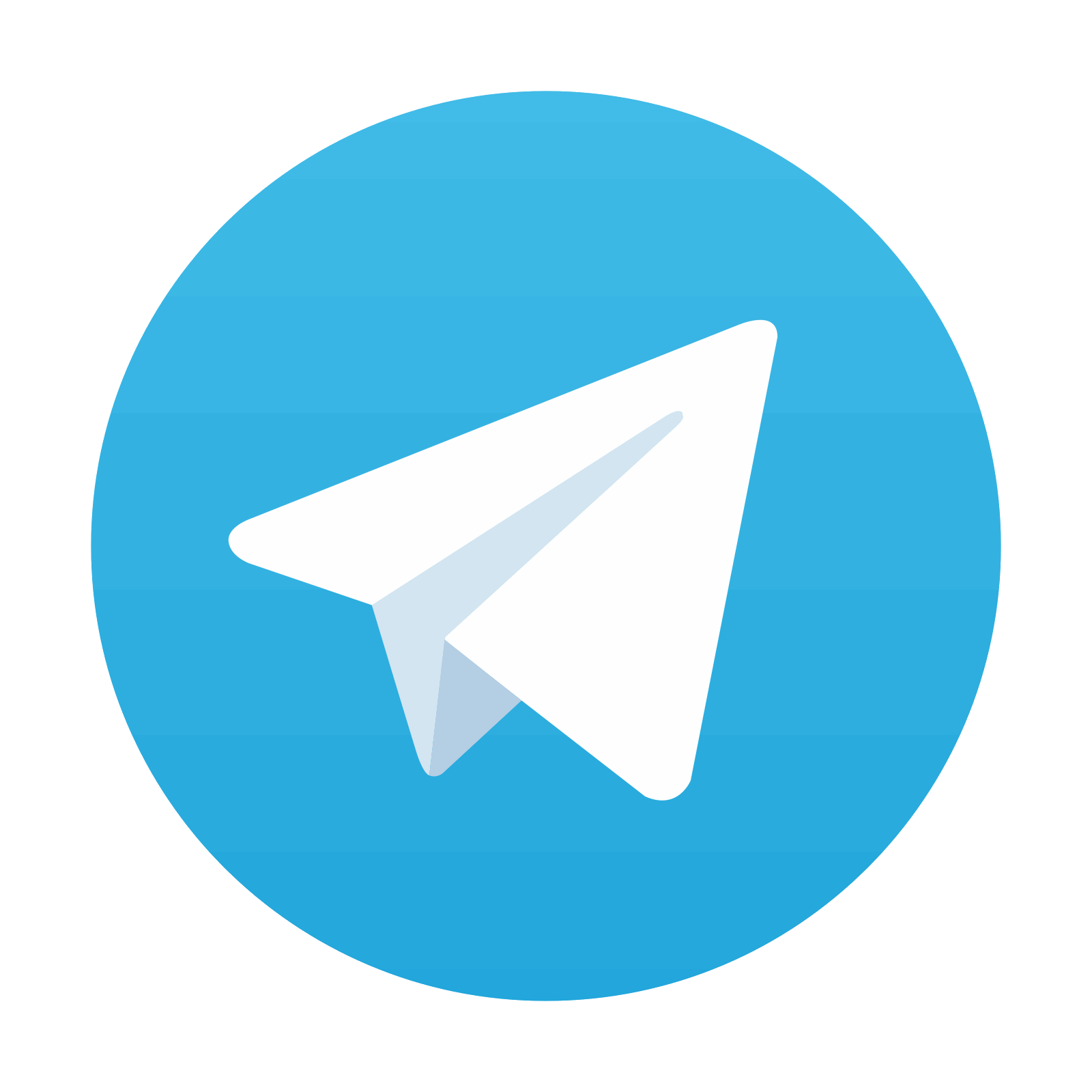
Stay updated, free articles. Join our Telegram channel

Full access? Get Clinical Tree
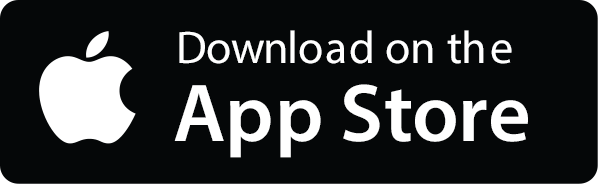
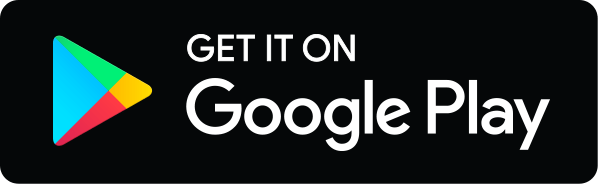
