Providing medical care to a newborn with known or suspected cardiovascular disease can be daunting. An understanding of the pathophysiology of these conditions and the application of a few general principles will promote effective care and minimize the chance of iatrogenic misadventures. It is imperative that a concerted team of specialists with expertise in advanced pediatric cardiac care be assembled, including physicians from several specialties (neonatology, cardiology, critical care, cardiac surgery, and anesthesiology), nurses (neonatal, pediatric cardiac intensive care, and pediatric cardiac acute care), and other health care professionals (respiratory therapists, physical therapists, dieticians, pharmacists, social workers, etc.). Effective and ongoing communication is essential for optimizing care and providing a uniform approach to the management of these complex medical patients.
Heart failure in infancy is a syndrome that occurs as a consequence of the inability of the cardiovascular system to meet the metabolic and growth demands of the infant. It is a common feature of congenital cardiovascular disease presenting symptomatically in neonates. Heart failure in neonates and infants is most commonly caused by structural defects that result in decreased systemic output. In contrast to adults, most infants with heart failure have preserved cardiac contractile function but have increased demands on the cardiovascular system with increased myocardial oxygen requirements. The most common conditions associated with heart failure in infants are those in which there is a dominant left-to-right shunt with excessive pulmonary blood flow and increased oxygen demand. Heart failure in neonates can also result from any structural defect that results in decreased systemic blood flow (eg, severe aortic stenosis) or from myocardial dysfunction regardless of the etiology. Occasionally, heart failure occurs in situations in which the heart is structurally normal, but systemic output is very high and is associated with abnormal distribution of flow (eg, a large arteriovenous malformation), severe anemia, or excessive metabolic demands (eg, neonatal thyrotoxicosis).
The development and progression of heart failure results from a complex interplay of hemodynamic and neurohormonal factors. As illustrated in Figure 11-1, heart failure is viewed as a clinical syndrome that incorporates hemodynamics and compensatory neurohormonal responses in the overall conceptual framework. It should be noted that the roles of compensatory mechanisms that regulate cardiovascular function have been studied largely in chronic compensated states in adult patients and mature animal models. Although it is likely that developmental differences impact the compensatory physiological responses and the responses to therapy that have been designed for adult patients, the general concepts are likely applicable to infants. Additional clinical and experimental studies are necessary to define the spectrum of pathophysiology of heart failure in preterm and term newborn infants.
FIGURE 11-1.
Interrelationships of various influences on the heart failure syndrome. Much of the theoretical and experimental framework for understanding the pathophysiology of heart failure has been developed in adults, but the general concepts are likely to be applicable (with modification) to infants. This schematic diagram is intended to illustrate the complexities involved in determining and modulating the responses to heart failure. Abbreviations: NPs, atrial natriuretic peptides; SNS, sympathetic nervous system; RAAS, renin–angiotensin–aldosterone system.

Systemic output may be insufficient because of (1) reduced ability to eject blood due to either myocardial contractile dysfunction or obstructed outflow (systolic dysfunction), (2) reduced ability of the heart to receive venous return caused by either myocardial diastolic dysfunction or obstructed inflow (diastolic dysfunction), (3) abnormal distribution of cardiac output, or (4) combinations of the first three factors. Regardless of the primary etiology of heart failure, the interaction between the contractile (inotropic) and relaxation (lusitropic) properties of the heart are altered. End-diastolic intraventricular pressure and volume are determined by preload (venous return), the lusitropic state of the myocytes, and the passive compliance of the nonmyocyte elements of the ventricle. On the other hand, end-systolic ventricular pressure and volume are determined by central impedance and peripheral resistance (which together make up the afterload against which the ventricle pumps) in combination with the inotropic state of the ventricular myocardium. These factors are interrelated, and it is often difficult to separate the primary factors from secondary responses.
The fundamental problem in systolic dysfunction is impaired ventricular contractility. The healthy heart is able to increase its output in response to an increase in preload (the Frank–Starling relationship) and can maintain stroke volume in the face of an increase in afterload by increasing its contractile state (homeometric autoregulation). The heart with impaired systolic function is incapable of doing either. The ability to increase stroke volume with an increase in preload is diminished, and a small increase in afterload may lead to a marked decline in the output of a ventricle. The corollary to this is that a small decrease in afterload may significantly improve cardiac output in a heart with systolic dysfunction. This forms the basis for the widespread use of vasodilator therapy for heart failure related to systolic dysfunction.
Afterload is determined primarily by the impedance of the aortic valve, aorta and the other central elastic arteries, and the resistance of the peripheral arterial vasculature. According to the LaPlace relationship, afterload (end-systolic wall stress) is proportional to both end-systolic pressure and end-systolic volume. As afterload increases, because of either an increase in central impedance (eg, aortic stenosis or coarctation of the aorta) or by an increase in peripheral resistance (eg, vasoconstriction caused by alpha-adrenergic stimulation), the ventricle with systolic dysfunction will not eject as much blood as a normal ventricle. Stroke volume will therefore decrease, and end-systolic volume will increase.
Diastolic dysfunction is characterized by either decreased passive ventricular compliance or impaired relaxation. Consequently, increased venous pressure is necessary to sustain adequate ventricular filling, and only small increases in venous return lead to large increases in venous pressure without concomitant increases in stroke volume. This is the basis of the concept of limited preload reserve in diastolic heart failure. That is, abnormal diastolic function may cause symptoms of inadequate cardiac output despite normal systolic function.
Heart failure may be present in neonates despite normal (or near normal) systolic and diastolic cardiac function if systemic blood flow is inadequate to meet the metabolic demands of the neonate. The most common scenario is a structural defect that results in a large left-to-right shunt (eg, large ventricular septal defect, single ventricle with unobstructed pulmonary blood flow, atrioventricular septal defect, large patent ductus arteriosus, or arteriovenous malformation). In this situation, combined ventricular output is high, but because of the large left-to-right shunt, there is excessive pulmonary blood flow. Even though systemic blood flow is usually normal or even increased, metabolic demand is increased far more, driven in large part by an increase in oxygen consumption related to increased work of breathing. In the normal newborn, approximately 20% to 30% of oxygen consumption is related to respiratory work, and it can increase two- to threefold when pulmonary blood flow is elevated. In this setting, which is common in infants with heart failure, compensatory neurohormonal mechanisms are activated, and the heart failure syndrome develops despite normal cardiac pump function. The increased sympathetic state stimulates brown-fat metabolism, which further increases oxygen consumption and contributes significantly to the increased overall metabolic demand.
A variety of neurohormonal signaling pathways and physiologic mechanisms are involved in the normal maintenance and regulation of the cardiovascular system. When systemic output is inadequate for whatever reason, a host of compensatory mechanisms are activated in an effort to maintain oxygen delivery to vital organs. Most of the physiological responses result from activation of the sympathetic nervous system and the renin–angiotensin–aldosterone system. In addition to the direct effects of increased sympathetic tone and increased levels of angiotensin and aldosterone, other responses include increases in vasopressin secretion and endothelin levels and perturbations in nitric oxide signaling in the vasculature and myocardium. In the early stages of heart failure, these and other compensatory mechanisms help to maintain cardiac output and oxygen delivery, but with time and disease progression, these processes become deleterious (Figure 11-2).
FIGURE 11-2.
Compensatory neurohormonal mechanisms in heart failure. Contractile dysfunction (or impaired distribution of blood flow) results in activation of the sympathetic nervous system and the renin–angiotensin–aldosterone system. These systems produce an integrated physiological response intended to provide compensatory support of the cardiovascular system. The potentially deleterious effects include hypertrophy, fibrosis, and cell death that further contribute to cardiac contractile dysfunction such that a cycle of progressive deterioration is established. It is essential to interrupt this cycle to provide optimal long-term management of the heart failure syndrome. Abbreviations: SNS, sympathetic nervous system; RAAS, renin–angiotensin–aldosterone system.

The compensatory increases in neurohormonal activities initially result in an increase in myocardial contractility, selective peripheral vasoconstriction, sodium and water retention, and maintenance of blood pressure. A new state of cardiovascular homeostasis occurs (compensated heart failure syndrome) with higher sympathetic output and increased activity of the renin–angiotensin–aldosterone system. However, when the heart failure state becomes chronic, the same responses that were beneficial in initially maintaining circulatory homeostasis may begin to accelerate myocardial cell death, increase fibrosis, and exacerbate the hemodynamic abnormalities. Furthermore, excessive activation of vasoconstrictor systems is accompanied by a loss of counterregulatory vasodilator influences (nitric oxide, prostacyclin) that adds to the burden of the failing heart.
Enhanced sympathetic activity occurs in adult patients with systolic dysfunction even before clinical signs appear. A clear association exists between increased sympathetic tone (eg, as reflected by increased plasma norepinephrine concentrations) and increased mortality in adult heart failure patients. Increased plasma levels of norepinephrine and β-receptor down-regulation are also reported in the pediatric population.
Although activation of the sympathetic nervous system and renin–angiotensin–aldosterone system is quite effective for short-term compensation, the adverse consequences of continued activation of these systems eventually overcome the initial benefits. Myocardial oxygen consumption increases due to increases in heart rate, contractility, and wall stress. If excessive, these oxygen demands may exceed oxygen delivery to the myocardium, particularly in the subendocardium.
Hypertrophy initially helps compensate for an acute overload by decreasing wall stress and maintaining stroke volume. This occurs by activation of the hypertrophic response genes, which increases the number of functioning contractile elements within the myocytes but does not increase myocyte numbers. These changes in gene expression also involve proteins external to the contractile elements that control myocardial calcium homeostasis. This may eventually diminish the ability to transport calcium to and from the contractile elements efficiently. Furthermore, hypertrophy increases the overall myocardial oxygen requirement.
Ventricular remodeling describes the structural changes in the myocardium that occur in response to changes in loading conditions. As indicated above, the myocyte compartment of the heart responds by hypertrophy. The nonmyocyte components of the heart also respond to autocrine and paracrine signals (eg, angiotensin II and aldosterone) independent of the hemodynamic status. Aldosterone acts on fibroblasts and promotes collagen synthesis. Increased production of collagen decreases the proportion of the myocyte-to-nonmyocyte component of the ventricular myocardium. This leads to increased stiffness of the ventricular wall and contributes significantly to diastolic dysfunction. Additionally, increased cardiac interstitial collagen deposition may contribute to reduced capillary density and increased oxygen diffusion distance. Other more direct effects of remodeling include activation of stretch-sensitive calcium channels. As a result of membrane deformation, resting intracellular calcium levels increase, promoting activation of the hypertrophic gene response. Increases in loading conditions have also been associated with re-expression of fetal genes, early response genes (c-fos, c-jun, c-myc), and atrial natriuretic peptide, all early markers of cardiac hypertrophy.
In addition to the changes mentioned above, activation of the sympathetic nervous system and the renin–angiotensin–aldosterone system may be toxic to the myocytes. Myocyte necrosis occurs via a variety of cytotoxic mechanisms and may be observed in situations of both acute and chronic myocardial dysfunction. Microscopically, cellular swelling and inflammation characterize necrosis. In addition to necrosis, an increased rate of apoptosis appears to be a common feature of the heart failure syndrome. Apoptosis is an active process that involves activation (or lack of suppression) of genes encoding for programmed cell death. Although data are limited in adult human hearts, the intermediary factors responsible for apoptosis appear to be activated by angiotensin II. Apoptosis appears to be increased in the context of vascular remodeling, hypertension, ischemia-reperfusion states, and other circumstances that promote ventricular remodeling. Both necrosis and apoptosis contribute to the progressive loss of myocytes that further diminishes the overall pumping capability of the heart and promotes perpetuation of the cycle of decompensation in heart failure (Figure 11-2).
Inflammation is a tightly regulated process that has been studied for many years. The same cascades that are operative in sepsis and autoimmune diseases are activated to varying degrees in the heart failure syndrome. Cytokines are central regulatory molecules involved in the systemic inflammatory response syndrome. Cytokines are produced by a variety of cells and can be categorized as “pro-inflammatory’ or “anti-inflammatory.” Pro-inflammatory cytokines, such as interleukin-1, interleukin-6, and tumor necrosis factor-α, depress myocyte contractile function, activate immune cells, and suppress production of anti-inflammatory cytokines. In contrast, anti-inflammatory cytokines (eg, interleukins-4, -5, and -10) reduce production of pro-inflammatory molecules and play a protective role in some disease states.
Although oversimplified, several clinical conditions, such as septic shock, are thought to result from an imbalance in these two systems with an unchecked pro-inflammatory response triggered by an inciting event (eg, bacterial infection). An emerging concept in heart failure is that similar immune system derangements contribute significantly to the pathophysiology and progression of the heart failure syndrome. The triggering mechanisms that initiate the systemic inflammatory response syndrome in heart failure and the potential of specific therapeutic strategies remain to be fully characterized. It is important to note that nearly all of this work has been performed in adult patients and mature animal models and that relatively little is known regarding the role of the systemic inflammatory response in neonates with heart failure.
As is evident from the preceding discussion, it is important to think of heart failure as a syndrome that is defined as a disorder of the cardiovascular system, not merely acute pump failure resulting in inadequate oxygen delivery. Heart failure develops as a consequence of compensatory hemodynamic and neurohormonal mechanisms that become overwhelmed or exhausted in response to inadequate systemic blood flow. The signs and symptoms of heart failure result from these compensatory physiological responses and are related to the acuity of the disease process. Based on these concepts, the following definitions provide a framework for understanding and characterizing heart failure in neonates.
Shock is defined as a state of acute circulatory dysfunction with completely overwhelmed and inadequate physiological compensatory mechanisms. These infants are lethargic, exhibit poor perfusion, are often hypotensive and tachycardic, and generally appear quite ill. Blood pressure may be difficult to measure noninvasively. Additionally, blood pressure may be maintained at normal or near normal levels until the terminal stages of shock in infants. Thus, it is important to not rely solely on blood pressure as an indicator of the presence shock in newborns. However, confirmed hypotension in a neonate is an important finding and should prompt immediate intervention. Shock can result from a variety of noncardiac causes in neonates (eg, sepsis, severe anemia). However, cardiogenic shock may also be a presenting feature of left-sided obstructive lesions (such as critical aortic stenosis, interrupted aortic arch or hypoplastic left heart syndrome, or perinatal cardiomyopathy). Less commonly, coronary artery anomalies, such as coronary ostial stenosis, anomalous left coronary artery arising from the pulmonary artery (usually does not present until after 2 months of age), and perinatal myocardial infarction caused by paradoxical venous emboli crossing into the left atrium through the foramen ovale, may present with neonatal cardiovascular collapse. Since the normal physiologic responses are inadequate to maintain circulatory homeostasis, shock must be treated immediately to avoid death.
Acute decompensated heart failure occurs when physiological and neurohormonal compensatory mechanisms are activated, but the responses are insufficient to maintain normal systemic circulation. The development of symptoms or the worsening of a previously compensated state marks the beginning of decompensation and onset of the acute heart failure syndrome. Additional myocardial injury, increased metabolic demands (eg, as a result of infection), or changes in loading conditions may trigger decompensation from a stable compensated state. Symptoms of fluid retention develop or progress because of peripheral vasoconstriction and sodium retention. Respiratory distress secondary to pulmonary venous congestion and hepatic distention caused by fluid retention and/or right ventricular dysfunction are common manifestations of decompensated heart failure syndrome in neonates. Infants with acute heart failure are symptomatic with restlessness, irritability, tachypnea, tachycardia, diminished peripheral perfusion, and decreased urine output. Without intervention, infants in a decompensated state will continue to deteriorate, often rapidly. It is important to recognize acute decompensated heart failure so that appropriate therapy can be provided urgently.
The chronic heart failure syndrome is defined as a stable balance between inadequate systemic blood flow and activation of compensatory hemodynamic and neurohormonal responses. These patients may exhibit few, if any, symptoms of “congestive” heart failure since activation of compensatory mechanisms, primarily the sympathetic nervous system and the renin–angiotensin–aldosterone system, maintains circulatory homeostasis. Often, the only noticeable finding in an infant is failure to thrive because caloric intake is often reduced and metabolic demand is increased because of enhanced sympathetic activity. The patient may demonstrate respiratory distress caused by elevated pulmonary venous pressures, but it is often subtle. This sustained activation of the sympathetic nervous system and the renin–angiotensin–aldosterone system ultimately contributes to progressive (and often silent) deterioration of ventricular function. Chronic heart failure is a common manifestation of many types of structural cardiac defects in neonates resulting from the abnormal distribution of blood flow or abnormal ventricular function.
Neonates represent a unique population in terms of cardiopulmonary function, clinical presentation, and approaches to management. Knowledge of these developmental differences is important in order to understand the pathophysiology of heart failure in neonates and to develop age-appropriate approaches to therapy. Heart failure is a clinical syndrome that must be diagnosed by a comprehensive history and physical examination. No single test can be performed to diagnose the presence of the heart failure syndrome. For example, an echocardiogram can be very helpful in defining cardiac anatomy, ventricular function, and pulmonary artery pressure, but it does not provide a diagnosis of heart failure. An infant may have no clinical evidence of heart failure despite the presence of a large ventricular septal defect or echocardiographic evidence of decreased ventricular systolic function. Conversely, heart failure may be present in the setting of a structurally normal heart and normal ventricular function but with inadequate effective output because of, for example, severe anemia or a large cerebral arteriovenous malformation.
The prenatal and perinatal history may provide clues as to possible acquired causes of heart failure, such as neonatal myocarditis or severe anemia caused by placental bleeding. However, the neonatal history is generally much more informative. Often, the most prominent manifestation of the heart failure syndrome in newborn and young infants is related to the respiratory system. Infants with heart failure are frequently tachypneic, especially with feeding. The feeding history reveals decreased oral intake and prolonged feeding times because of tiring during feeding and increased respiratory effort during oral feeding. Diaphoresis with feeding and emesis are commonly present. These symptoms are exacerbated by a concomitant viral respiratory infection, which may be the reason that the infant is brought to medical care. Infants with chronic heart failure generally exhibit failure to thrive with decreased growth velocity. Unfortunately, the signs and symptoms may be nonspecific as other neonatal illnesses present similarly to heart failure.
Infants with heart failure often appear apathetic and uninterested in their environment and are frequently characterized as irritable. The physical examination often shows tachycardia and tachypnea, even at rest. It is unusual to hear a gallop in newborn infants, even in the presence of severely depressed ventricular function. In infants with a left-to-right shunt, the presence of a diastolic inflow rumble indicates a large shunt with markedly increased pulmonary blood flow. Absence of a heart murmur does not exclude cardiovascular disease. For example, an infant with coarctation of the aorta may have severe obstruction leading to left ventricular dysfunction yet not have a murmur.
The lungs are usually clear to auscultation. With advanced heart failure, there may be diffuse inspiratory crackles or rales, but this is a rare finding. Neonates are more susceptible to atelectasis than older children and adults because of differences in pulmonary function. Functional residual capacity, or the volume of gas present in the lungs at the end of a normal expiration, is the balance between the inward recoil of the lung and the outward recoil of the chest wall. In neonates, the chest wall generates little outward recoil, making the relaxation volume of the thorax smaller than that of the adult. Closing capacity, or the point at which small conducting airways begin to collapse, exceeds functional residual capacity, resulting in the neonatal lung being more susceptible to atelectasis. Furthermore, failure to thrive, a common feature of chronic heart failure in infants, leads to respiratory muscle weakness that can compromise alveolar stability and pulmonary compliance.
The liver is usually enlarged. Jugular venous distension is almost never seen because the liver is very compliant in young infants. The size of the liver is used as a guide to the severity of fluid overload or right ventricular dysfunction. The presence of hepatomegaly in a critically ill infant may differentiate heart failure from sepsis. This is extremely important when considering the need for rapid fluid replacement, which may be harmful in a patient with heart failure. Neonates rarely exhibit peripheral edema unless there is hypoproteinemia or renal failure. The extremities may be cool with diminished cutaneous perfusion if systemic cardiac output is markedly compromised.
Given that heart failure is a dynamic clinical syndrome with involvement of neurohormonal, genetic, biochemical, and inflammatory mediators, a variety of biomarkers may provide useful information for the diagnosis, risk stratification, and monitoring of infants with heart failure. Biomarkers of inflammation, oxidative stress, protease activity, neurohormonal activation, myocyte injury, matrix remodeling, and other pathways have been reported or are currently being studied in adults with heart disease. Most of the heart failure biomarkers that have been studied in adults have not been fully characterized in neonates and young children. Two biomarkers that have been evaluated in children are norepinephrine and b-type natriuretic peptide (BNP). Norepinephrine levels correlate reasonably well with clinical assessments of heart failure severity in infants and children. BNP has been shown to be elevated in newborns with patent ductus arteriosus, and other studies suggest that BNP levels can discriminate between heart disease and respiratory disease in children. However, additional studies are required to determine the utility of measuring levels of norepinephrine, BNP, or other newer biomarkers (such as N-terminal pro-BNP) for risk stratification and monitoring the response to therapy in infants with cardiovascular disease.
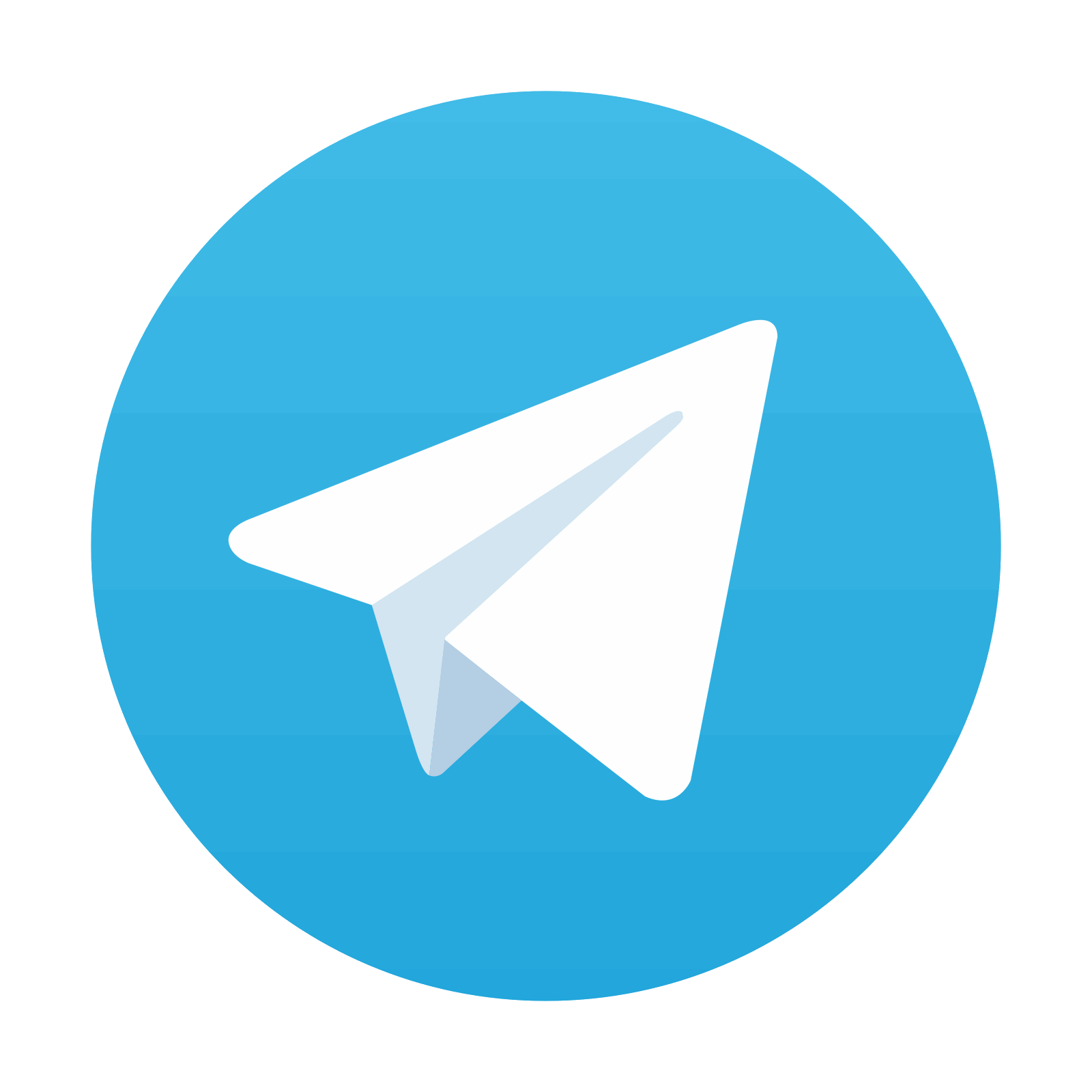
Stay updated, free articles. Join our Telegram channel

Full access? Get Clinical Tree
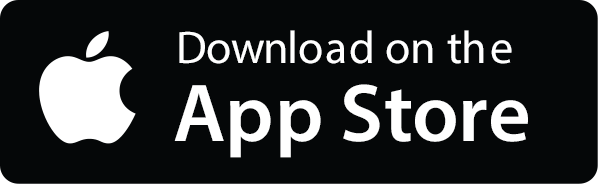
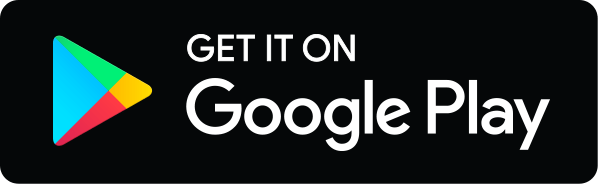