LEARNING OBJECTIVES
Learning Objectives
The student will be able to distinguish between the conducting zone where ventilation occurs, and the respiratory parenchyma where diffusion occurs.
The student will be able to define terms for normal lung volumes, including tidal volume, dead space, total lung capacity, vital capacity, functional residual capacity, inspiratory and expiratory reserve volumes, and residual volume.
The student will be able to describe the basic procedures used in routine pulmonary function testing, particularly bench top spirometry.
The student will be able to describe two techniques to quantify residual volume and functional residual capacity, including underlying assumptions of each.
The student will be able to explain the origin and maintenance of negative intrapleural pressures that are caused by elastic recoil forces within normal lung tissues and the chest wall/diaphragm complex.
Respiration consists of two critical and nonoverlapping processes, ventilation and diffusion. Ventilation is the bulk movement of gases in and out of the lungs via the large and intermediate airways that collectively are termed the conducting zone. Here, inspired gas is warmed to 37°C, humidified to 100% relative humidity (RH), and cleansed of most airborne particulates, but its molecular composition is changed only by addition of water vapor. This is because conducting zone airways are too wide and their walls are too thick to allow appreciable molecular diffusion of O2 and CO2 between inspired air and the pulmonary arterial blood coursing through alveolar capillaries. On the other hand, the delicate alveolar membranes of the respiratory parenchyma are thin and have an enormous aggregate surface area. As will be formally introduced in Chap. 9, molecular diffusion requires such large, thin surfaces to maximize O2 and CO2 exchange. The remainder of this chapter will focus on ventilation, and the forces and constraints that make it more or less effective. It is worth noting now that the conducting zone volume is nearly constant during normal breathing, while respiratory parenchymal volume changes very dramatically as the depth of breathing is adjusted.
NORMAL LUNG VOLUMES AND SPIROMETRY
Despite the histological complexity of lung tissue (Chap. 2), its key elements can be represented by a simple line drawing (Fig. 4.1). The many branching airways of the conducting zone that support ventilation are shown as a single tube comprising the anatomic dead space (VD) (Fig. 4.2). VD is normally ~2 mL/kg of ideal body weight, or about 160 mL in a normal adult. In Fig. 4.1, the respiratory parenchyma where diffusion occurs is shown as one contiguous compartment, the alveolar gas volume (VA), here 3.0 L. We define tidal volume (VT) as the total volume of a typical inspiration, but clearly only a portion of each breath reaches the parenchyma while the remainder occupies VD. Thus, in this example where VD = 160 mL, only 440 mL of a 600 mL VT reaches alveoli of the respiratory parenchyma, or 740 mL of a 900 mL VT. Further, we define total ventilation, V̇E (L/min) as the product of VT and respiratory frequency, f (breaths/min). Using the data of Fig. 4.1, V̇E = 9.0 L/min (= 0.60 L/breath × 15 breaths/min). Consequently, alveolar ventilation, V̇A (L/min) equals (VT – VD) × f. Using volumes in Fig. 4.1, we can compute that at a resting f = 15 breaths/min and VT = 0.60 L, the resulting V̇A of 6.6 L/min will exchange a VA of 3.0 L about twice each minute.
FIGURE 4.2
Actual and schematic views of anatomical dead space VD. (a) Corrosion cast preserving the first 10-12 generations of dichotomously branched human airways. Surrounding lung tissue was dissolved with alkali after liquid plastic instilled into the airways had hardened. (b) Diagram of airways that terminate in true alveoli; the estimate of 22-23 generations was first made by Ewald Weibel in 1962. (a): From Fox. Human Physiology, 10th ed.; 2008. (b): From West Respiratory Physiology – the Essentials. 6th ed. Williams & Wilkins; 2005.
CLINICAL CORRELATION 4.1
Virtually all patients requiring mechanical ventilation will experience an increase in dead space due to the added volume of endotracheal tubing, valves, and associated hardware needed to use the ventilator effectively.
Based on lung tissue morphometry, alveolar capillary volume (VC) contains perhaps 70-100 mL of blood at any instant. Since baseline cardiac output (Q̇) is ~6 L/min, a subject’s VC is typically replenished with mixed venous blood via the pulmonary arterioles at least once per second at rest [(6,000 mL/min)/70 mL] and more frequently during exercise. Thus, erythrocytes spend less than a second traversing a pulmonary capillary that is sufficiently close to alveolar air for diffusive gas exchange. We can also estimate that for an ideal lung with homogeneous architecture from apex to base, the alveolar ventilation/perfusion ratio, V̇A/Q̇ is ~1.0 (ie, 6.6 L/6.0 L). Whether an ideal V̇A/Q̇ ratio of 1.0 actually occurs in normal lung will be discussed in Chap. 8.
Most lung volumes are measured directly by spirometry, an apparatus originally consisting of an inverted, water-sealed bell filled with 100% O2 from which a subject breathes through a mouthpiece while wearing nose clips (Fig. 4.3). As gases move in and out of the bell, pen tracings record volume changes even as expired CO2 is trapped chemically by an internal canister of soda lime. Thus, the eventual decline in bell height (superimposed on ventilatory pen movements) reflects V̇o2. Routine spirometry provides multiple lung volumes plus the rate functions V̇E and V̇o2.
Most spirometric volumes are self-explanatory. A capacity is the sum of two or more volumes. After a normal expiration, the amount of gas remaining in the lungs is called the functional residual capacity (FRC), an important reference value and the standard starting point for other pulmonary function measurements. By forcefully exhaling as much gas as possible beyond a normal VT, we see that FRC is really the sum of the expiratory reserve volume (ERV)
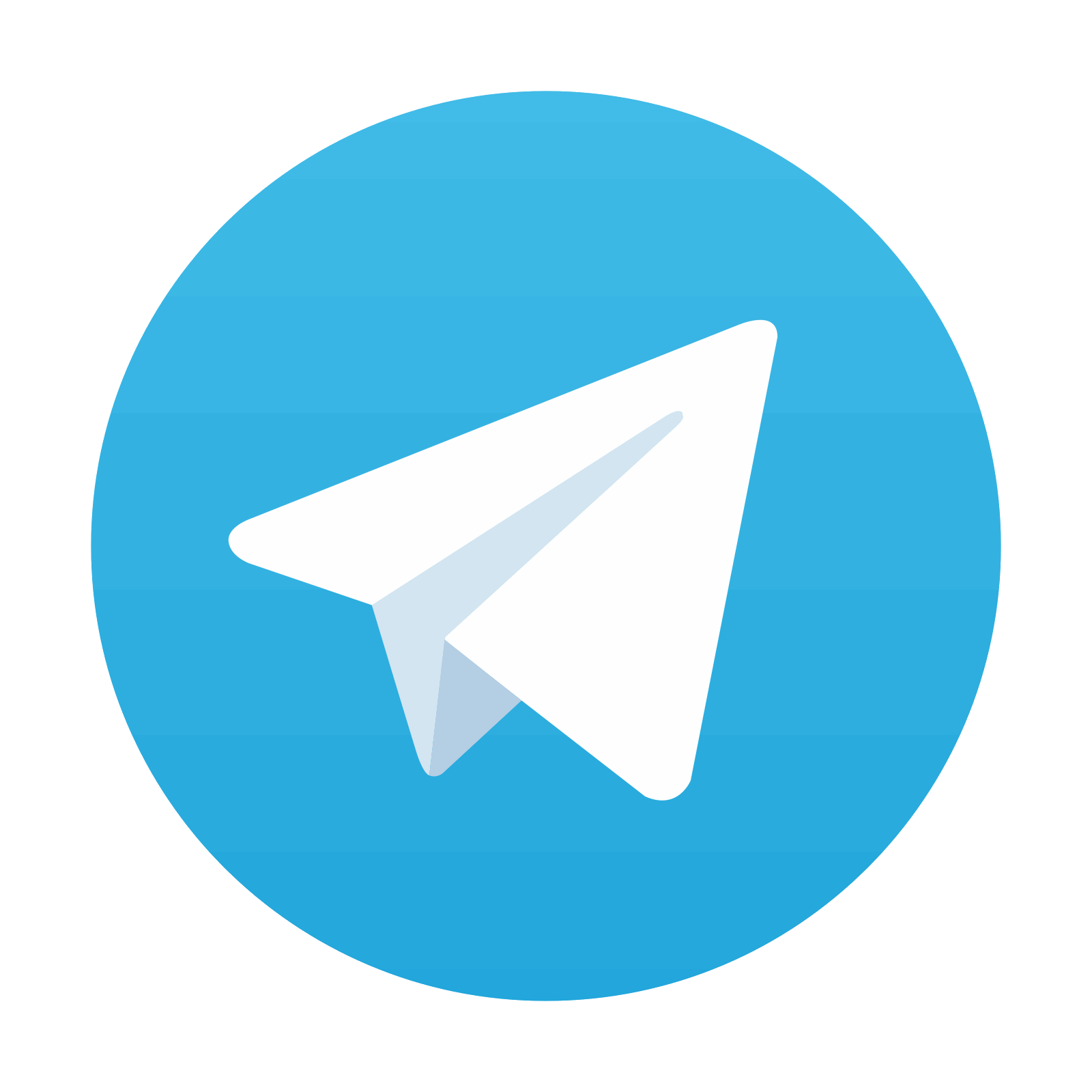
Stay updated, free articles. Join our Telegram channel

Full access? Get Clinical Tree
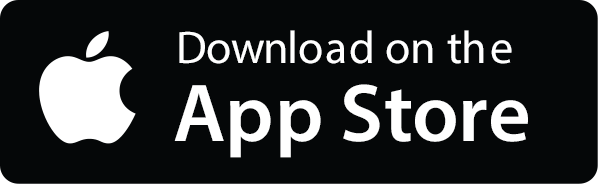
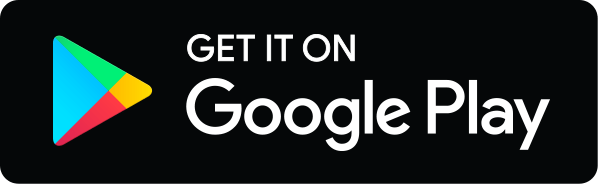
