Fig. 9.1
Simultaneous ECG (black), left ventricular pressure (blue) and volume (red) waveforms illustrate the electromechanical relationship of a cardiac cycle. The P wave represents atrial depolarization and occurs at the end of ventricular diastole. The QRS complex signifies ventricular depolarization and precedes ventricular contraction. The T wave heralds ventricular relaxation and the beginning of diastole. Diastasis is the period of ventricular standstill and the ideal phase for anatomic imaging
ECG Setup
First and foremost, MR-safe electrodes must be used in the MR-environment in order to avoid skin burns. ECG wires should be draped in a straight line over the patient avoiding loops or coil patterns. Most MR systems will use a three or four lead telemetry system to transmit the ECG signal. Electrodes are placed in the left chest area forming a triangle or square around the heart (Fig. 9.2). In women the “left leg” electrode should be placed just below the left breast fold and not on the breast tissue itself. A closer electrode configuration tends to yield higher amplitude signals. Signal amplitude is also increased by ensuring good electrode contact with the skin, facilitated by shaving hirsute patients and the use of coupling gel in all patients.
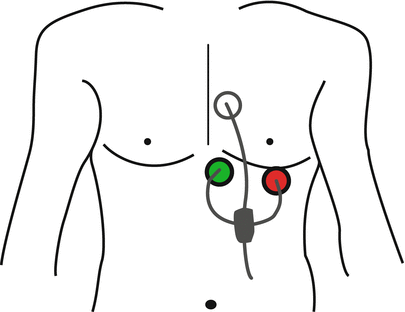
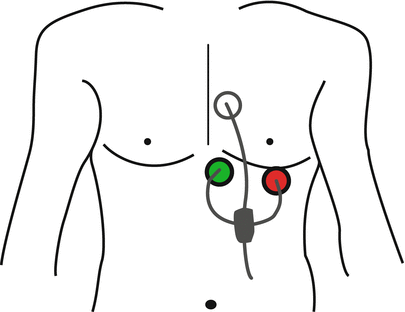
Fig. 9.2
Typical ECG lead placement configuration
In order to ensure proper ECG-gating, the ideal tracing should have predominant R-wave with minimum T wave amplitude. In some individuals the T wave amplitude can exceed the R-wave, which may cause the scanner to inappropriately trigger off of the T wave. While this is not absolutely detrimental to image quality, it can lead to improper placement of the acquisition window during prospective gating and errors in quantitative analysis. Thus, it is best to make adjustments to allow triggering off the R-wave. The ECG tracing profile can be changed by selecting different lead polarities (Lead I, aVF, etc.) at the MR console, however manual electrode adjustments may be necessary. Modern scanners are typically equipped with vectorcardiography (VCG), which can mitigate magnetohydrodynamic (MHD) artifacts as discussed later in the chapter.
Interpreting the ECG Tracing
The ECG tracing can reveal the origin of the electrical activity, rhythm, rate, conduction abnormalities and even give clues about cardiac ischemia or prior myocardial infarctions. For the purpose of cardiac imaging, we are most concerned about the heart rate and rhythm pattern. Heart rate (HR) can be expressed in beats per minute (bpm) as determined by the number of QRS complexes per minute. Cardiac activity is also described by the R–R interval, the cardiac cycle duration between R waves in milliseconds (ms). Heart rate and R-R intervals are related by Eq. 9.1. Table 9.1 lists typical heart rates with the corresponding R-R intervals.

Table 9.1
Heart rate in beats per minute and the corresponding R-R interval as calculated from Eq. 9.1
HR (bpm) | R-R interval (ms) |
---|---|
50 | 1200 |
60 | 1000 |
70 | 857 |
80 | 750 |
90 | 667 |
100 | 600 |
110 | 545 |
120 | 500 |

(9.1)
Heart rhythm is defined by the origin of each electrical impulse and the regularity of the stimuli. Rhythm regularity is most important during CMR and it is easily determined by observing the R-R interval variability. The normal sinus rhythm is regular and usually between 60 and 100 bpm corresponding to R-R intervals of 1000–600 ms. However, individuals referred for CMR may often have slow (bradycardia; <60 bpm), fast (tachycardia; >100 bpm) or irregular heart rhythms (arrhythmias). The most common irregular rhythms seen in typical patients with cardiac disease referred for CMR examination are atrial fibrillation, premature atrial or ventricular contractions, and non-sustained ventricular tachycardia. Prompt recognition of these abnormalities allows the imager to adjust the acquisition technique to achieve optimal image quality and make sure that the exam is completed safely or terminated early if needed to facilitate rhythm management. Later in the chapter we will discuss how to tailor the exam in the setting of cardiac arrhythmias.
ECG-Gating and Data Acquisition
Basic Concepts
The goal of ECG-gating is to synchronize data acquisition to the cardiac cycle, which reduces cardiac motion-induced artifacts and optimizes image quality. The R-wave is the most prominent and easily detected feature on the ECG and serves as a temporal reference point within the cardiac cycle. Thus, it is the preferred marker to gate and trigger data acquisition. Although the terms often used interchangeably, ECG-triggering and ECG-gating are not synonymous. ECG–triggering specifically refers to the initiation of the MRI pulse sequence after detection of an R-wave, whereas ECG–gating refers to the process of matching the acquired data to the corresponding temporal phase within the RR-interval.
Two primary ECG gating modes are commonly used in CMR: prospective and retrospective. In prospective gating, detection of an R wave triggers the MR pulse sequence to acquire a fixed number of data lines at a given timepoint within the R-R interval. Thus, data is prospectively collected at a particular time in the cardiac cycle with respect to the R-wave detection. In retrospective mode, data is collected continuously over several heart beats and retrospectively binned into a fixed number of reconstructed cardiac phases using the detected R-waves as reference points.
These ECG gating modes are combined with two data collection strategies: single–shot or segmented acquisitions. Single-shot pulse sequences are typically prospectively gated and all the data needed to make an image is acquired during a single heart beat. Alternatively, during segmented acquisitions, a fraction of the total number of data lines is acquired with each heartbeat, and the data needed to reconstruct a single image is obtained over multiple R-R intervals. In the next sections we will describe in detail the various ECG-gating and acquisition strategy combinations that are used in a cardiac exam. Table 9.2 summarizes typical ECG-gating and acquisition mode combinations, their respective applications, and their advantages and limitations.
Table 9.2
Common k-space filling strategies and ECG-gating mode combinations, sequences, advantages and limitations
Acquisition mode | ECG-gating mode | Sequence | Advantages and limitations |
---|---|---|---|
Single heartbeat | Prospective | Scouts (SSFP or TSE) | Robust to arrhythmias and breathing artifacts |
Perfusion | Fast acquisition | ||
LGE | Low spatial resolution | ||
Non-ECG-gated | Real time | Insensitive to rhythm or breathing variations | |
Free breathing acquisition | |||
Low spatial and temporal resolution | |||
Segmented | Prospective | T1-weighted | High temporal and spatial resolution |
T2-weighted | Longer acquisition/breath hold | ||
Cine SSFP | |||
Cine VENC | Sensitive to arrhythmias and breathing artifacts | ||
Cine Grid-tagging | Incomplete interrogation of the cardiac cycle for cine and grid-tagging images | ||
LGE | |||
Retrospective | Cine SSFP | High temporal and spatial resolution | |
Cine VENC | Complete acquisition of the cardiac cycle | ||
Cine Grid-tagging | Sensitive to arrhythmias and breathing artifacts | ||
Longer acquisition time |
Prospectively Gated Single-Shot Acquisition
Single-heartbeat (also termed ‘single-shot’) pulse sequences are typically prospectively gated and all the data needed to make an image is acquired during a single cardiac cycle (Fig. 9.3). During a prospectively gated acquisition, data is acquired after a programmed delay, or trigger delay (TD), from the R-wave. Data is then collected for a period called the acquisition window (AW), which typically ends several milliseconds prior to the next heartbeat. This gap, or trigger window (TW), allows the scanner to detect the next R-wave. As a result, data is not sampled during this end-diastolic period of the cardiac cycle. For still frames or anatomic images, the TD and AW are adjusted to allow data collection during diastasis which coincides with the T-P segment (Fig. 9.1). One or multiple slice locations can be acquired during each breath hold.
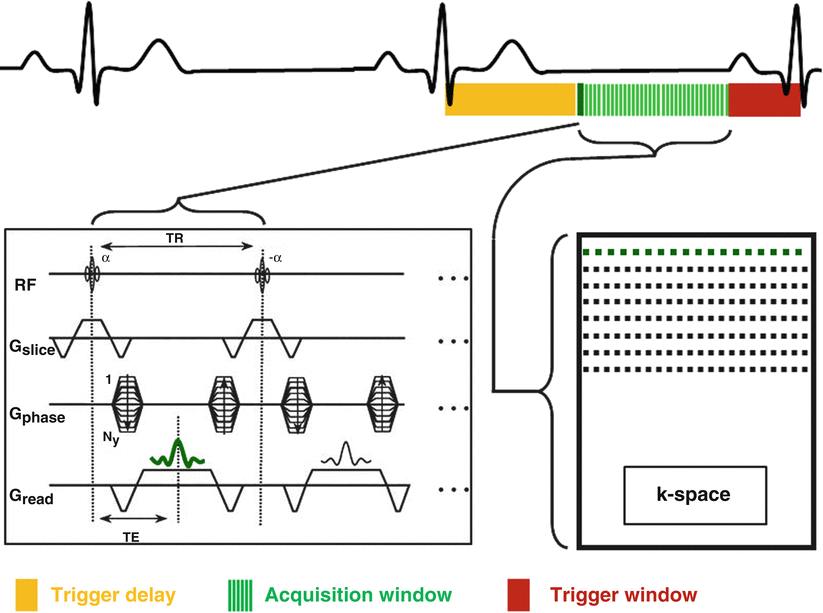
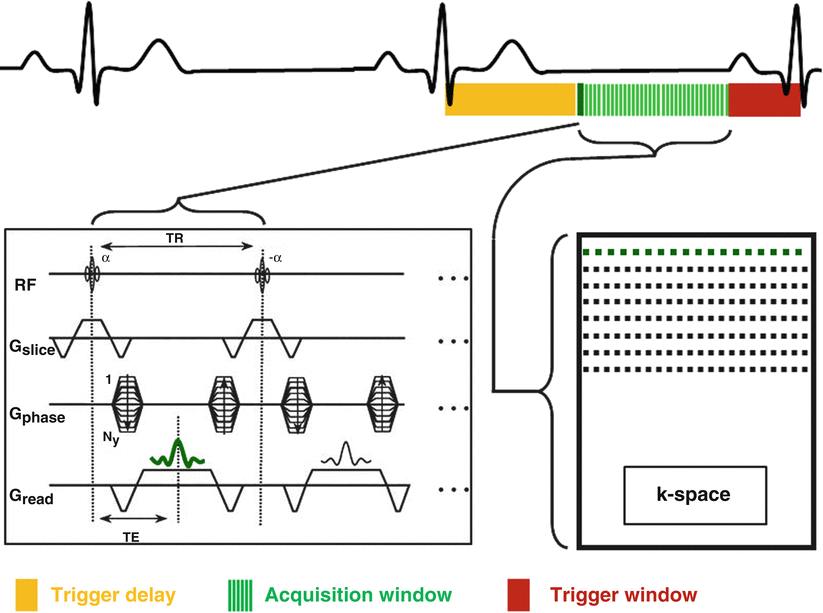
Fig. 9.3
Schematic of a single-shot prospectively-gated sequence. The AW (green bars) is positioned during the diastasis period. It is preceded by a TD (yellow rectangle) and ends at the beginning of the P wave, leaving a TW (red rectangle) to allow detection of the following R-wave. In this case all the data needed to make an image is acquired during a single heart beat. One or multiple slices can be acquired during a breath-hold. AW acquisition window, TD trigger delay, TW trigger delay
Prospectively gated single-shot sequences are used for anatomic or static imaging. Examples include dark-blood half-Fourier turbo spin echo (HASTE) and bright-blood steady-state free precession (SSFP) images which are used as scouts during the initial survey of cardiac exams or to image cardiac anatomy (Fig. 9.4). Single-shot sequences may be used instead of segmented acquisitions for applications such as late gadolinium enhanced imaging (LGE) in patients with arrhythmias or in subjects who cannot perform adequate breath holding to acquire diagnostic segmented images.
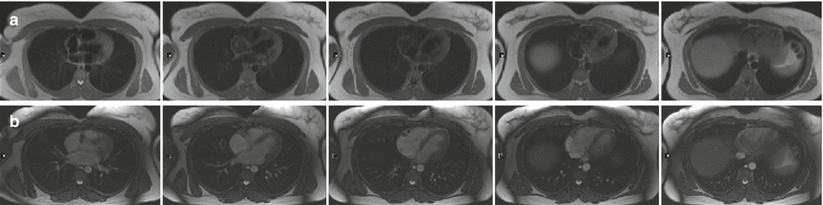
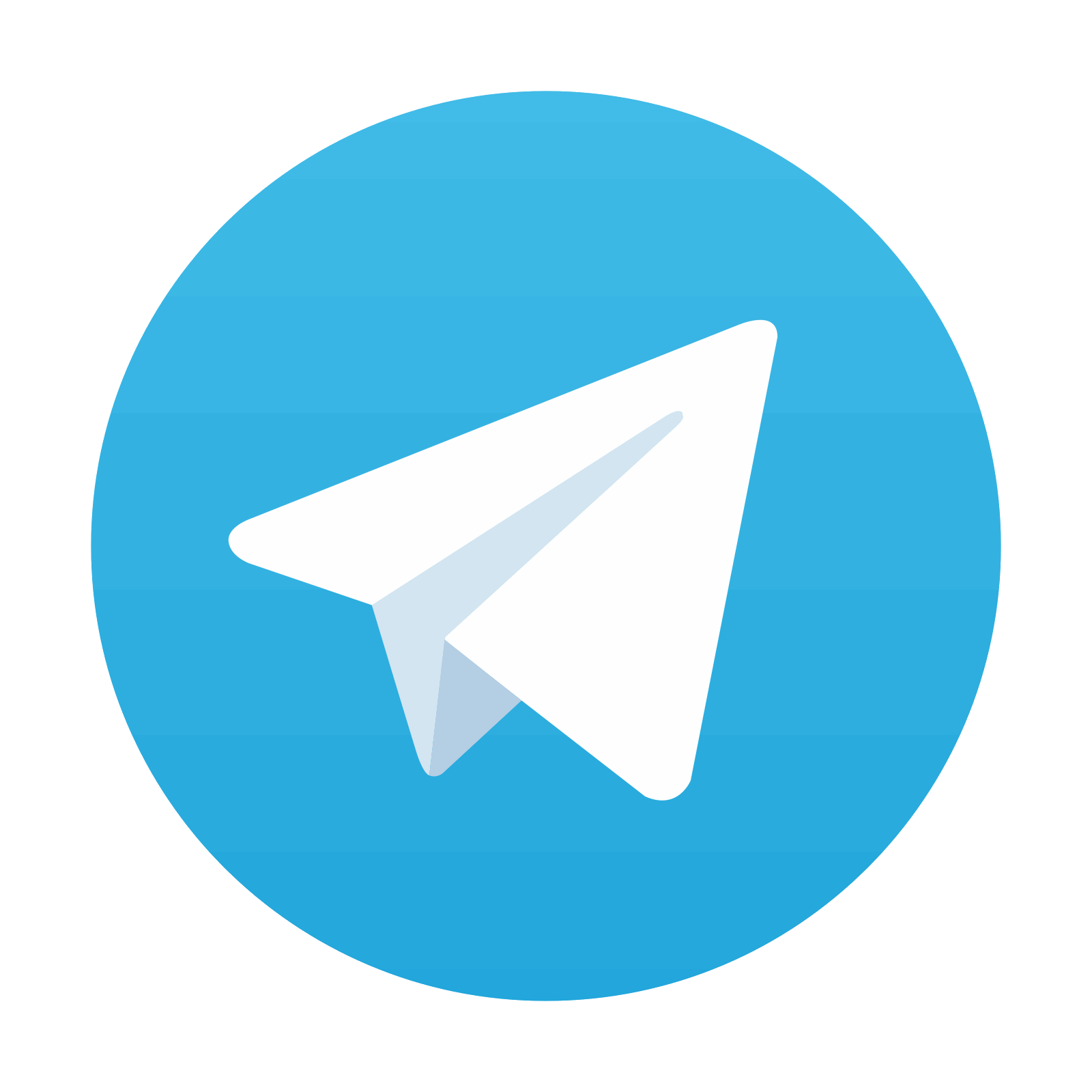
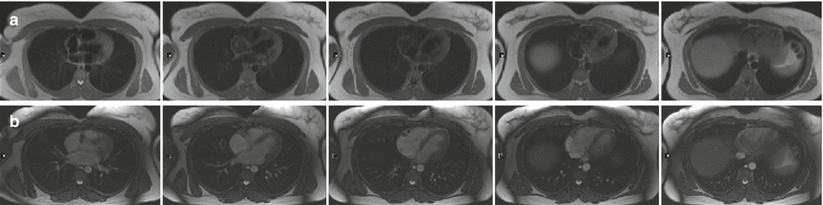
Fig. 9.4
Single-shot HASTE (a) and SSFP (b) axial anatomic images. Multiple slices can be acquired during a single breath-hold. HASTE half-Fourier turbo spin echo, SSFP steady-state free precession
< div class='tao-gold-member'>
Only gold members can continue reading. Log In or Register a > to continue
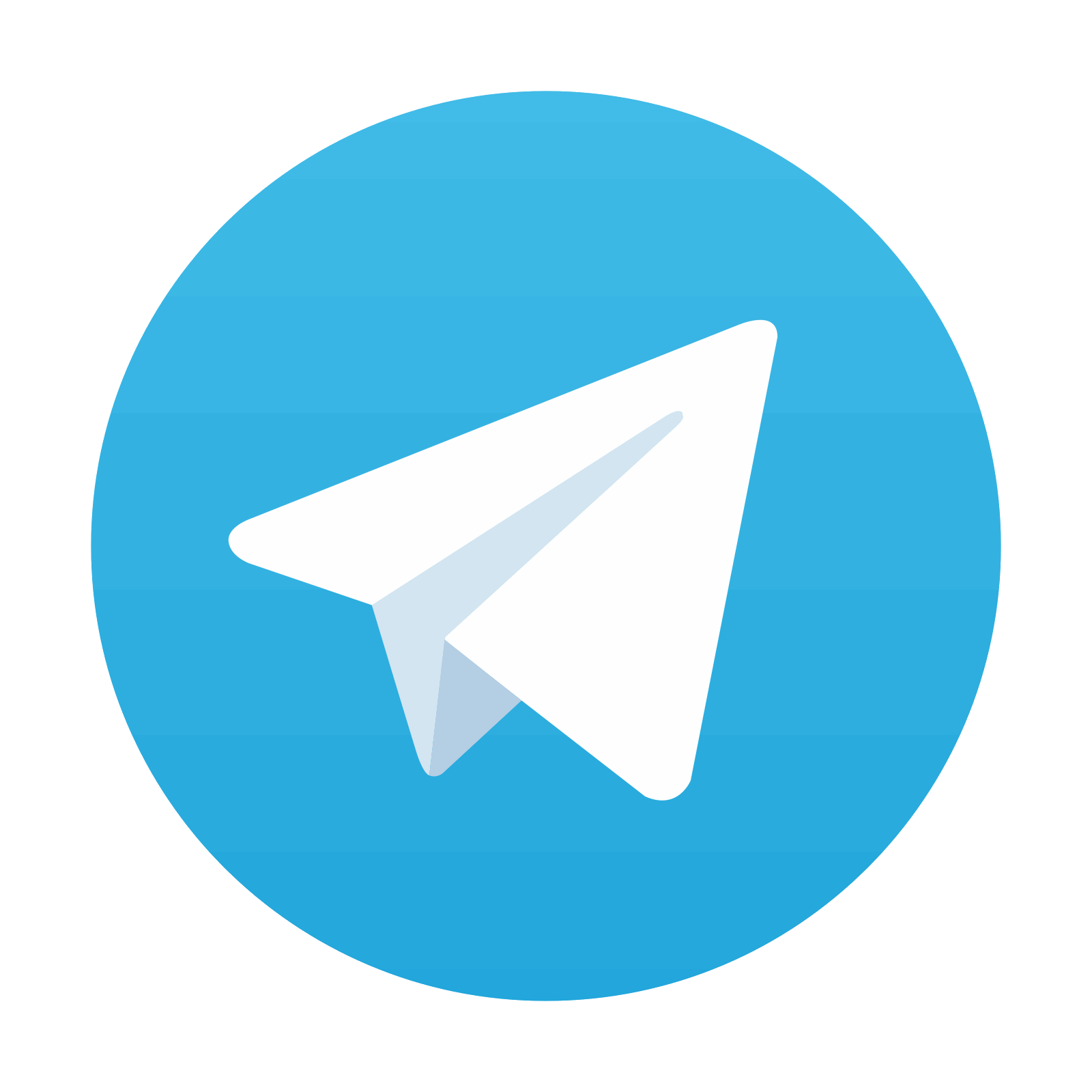
Stay updated, free articles. Join our Telegram channel

Full access? Get Clinical Tree
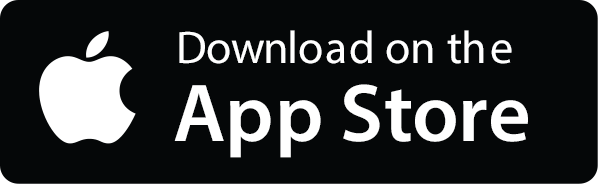
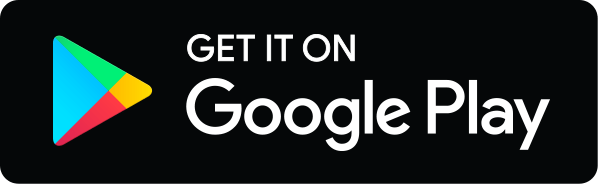
