Background
Preterm birth has been associated with myocardial remodeling and accelerated cardiovascular ageing in later life, but the underlying mechanisms are unknown. The investigators used echocardiography to undertake a sequential analysis of myocardial function in preterm infants.
Methods
This study evaluated the cardiac performance of 25 very preterm infants (born at a gestational age of 26–30 weeks), at birth, 3 months (term-equivalent age), and 6 months later (3 months of corrected age). Speckle-tracking echocardiography was used to determine myocardial function, assessing the magnitude of myocardial deformation as longitudinal strain, deformation rate (strain rate), and velocity in both ventricles during systole and diastole. The results were compared with those in 30 infants born at term investigated at birth and at 3 months of age.
Results
At term-equivalent age, the speckle-tracking estimates were similar in both groups. Three months later, very preterm infants exhibited significantly lower left ventricular mean free wall longitudinal strain (−20.0% vs −22.0%, P = .010) and lower left ventricular early diastolic (median, −7.37 vs −10.9 cm/sec, P = .003) and late diastolic (median, −5.11 vs −6.95 cm/sec, P = .009) myocardial velocities than infants born at term. There were no statistically significant group differences in right ventricular or interventricular septal measurements. Conventional echocardiographic variables did not differ significantly between the two groups at any age.
Conclusions
Very preterm infants develop altered left ventricular myocardial function 6 months after birth. Follow-up examinations are needed to determine the implications for cardiovascular health in the growing number of children surviving very preterm birth.
Each year, approximately 15 million infants are born preterm. Although preterm birth is still a major cause of death worldwide, significant improvements in perinatal care in developed countries mean that it is no longer a fatal pregnancy complication, and a growing number of infants now survive. This development has contributed to an emerging interest in the long-term health and diseases experienced by children and adults who were born preterm.
So far, the significance of preterm birth for cardiac function over the course of a person’s lifetime is largely unknown. It is reassuring that premature birth was not associated with increased mortality from ischemic heart disease at an older age in a study that examined births between 1925 and 1949. However, the neonatal survival rates reported for that historic cohort were very limited and selective. In a more contemporary cohort of young adults, preterm birth was associated with structural and functional changes to the left and right sides of the heart, also after adjusting for other risk factors such as blood pressure. In a large registry study, the adjusted hazard ratio for cardiovascular mortality in 35- to 45-year-old Swedes decreased by 7% with each additional week of gestation. Given that prematurity has also been associated with adult hypertension, diabetes, and stroke, it is important to explore and understand the early effects of preterm birth on cardiac function and development.
To our knowledge, no previous study compared the evolution of myocardial function in preterm and term infants beyond the neonatal period into infancy. One animal study involved the delivery of healthy pregnant sheep before they reached full term gestation to study the effects of prematurity in isolation from selection bias and confounding factors. This intervention resulted in irreversible myocardial remodeling in the preterm lambs, with cardiomyocyte hypertrophy and collagen deposition in both ventricles already observed 9 weeks after term-equivalent age. In two recent human studies, altered diastolic myocardial function was reported only weeks after preterm birth, but follow-up ended before or at term-equivalent age.
Given significant reductions in longitudinal strain and strain rate previously reported in adults born preterm, we hypothesized that such reductions in myocardial function occur in infancy as a consequence of the preterm transition from fetal to neonatal hemodynamics. To test this hypothesis, we used speckle-tracking echocardiography as well as conventional echocardiography to provide serial determinations of cardiac function after very preterm delivery during the first 6 months of postnatal life.
Methods
This observational, longitudinal study measured cardiac function in very preterm infants at birth, at term-equivalent age, and at 3 months of corrected age. For comparison, we studied a healthy control group of term infants at birth and at 3 months of age. Both groups were recruited in parallel, and they represent a randomly selected, convenience sample in which a total of 135 echocardiographic investigations were performed during a study period of 2 years. Parental informed consent was obtained before each examination, and the study protocol was approved by the regional ethical review board in Stockholm, Sweden. All the parents who were invited to take part agreed to participate in the study, and there were no dropouts because of pregnancy or postnatal complications, fetal or infant death, or withdrawal of parental consent.
Subjects
The study group comprised 25 very preterm singleton infants, 13 boys and 12 girls, born at the Karolinska University Hospital in Stockholm. The gestational age of the infants varied between 26 and 30 weeks. According to Swedish sex- and gestational age–specific reference data for normal fetal growth, 21 preterm infants were appropriate for gestational age, with birth weights within ±2 SDs of the mean, and four were small for gestational age, with birth weights of >2 SDs below the mean. There were no cases of chromosomal disorders, congenital syndromes or infections, or malformations.
We included 30 healthy infants born at term with normal birth weights as control subjects. This reference group was recruited at routine antenatal visits in the second trimester at three primary health care maternity clinics in Stockholm.
Gestational age was prospectively determined in all pregnancies by fetal ultrasound examinations at 17 to 18 postmenstrual weeks, according to Swedish recommendations for antenatal care. The parents were interviewed about their family histories of cardiovascular disease, and a positive history was defined as a report of myocardial infarction, stroke, treated hypertension, or hyperlipidemia among the parents’ first-degree relatives.
Umbilical artery blood flow velocity measurements had been performed before delivery, on the basis of clinical indications, in 10 of the 25 pregnancies that ended very preterm, and the pulsatility index was found to be normal in all cases, within ±2 SDs for gestational length. There were no data or measurements on fetal umbilical blood flow in the control subjects born at term.
None of the mothers had diabetes. The most common reasons for premature delivery were premature rupture of membranes ( n = 9), preterm labor ( n = 7), and vaginal bleeding ( n = 7). In addition, one mother needed a cesarean delivery because she developed preeclampsia, and another delivery was medically indicated because the mother had a liver tumor.
The infant and maternal characteristics are presented in Table 1 .
Preterm infants ( n = 25) | Term control infants ( n = 30) | P | |
---|---|---|---|
Maternal data | |||
Age (y) | 32.6 ± 4.2 | 31.1 ± 4.3 | .20 |
Parity | 1.7 ± 1.0 | 1.9 ± 1.0 | .46 |
Family history of CVD | 3/25 (12%) | 5/30 (17%) | .62 |
Smoking in pregnancy | 5/25 (20%) | 1/30 (3.3%) | .048 |
Perinatal data | |||
Gestational age (wk) | 27.7 ± 1.2 | 39.0 ± 1.4 | — |
Boys | 13/25 (52%) | 10/30 (33%) | .16 |
Birth weight (g) | 1,153 ± 258 | 3,456 ± 437 | — |
Birth-weight SDS | −0.82 ± 1.01 | 0.09 ± 1.10 | .003 |
Birth length (cm) | 37.2 ± 2.7 | 50.3 ± 1.9 | — |
Infant data at final assessment | |||
Corrected age (wk) ∗ | 13.7 ± 2.1 | 13.0 ± 1.4 | .13 |
Weight (kg) | 5.61 ± 0.48 | 6.09 ± 0.62 | .003 |
Length (cm) | 59.8 ± 2.5 | 61.2 ± 2.7 | .072 |
Body surface area (m 2 ) † | 0.305 ± 0.018 | 0.321 ± 0.021 | .004 |
Heart rate (beats/min) | 144 ± 17 | 143 ± 15 | .90 |
Systolic blood pressure (mm Hg) | 91 ± 13 | 94 ± 9 | .41 |
Diastolic blood pressure (mm Hg) | 59 ± 11 | 63 ± 12 | .32 |
∗ Corrected age = chronological age reduced by the number of weeks born before 40 weeks of gestation.
Neonatal Characteristics and Morbidity among Very Preterm Infants
In the very preterm group, 22 of 25 infants (88%) received antenatal steroids for induction of lung maturation, and 10 infants (40%) needed ventilator support during their initial hospitalization. Twenty (80%) had umbilical artery catheters inserted after birth, with the catheter tip located in the lower thoracic aorta (Th6–Th10). All umbilical catheters were removed before the first ultrasonographic assessment. Five infants (20%) were pharmacologically treated with ibuprofen for hemodynamically significant patent ductus arteriosus (PDA), but none of the infants needed surgical ligation for this condition. Ten infants (40%) had septicemia; three (12%) had diagnoses of mild intraventricular hemorrhage (grades 1 and 2); four (16%) had bronchopulmonary dysplasia, defined as needing supplementary oxygen at 36 weeks of postmenstrual age; and one infant (4%) had retinopathy of prematurity stage 1. There were no cases of necrotizing enterocolitis.
Study Protocol
In accordance with recommendations of the American Academy of Pediatrics, participants’ age is reported as “postmenstrual age” (defined as gestational age + chronological age in weeks) in the perinatal period (ending 1 week after term). After the perinatal period, age in preterm infants is reported as “corrected age” (defined as chronological age reduced by the number of weeks born before 40 weeks of gestation).
The study protocol consisted of carrying out three consecutive echocardiographic assessments of the preterm infants and two in the term group. The preterm infants had their first ultrasound examinations at an average postmenstrual age of 28 weeks and were then reexamined at term-equivalent age. Finally, the preterm infants were investigated at a mean corrected age of 13.7 ± 2.1 weeks. In the term control group, the first ultrasound assessment was carried out in the first week of postnatal life and the second at 13.0 ± 1.4 weeks of age ( P = .13 for group difference) ( Figure 1 ).

Ultrasonographic Assessments
All recordings were performed without sedation by one experienced examiner (U.S.) using the same ultrasound machine (Vivid 7; GE Vingmed Ultrasound AS, Horten, Norway) and the same phased-array sector probe (GE 10-S; 4.0–10.5 MHz). A complete diagnostic echocardiographic assessment was performed on all subjects to rule out structural heart defects other than PDA or patent foramen ovale. Persistent pulmonary hypertension of the newborn, in this study defined as right ventricular (RV) systolic pressure > 30 mm Hg, was also ruled out at the first examination. RV systolic pressure was estimated from physiologic tricuspid regurgitation in all cases.
For each examination, digital loops containing at least five heart cycles in a B-mode four-chamber projection were acquired on high-quality two-dimensional imaging and at frame rates ranging between 176 and 200 frames/sec (mean, 187 frames/sec) in the preterm infants and between 168 and 205 frames/sec (mean, 190 frames/sec) in the term infants. In examinations with frame rates initially <160 frames/sec in the four-chamber projection ( n = 25 of 135 [18% of investigations]), we reduced sector width to visualize the left and right ventricles and the corresponding atria separately without rotating the transducer. Accordingly, the speckle-tracking outcomes were analyzed in the lateral walls of the left and right ventricles. No harmonic imaging was used.
All recordings were evaluated offline by an independent and blinded examiner (M.M.) using commercially available software (EchoPAC; GE Healthcare, Milwaukee, WI, USA). A semiautomatic system traced the myocardium-endocardium border of each ventricle in a separate analysis, marking six regions of interest (ROIs). Measurements of the three ROIs of the interventricular septum (IVS) were performed as part of the left ventricular (LV) analyses and are reported separately ( Figure 2 ). For each outcome variable and each ROI, three measurements were performed, and the mean value was calculated. When necessary, the tracking process was visually optimized by the examiner, as the midpoint of the endocardial tracking line was not necessarily the anatomic apex of the respective ventricle. We measured myocardial deformation variables as longitudinal strain, strain rate, and maximum myocardial velocities. Longitudinal strain and strain rate measurements were performed at the basal (atrioventricular annulus), medial, and apical levels in the lateral walls of the left and right ventricles and the IVS. Mean strain rate values were calculated from basal, medial, and apical values. Myocardial velocities were measured only at the level of the atrioventricular annulus (basal level). The underlying software measured velocity by the derivation of speckle displacement per time unit and Lagrangian strain by the temporal integration of strain rate, where the peak longitudinal strain was defined as the relationship between the initial ( L 0 ) and final ( L ) maximal length of the RV and LV walls: strain = ( L − L 0 )/ L 0 .

The intraobserver coefficient of variation for speckle-tracking outcomes in infants has previously been found to be 5.8% and the interobserver coefficient of variation to be 6.5% in our echocardiographic laboratory.
Calculation of Cardiac Indices
The myocardial performance index (MPI) was assessed using pulsed-wave Doppler echocardiography. MPI was calculated as the sum of the isovolumetric contraction and relaxation times by subtracting ventricular ejection time from the interval between cessation and onset of atrioventricular inflow and by dividing the sum of both isovolumetric intervals by ejection time: MPI = (isovolumetric contraction time + isovolumetric relaxation time)/ejection time.
The relation between early diastolic filling velocities as measured by the conventional pulsed-wave Doppler technique (E) and by the speckle-tracking technique (E′) was represented by the E/E′ ratio. The relationship between early (E) and late (A) diastolic filling was expressed as the E/A ratio for the conventional pulsed-wave Doppler technique and E′/A′ ratio for the speckle-tracking technique.
Stroke volume (SV) and cardiac output were measured with the help of Doppler-derived flow velocity integrals according to the following formulas: SV = velocity-time integral × valve area, and cardiac output = SV × heart rate.
In addition, ejection fraction was estimated using the modified Simpson rule (biplane) and shortening fraction in M mode, calculating the percentage change in LV diameter during systole.
Finally, LV mass (LVM) at 3 months of corrected age was calculated according to the following formula: LVM = 1.04[(LV end-diastolic dimension + interventricular septal dimension + LV posterior wall dimension) 3 − (LV end-diastolic dimension) 3 ]. Values for LVM were adjusted for body surface area (calculated according to the Mosteller formula ).
Statistical Analysis
Data are presented as mean ± SD, median (interquartile range), or numbers and percentages, depending on the type and distribution of data. The Shapiro-Wilk test was used to test whether data were normally distributed (longitudinal strain) or not (strain rate and myocardial velocity). For normally distributed data, Student’s t test was used to investigate intergroup differences, and the paired t test was used to analyze intragroup differences from term to 3 months of corrected age. For data without normal distribution, the Mann-Whitney U test was used to test for intergroup differences and the Wilcoxon signed rank test to test for intragroup differences, from term to 3 months of corrected age.
The primary objective of this study was to compare myocardial function in preterm and term infants, particularly if group differences in myocardial deformation in the left or right ventricle developed over time. Speckle-tracking variables, such as mean free wall longitudinal strain, strain rate, and myocardial velocity at the end point of our study, were considered as primary outcomes and conventional echocardiographic measurements and calculated indices as secondary outcomes.
We considered maternal smoking during pregnancy, birth-weight SD score, and weight at 3 months of corrected age as potential confounders or covariates. Therefore, we tested for any associations between these covariates and myocardial function at the final examination (at 3 months of corrected age). In the very preterm group, we also tested for intragroup differences in cardiac outcomes at the last examination in relation to selected neonatal characteristics and morbidities that may have had an impact on cardiac function 6 months after birth: antenatal steroid exposure (a potential confounder), gestational age (shown to exhibit a dose-response relationship to adult cardiac structure), a neonatal diagnosis of PDA (known to impose a left-sided volume load on the heart in the neonatal period), and bronchopulmonary dysplasia (associated with increased pulmonary vascular resistance).
The sample-size was based on the calculation that with 25 infants in each group, we would be able to detect a group difference in any outcome variable of ≥0.8 SDs, using a two-sided t test with an α level of 0.05 and power of 0.80. To reduce the risk for a type I statistical error due to multiple testing, the α levels after Bonferroni correction were set to 0.017 for the three speckle-tracking outcomes, 0.012 for the four RV outcomes, and 0.006 for the eight LV outcomes using conventional echocardiography.
All data were analyzed using JMP version 11.0.0 (SAS Institute, Cary, North Carolina).
Results
Success rates for speckle-tracking echocardiographic measurements in the left ventricle and IVS varied between 96% and 100% and in the right ventricle between 82% and 96%. The lowest success rate occurred at the last examination at 3 months of age, mainly because of movement artifacts.
Longitudinal Myocardial Strain
At term-equivalent age, there were no significant differences in LV or RV mean free wall strain or IVS strain between the preterm and term infants.
In term infants but not in preterm infants, there was a significant increase in LV free wall strain from birth to 3 months of age. There were no other statistically significant age-related intragroup changes in strain.
At 3 months of corrected age, mean free wall LV strain was significantly lower in the preterm infants ( P = .010), whereas IVS and RV mean free wall strain did not differ significantly from the values found in infants born at term ( Table 2 , Figure 3 A).
ROI | Postmenstrual age (wk) | P value for difference between groups at 53 wk | |||||
---|---|---|---|---|---|---|---|
28 | 40 | 53 | |||||
Preterm | Control | Preterm | Control | Preterm | Control | ||
Left ventricle | |||||||
Basal | −18.6 ± 3.5 | — | −19.3 ± 4.6 | −20.0 ± 3.8 | −19.8 ± 4.2 | −22.1 ± 3.8 | .03 |
Medial | −17.5 ± 3.2 | — | −18.1 ± 3.4 | −19.1 ± 2.6 | −19.8 ± 3.4 | −21.7 ± 3.8 | .05 |
Apical | −17.5 ± 4.2 | — | −18.7 ± 3.3 | −19.4 ± 2.8 | −20.5 ± 3.4 | −22.2 ± 4.5 | .12 |
Mean | −17.9 ± 2.5 | — | −18.7 ± 2.6 | −19.5 ± 2.1 | −20.0 ± 2.2 | −22.0 ∗ ± 3.2 | .010 |
IVS | |||||||
Basal | −18.4 ± 3.6 | — | −20.4 ± 4.1 | −20.6 ± 3.7 | −21.1 ± 2.8 | −21.7 ± 3.3 | .48 |
Midseptal | −18.8 ± 3.4 | — | −20.2 ± 3.1 | −19.6 ± 3.5 | −22.1 ± 2.5 | −22.2 ± 3.4 | .86 |
Apical | −19.8 ± 3.8 | — | −20.3 ± 3.0 | −19.9 ± 3.7 | −22.5 ± 3.0 | −23.6 ± 4.7 | .36 |
Mean | −19.0 ± 3.4 | — | −20.3 ± 3.0 | −20.1 ± 3.4 | −21.9 ± 2.2 | −22.5 ∗ ± 3.3 | .44 |
Right ventricle | |||||||
Basal | −20.7 ± 8.9 | — | −24.7 ± 7.6 | −25.3 ± 7.3 | −25.2 ± 6.7 | −28.0 ± 7.1 | .17 |
Medial | −20.0 ± 6.2 | — | −24.0 ± 4.7 | −22.6 ± 4.7 | −24.2 ± 4.3 | −23.9 ± 6.0 | .86 |
Apical | −21.0 ± 4.9 | — | −21.4 ± 4.7 | −21.2 ± 5.0 | −22.2 ± 7.2 | −21.4 ± 5.8 | .69 |
Mean | −20.5 ± 5.5 | — | −23.3 ± 4.0 | −23.0 ± 4.2 | −23.9 ± 4.2 | −24.4 ± 5.2 | .69 |
∗ P < .05 for within-group difference from 40 to 53 weeks of postmenstrual age.
Strain Rate
There were no intergroup differences in strain rate at term-equivalent age, with the exception of a higher RV systolic strain rate in preterm infants than in term infants.
In term infants, there was a significant increase in all LV, IVS, and RV strain rates from birth to 3 months of age. In preterm infants, there was a significant increase in LV systolic, IVS, and RV early diastolic strain rates from term-equivalent age to 3 months of corrected age.
At 3 months of corrected age, there were no intergroup differences in LV, IVS, or RV strain rates ( Table 3 ).
Postmenstrual age (wk) | P value for difference between groups at 53 wk | ||||||
---|---|---|---|---|---|---|---|
28 | 40 | 53 | |||||
Preterm | Control | Preterm | Control | Preterm | Control | ||
Left ventricle | |||||||
Systole | −2.33 (−2.9 to −2.1) | — | −2.60 (−3.5 to −2.3) | −2.40 (−3.2 to −2.0) | −3.37 ∗ (−3.8 to −2.7) | −3.06 ∗ (−3.7 to −2.6) | .53 |
Early diastole | 2.78 (2.4 to 3.5) | — | 3.51 (3.1 to 3.7) | 3.05 (2.7 to 4.0) | 3.33 (3.1 to 5.1) | 4.33 ∗ (3.5 to 5.3) | .28 |
Late diastole | 2.28 (1.8 to 2.9) | — | 2.76 (2.0 to 3.3) | 2.10 (1.6 to 3.2) | 2.52 (2.1 to 3.6) | 3.22 ∗ (2.2 to 3.9) | .28 |
IVS | |||||||
Systole | −2.12 (−2.6 to −1.8) | — | −2.32 (−2.8 to −1.9) | −2.08 (−2.4 to −1.7) | −2.62 (−3.2 to −2.0) | −2.60 ∗ (−3.1 to −2.2) | .75 |
Early diastole | 2.72 (2.0 to 3.1) | — | 2.62 (2.2 to 3.2) | 2.32 (2.0 to 2.9) | 3.22 ∗ (2.9 to 4.3) | 3.44 ∗ (2.6 to 4.3) | .93 |
Late diastole | 2.45 (1.8 to 2.9) | — | 2.47 (1.9 to 2.9) | 1.88 (1.5 to 2.4) | 2.56 (2.0 to 3.0) | 2.57 ∗ (2.2 to 3.4) | .23 |
Right ventricle | |||||||
Systole | −2.79 (−3.2 to −2.6) | — | −3.81 (−4.1 to −3.1) | −2.70 (−3.7 to −2.3) | −4.17 (−4.7 to −2.8) | −3.79 ∗ (−5.3 to −3.3) | .38 |
Early diastole | 3.20 (2.3 to 3.5) | — | 3.59 (2.8 to 4.5) | 3.00 (2.3 to 4.3) | 4.54 ∗ (3.9 to 5.5) | 4.16 ∗ (2.9 to 6.1) | .82 |
Late diastole | 2.64 (2.1 to 3.1) | — | 3.33 (2.5 to 4.2) | 2.30 (1.7 to 3.0) | 2.90 (2.2 to 4.1) | 3.53 ∗ (2.7 to 4.3) | .38 |
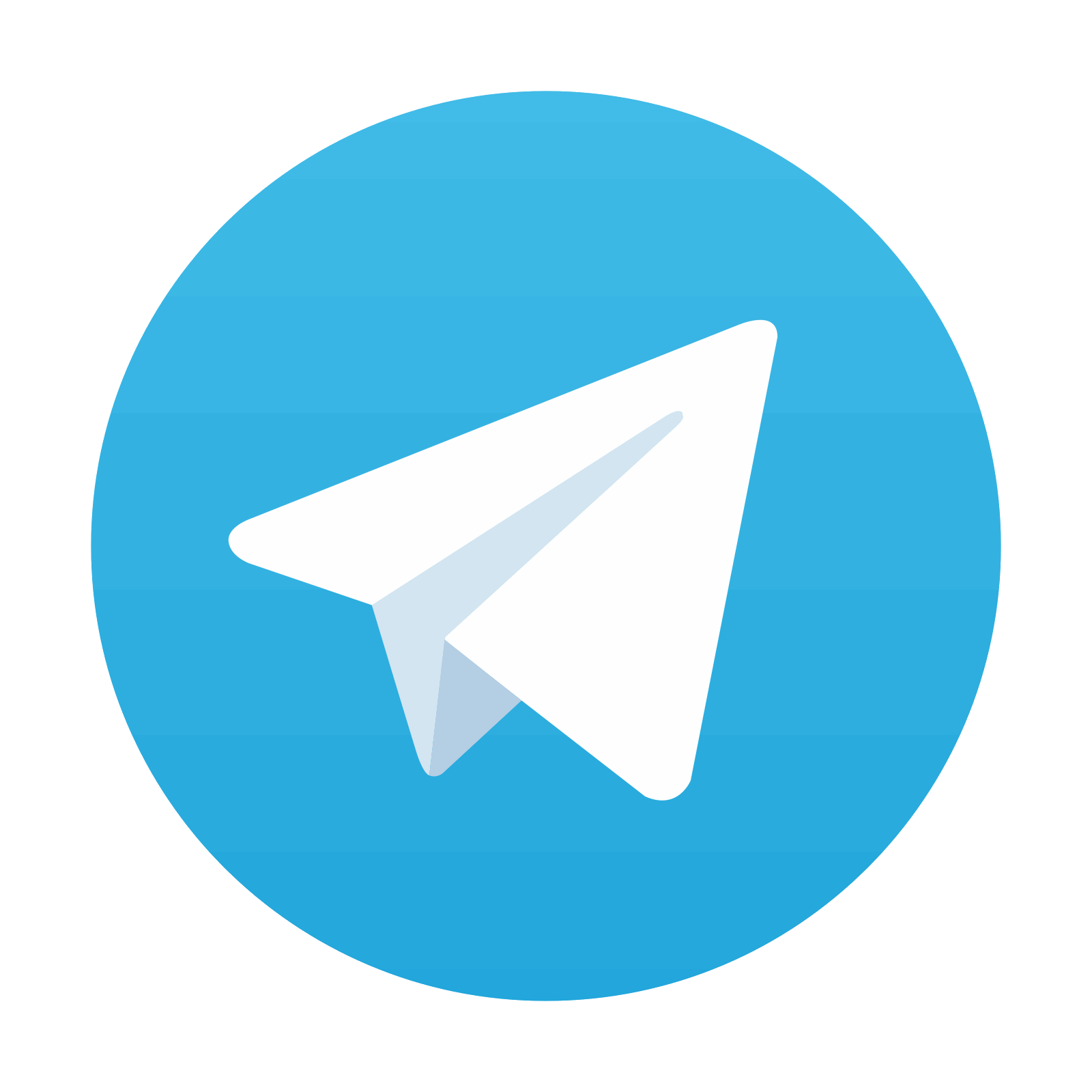
Stay updated, free articles. Join our Telegram channel

Full access? Get Clinical Tree
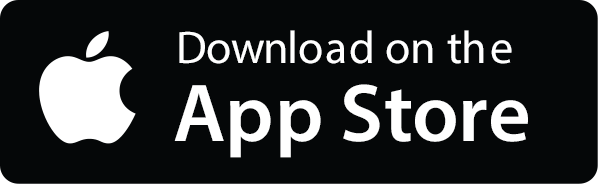
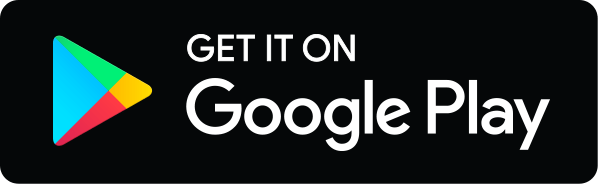
