Background
The pressure-volume relationship (PVR) is a useful method for evaluating left ventricular (LV) myocardial contractility during dobutamine stress echocardiography (DSE). The investigators assessed PVRs, systolic and diastolic function, B-type natriuretic peptide (BNP) levels, and aerobic exercise capacity in patients with congestive heart failure (CHF).
Methods
A total of 84 patients with CHF (mean age, 68 ± 9 years) underwent high-dose DSE. PVR was defined as the systolic cuff pressure/end-systolic volume index difference between rest and peak DSE. Of these, 67 patients also underwent cardiopulmonary exercise testing. The patients were divided into 3 equal groups on the basis of PVR: lower, intermediate, and higher.
Results
PVRs were significantly lower in more symptomatic patients (New York Heart Association class II vs III) (2.17 ± 1.99 vs 0.91 ± 0.72 mm Hg/mL/m 2 , P < .001). Patients with elevated LV filling pressures (E/Ea ≥ 14) showed significantly lower PVRs compared with patients with normal or slightly abnormal LV filling pressures (1.1 ± 1.1 vs 2.96 ± 3.11 mm Hg/mL/m 2 , P = .006). Patients with higher PVRs showed lower logarithmically transformed BNP levels (2.0 ± 0.5, 2.5 ± 0.3, and 2.6 ± 0.5 pg/mL; P < .001), higher peak oxygen consumption (17 ± 4, 13 ± 3, and 12 ± 3 mL/kg/min; P < .001), and higher rest-stress percentage changes in ejection fraction (80 ± 50%, 56 ± 29%, and 22 ± 32%; P < .001) compared with patients with intermediate and lower PVRs, respectively. The parameters predictive of exercise tolerance were PVR (odds ratio [OR]; 1.582, 95% confidence interval [CI], 1.136-2.204; P = .007), ejection fraction (OR, 1.172; 95% CI, 1.070-1.283; P = .001), log BNP (OR, 0.080; 95% CI, 0.020-0.325; P < .001), and E/Ea (OR, 0.836; 95% CI, 0.733-0.953; P = .007).
Conclusions
In patients with CHF, impaired noninvasively assessed myocardial contractility is related to impaired systolic and diastolic function, higher BNP levels, and poorer exercise tolerance.
Dobutamine stress echocardiography (DSE) is widely used in patients with congestive heart failure (CHF) to search for myocardial viability in ischemic cardiomyopathy and to evaluate myocardial contractile reserve in idiopathic cardiomyopathy. Moreover, it has also been shown that the presence of an inotropic response elicited by pharmacologic stress echocardiography identifies a subset of patients at lower risk for death. However, there is no consensus on which index should be used in clinical practice.
The assessment of the force-frequency relationship is a theoretically robust approach for evaluating left ventricular (LV) contractility, which has been used clinically by using invasive, complex, and technically demanding methods. Recently, a noninvasive echocardiographic method has been proposed to assess changes in contractility during exercise echocardiography.
The pressure-volume relationship (PVR), expressed as the difference between systolic pressure (SP)/end-systolic volume index (ESVI) at rest and during peak stress or as the slope of SP/ESVI at different heart rates, may be assessed during semisupine exercise, external pacing echocardiography in patients with permanent pacemakers, and DSE. During exercise and DSE, the end-systolic pressure-volume ratio depends on the force-frequency relationship and inotropic stimulation. In previous studies, abnormal stress-induced variation in the end-systolic pressure-volume ratio was found to be associated with adverse outcomes in unselected series of patients with negative results on exercise stress echocardiography and DSE.
Patients with diabetes mellitus who have negative results on DSE may still experience adverse outcomes, which can be identified by the difference between rest and peak DSE, providing incremental prognostic stratification over that supplied by regional wall motion abnormalities.
The aim of our study was to evaluate the relationships between SP/ESVI at rest and during peak stress (PVR), systolic and diastolic function, B-type natriuretic peptide (BNP) levels, and aerobic exercise capacity in patients with CHF.
Methods
Study Patients
In a prospective multicenter study design, from 2004 to 2008, 94 patients were prospectively, nonconsecutively enrolled from the Division of Cardiology at Fatebenefratelli Hospital (Benevento, Italy) (n = 65) and the Institute of Clinical Physiology at the National Council of Research (Pisa, Italy) (n = 29). The study population consisted of patients with CHF with LV ejection fractions < 45% on echocardiography who had typical symptoms of heart failure that were classified according to New York Heart Association (NYHA) functional class. All patients had transthoracic echocardiograms adequate for assessing resting regional wall function (an echocardiogram were considered adequate if >13 of the maximum of 17 segments were visualized in at least one projection). Exclusion criteria were (1) a technically poor acoustic window precluding satisfactory imaging of left ventricle (for two-dimensional echocardiography), (2) hemodynamic instability, (3) documentation of life-threatening ventricular arrhythmias (sustained ventricular tachycardia or ventricular fibrillation), (4) significant comorbidity reducing life expectancy to <1 year, and (5) unwillingness to give informed consent.
Of the 94 patients initially selected for the study, 10 patients were subsequently excluded for inadequate echocardiographic image quality during stress because of inadequate wall motion analysis. Thus, 84 patients (63 men; mean age, 68 ± 9 years) constituted the final study group. The study was approved by the institutional review board. All patients gave written informed consent when they underwent stress echocardiography. When patients signed the consent forms, they also authorized physicians to use their clinical data according to Italian law.
All patients were in sinus rhythm and on optimal and maximal tolerated pharmacological therapy, according to current guidelines for treatment of heart failure. Ongoing medical therapy was kept unchanged at the time of the stress test. No patients had implantable cardioverter-defibrillators or pacemakers.
Forty-seven patients (56%) had ischemic heart disease, with histories of myocardial infarctions. No patients with ischemic cardiomyopathy during DSE showed the presence of stress-induced ischemia and/or a biphasic response: the presence of an ischemic response represented an exclusion criterion. Coronary angiography was performed in all patients. All patients with ischemic cardiomyopathy had coronary anatomy unsuitable for further revascularization.
Clinical information was collected on the case report form at study entry by the accredited cardiologist-echocardiographer at each center. Resting and stress echocardiographic data were obtained at each recruiting center by cardiologist-sonographers accredited by the European Association of Echocardiography who had passed the quality control for stress echocardiography reading, as previously described.
All images digitally stored were independently read and reviewed by a core laboratory for wall motion (Institute of Clinical Physiology, Pisa, Italy). All investigators were instructed to obtain all the standard stress echocardiographic projections both for wall motion assessment; images were digitally stored at rest, at peak dobutamine stress, and after dobutamine discontinuation or β-blocker infusion, when needed. The digital images obtained were sent to the core laboratory on the Web, together with the investigator’s stress echocardiography report. Briefly, the reader at each recruiting center met the predefined criteria for stress echocardiography reading. At that point, the center could start recruiting patients, and readings of the stress echocardiograms from the recruiting center were then directly sent to the core labs.
All measurements were obtained following the recommendations of the American Society of Echocardiography at baseline and during follow-up. In particular, LV volumes and ejection fraction were assessed from 2-dimensional, 2-chamber and 4-chamber views by biplane area.
Diastolic function was assessed using transmitral patterns and tissue Doppler. Pulsed mitral Doppler measurements were obtained with the transducer in the apical 4-chamber view by positioning a 1-mm to 2-mm sample volume between the tips of the mitral valve leaflets in diastole, with the Doppler beam aligned perpendicular to the plane of the mitral annulus. We derived early peak filling velocity (E), late peak filling velocity (A), the early-to-late filling ratio, and the deceleration time of the mitral E wave.
Mitral regurgitation was classified according to the recommendations of the American Society of Echocardiography.
Tissue Doppler was performed in pulsed-wave Doppler mode. Gain and filters were adjusted as needed to eliminate background noise and to allow for a clear tissue signal. The tissue Doppler signals were recorded at a sweep speed of 100 mm/s. From the apical 4-chamber view, a 5-mm sample volume was placed at the lateral and septal corners of the mitral annulus. The following measurements were made: peak systolic velocity (Sa), early (Ea) and late (Aa) diastolic velocities at the mitral annulus, and the ratio between early-wave mitral flow and mitral annular early diastolic velocity (E/Ea). E/Ea and Sa were analyzed as mean values of lateral and septal corners. Measurements were made over 3 to 5 cardiac cycles and averaged.
Stress Echocardiographic Protocol
All patients underwent high-dose DSE, and dobutamine was infused in 3-minute dose increments, starting from 5 μg/kg/min and increasing to 10, 20, 30, and 40 μg/kg/min. Wall motion score index (1 = normal to 4 = dyskinetic in a 17-segment model of the left ventricle) was assessed following the recommendations of the European Association of Echocardiography.
During the procedure, blood pressure and the electrocardiogram were recorded each minute. Nonechocardiographic criteria for ending the test were peak dobutamine dose, the achievement of 85% of the target heart rate (determined according to the equation predicted target heart rate = 220 − age), and severe chest pain. The test was also halted in case of intolerable symptoms or limiting side effects, including hypertension (systolic blood pressure > 220 mm Hg, diastolic blood pressure > 120 mm Hg), hypotension (relative or absolute; > 30 mm Hg decrease in blood pressure), supraventricular arrhythmias (supraventricular tachycardia or atrial fibrillation), ventricular arrhythmias (ventricular tachycardia, frequent, polymorphous premature ventricular beats), and bradyarrhythmias. A maximal test was defined by the achievement of 85% of age-predicted maximal heart rate. We assessed 5 beats at each stage analyzed (rest and peak DSE).
PVR Assessment
The PVR was measured as the difference between the value at rest and the value at peak DSE of the ratio between SP (evaluated by aneroid cuff sphygmomanometer) and LV end-systolic volume normalized to body surface area (ESVI). End-systolic volume was obtained offline from apical 4-chamber and 2-chamber views using the biplane Simpson method. Only representative cycles with optimal endocardial visualization were measured, and the average of 3 measurements was taken. The endocardial border was traced, excluding the papillary muscles. The frame with the smallest LV cavity was considered to be the end-systolic frame. We divided the patients into 3 equal groups on the basis of PVR: lower (mean, 0.18 ± 0.27 mm Hg/mL/m 2 ; range < 0.48 mm Hg/mL/m 2 ), intermediate (mean, 1.02 ± 0.27 mm Hg/mL/m 2 ; range, 0.49-1.41 mm Hg/mL/m 2 ), and higher (mean, 4.02 ± 2.88 mm Hg/mL/m 2 ; range > 1.42 mm Hg/mL/m 2 ).
Measurement of Plasma BNP Levels
In all patients, blood was sampled from the antecubital vein following 20 minutes of supine rest before DSE. The peripheral venous blood was collected into a sampling tube containing ethylenediaminetetraacetic acid as an anticoagulant. We used a rapid bedside test for the determination of BNP (Triage BNP; Biosite Diagnostics, San Diego, CA). The Triage BNP test is an immunofluorometric assay for the quantitative determination of BNP in whole blood or plasma anticoagulated with ethylenediaminetetraacetic acid.
Exercise Test
Within 24 hours of DSE, 67 of 84 patients (80%) underwent treadmill cardiopulmonary exercise tests according to the modified Bruce protocol, with expired gas measurement. Seventeen patients were excluded from the cardiopulmonary exercise tests because of difficulty carrying out the treadmill exercise. Oxygen uptake, carbon dioxide production, and minute ventilation were measured using breath-by-breath gas analysis. A 12-lead electrocardiogram was continuously recorded, and blood pressure was recorded every minute using a cuff sphygmomanometer. Peak oxygen uptake was determined as the highest value at the end of exercise.
Statistical Analysis
Data are expressed as mean ± SD for continuous variables and as number (percentage) for categorical variables. Logarithmic transformation was performed on BNP values because of their nonlinear distribution. Univariate analysis was performed to compare the differences between PVR with continuous variables, using Pearson’s coefficient.
We divided the patients into 3 equal groups on the basis of PVR: lower, intermediate, and higher. The differences between groups were analyzed using one-way analysis of variance for repeated measures. If any interactions were significant, post hoc comparisons were performed using unpaired t tests with Bonferroni’s correction to detect differences between two groups.
A P value < .05 was considered statistically significant. All statistical calculations were performed using SPSS for Windows version 12.0 (SPSS, Inc, Chicago, IL).
Results
Patient Characteristics
The clinical and echocardiographic characteristics of the study population are described in Table 1 . The mean LV ejection fraction was 29 ± 7%. Twenty-seven patients (32%) were in NYHA class II, and 57 (68%) were in NYHA class III. The cause of LV systolic dysfunction was ischemic heart disease in 47 patients (56%).
Variable | Value |
---|---|
Age (y) | 68 ± 9 |
Men | 63 (75%) |
NYHA class > II | 57 (68%) |
Left atrial diameter (mm) | 48 ± 5 |
LV end-diastolic diameter (mm) | 65 ± 7 |
LV end-systolic diameter (mm) | 55 ± 8 |
LV end-diastolic volume (mL) | 158 ± 55 |
LV end-systolic volume (mL) | 115 ± 47 |
LV ejection fraction at rest (%) | 29 ± 7 |
WMSI at rest | 2.35 ± 0.28 |
Restrictive filling pattern | 11 (13%) |
Mitral regurgitation ≥ moderate | 30 (36%) |
E/Ea ratio | 15 ± 8 |
Peak mitral annular systolic velocity (cm/s) | 4.5 ± 1.2 |
BNP (pg/mL) | 419 ± 465 |
Log BNP (pg/mL) | 2.4 ± 0.5 |
Peak oxygen consumption (mL/kg/min) | 14 ± 4 |
Feasibility and Tolerability of High-Dose DSE
All the patients reached the maximal dose of DSE (up to 40 μg/kg/min). During dobutamine infusion, nonsustained ventricular tachycardia was observed in 7 patients (8%) and hypotension in 1 patient. These complications were always well tolerated, and they did not cause premature interruption of the test. Hemodynamic and echocardiographic responses to dobutamine stress are described in Table 2 . Sixteen patients (19%) achieved maximal DSE.
Variable | Rest | Stress | Change | P |
---|---|---|---|---|
Heart rate (beats/min) | 67 ± 12 | 103 ± 22 | +54% | <.001 |
Systolic blood pressure (mm Hg) | 117 ± 20 | 128 ± 28 | +10% | <.001 |
Double product | 7839 ± 1743 | 13,145 ± 3923 | +68% | <.001 |
LV end-diastolic volume (mL) | 158 ± 55 | 143 ± 58 | −10% | <.001 |
LV end-diastolic volume (mL) | 115 ± 47 | 85 ± 52 | −26% | <.001 |
LV ejection fraction (%) | 29 ± 7 | 44 ± 15 | +52% | <.001 |
WMSI | 2.35 ± 0.28 | 2.00 ± 0.44 | −15% | <.001 |
PVR (mm Hg/mL/m 2 ) | 2.1 ± 0.9 | 3.9 ± 2.9 | +86% | <.001 |
Interobserver variability of LV end-systolic volume measurements by two independent sonographers showed excellent correlation ( r = .89, P < .01), with no significant difference at rest (0.7 ± 22 mL; 95% confidence interval [CI], −43.7 to 45.2 mL; P = .80) and during peak stress (5.4 ± 12 mL; 95% CI, −19.4 to 30.2 mL; P = .40).
PVR and Clinical Parameters
PVRs were significantly lower in symptomatic patients in NYHA class > II (2.17 ± 1.99 vs 0.91 ± 0.72 mm Hg/mL/m 2 , P < .001; Figure 1 ). PVR was inversely related to log BNP levels ( r = −0.557, P < .001; Table 3 ).

Variable | SP/ESVI at Rest | SP/ESVI at Peak Stress | PVR | |||
---|---|---|---|---|---|---|
Rest | r | P | r | P | r | P |
LV end-diastolic volume | −0.754 | <.001 | −0.497 | <.001 | −0.335 | .002 |
LV end-systolic volume | −0.820 | <.001 | −0.592 | <.001 | −0.418 | <.001 |
LV ejection fraction | 0.678 | <.001 | 0.605 | <.001 | 0.498 | <.001 |
WMSI | −0.384 | .003 | −0.399 | .019 | −0.360 | .005 |
E/Ea ratio | −0.325 | .011 | −0.354 | .006 | −0.326 | .011 |
Peak mitral annular systolic velocity | 0.362 | .004 | 0.491 | <.001 | −0.480 | <.001 |
Log BNP | −0.556 | <.001 | −0.600 | <.001 | −0.537 | <.001 |
Peak oxygen consumption | 0.485 | <.001 | 0.594 | <.001 | 0.560 | <.001 |
Peak DSE | ||||||
LV end-diastolic volume | −0.679 | <.001 | −0.568 | <.001 | −0.453 | <.001 |
LV end-systolic volume | −0.719 | <.001 | −0.689 | <.001 | −0.589 | <.001 |
LV ejection fraction | 0.596 | <.001 | 0.823 | <.001 | 0.807 | <.001 |
WMSI | −0.484 | <.001 | −0.584 | <.001 | −0.524 | <.001 |
Change in LV ejection fraction | 0.132 | NS | 0.474 | <.001 | 0.416 | <.001 |
Sixty-seven patients (80%) underwent cardiopulmonary exercise testing limited by symptoms (either shortness of breath or fatigue). Systolic blood pressure increased significantly during exercise (from 118 ± 22 to 143 ± 26 mm Hg, P < .001), as well as heart rate (from 78 ± 14 to 118 ± 16 beats/min, P < .001) and the double product (from 7839 ± 1743 to 13,145 ± 3923, P < .001). Mean peak oxygen consumption was 14 ± 4 mL/kg/min. Patients with lower PVRs during DSE had lower exercise tolerance, assessed by peak oxygen consumption ( r = 0.516, P < .001; Table 3 ).
We divided the patients into 3 equal groups on the basis of PVR: lower (mean, 0.18 ± 0.27 mm Hg/mL/m 2 ; range < 0.48 mm Hg/mL/m 2 ), intermediate (mean, 1.02 ± 0.27 mm Hg/mL/m 2 ; range, 0.49-1.41 mm Hg/mL/m 2 ), and higher (mean, 4.02 ± 2.88 mm Hg/mL/m 2 ; range > 1.42 mm Hg/mL/m 2 ) ( Table 4 ). Patients with CHF with higher PVRs showed lower log BNP levels ( Figure 2 ) and better exercise tolerance, defined according to peak oxygen consumption, than those with intermediate and lower PVRs ( Figure 3 ).
Variable | PVR Lower (n = 28) | Intermediate (n = 28) | Higher (n = 28) | P |
---|---|---|---|---|
Age (y) | 66 ± 9 | 79 ± 9 | 68 ± 9 | NS |
Men | 21 (75%) | 23 (82%) | 20 (71%) | NS |
NYHA class > II | 21 (75%) | 17 (61%) | 14 (50%) | .045 |
Left atrial diameter (mm) | 49 ± 6 | 47 ± 5 | 48 ± 5 | NS |
LV end-diastolic diameter (mm) | 68 ± 9 | 65 ± 4 | 62 ± 5 ∗ | .005 |
LV end-systolic diameter (mm) | 58 ± 10 | 56 ± 4 | 51 ± 5 ∗† | .001 |
LV end-diastolic volume (mL) | 186 ± 73 | 155 ± 37 | 134 ± 34 ∗ | .001 |
LV end-systolic volume (mL) | 140 ± 63 | 113 ± 28 | 91 ± 30 ∗ | <.001 |
LV ejection fraction (%) | 26 ± 7 | 27 ± 6 | 33 ± 8 ∗† | <.001 |
WMSI at rest | 2.42 ± 0.31 | 2.34 ± 0.26 | 2.21 ± 0.23 | NS |
Mitral regurgitation ≥ moderate | 15 (54%) | 11 (39%) | 4 (14%) | .003 |
E/Ea ratio | 18 ± 11 | 17 ± 8 | 12 ± 4 ∗ | .023 |
Peak mitral annular systolic velocity (cm/s) | 4.1 ± 1.2 | 4.1 ± 0.9 | 5.1 ± 1.3 ∗† | .019 |
Log BNP (pg/mL) | 2.6 ± 0.5 | 2.5 ± 0.3 | 2.0 ± 0.5 ∗† | <.001 |
Peak oxygen consumption (mL/kg/min) | 12 ± 3 | 13 ± 3 | 17 ± 4 ∗† | <.001 |
Peak DSE | ||||
LV end-diastolic volume (mL) | 182 ± 74 | 140 ± 34 ∗ | 106 ± 26 ∗† | .001 |
LV end-systolic volume (mL) | 128 ± 74 | 82 ± 22 ∗ | 45 ± 22 ∗† | <.001 |
LV ejection fraction (%) | 31 ± 9 | 42 ± 7 ∗ | 59 ± 13 ∗† | <.001 |
WMSI at peak DSE | 2.2 ± 0.4 | 2.0 ± 0.3 ∗ | 1.6 ± 0.5 ∗† | <.001 |
Change in LV ejection fraction (%) | 22 ± 32 | 56 ± 29 ∗ | 84 ± 50 ∗† | <.001 |
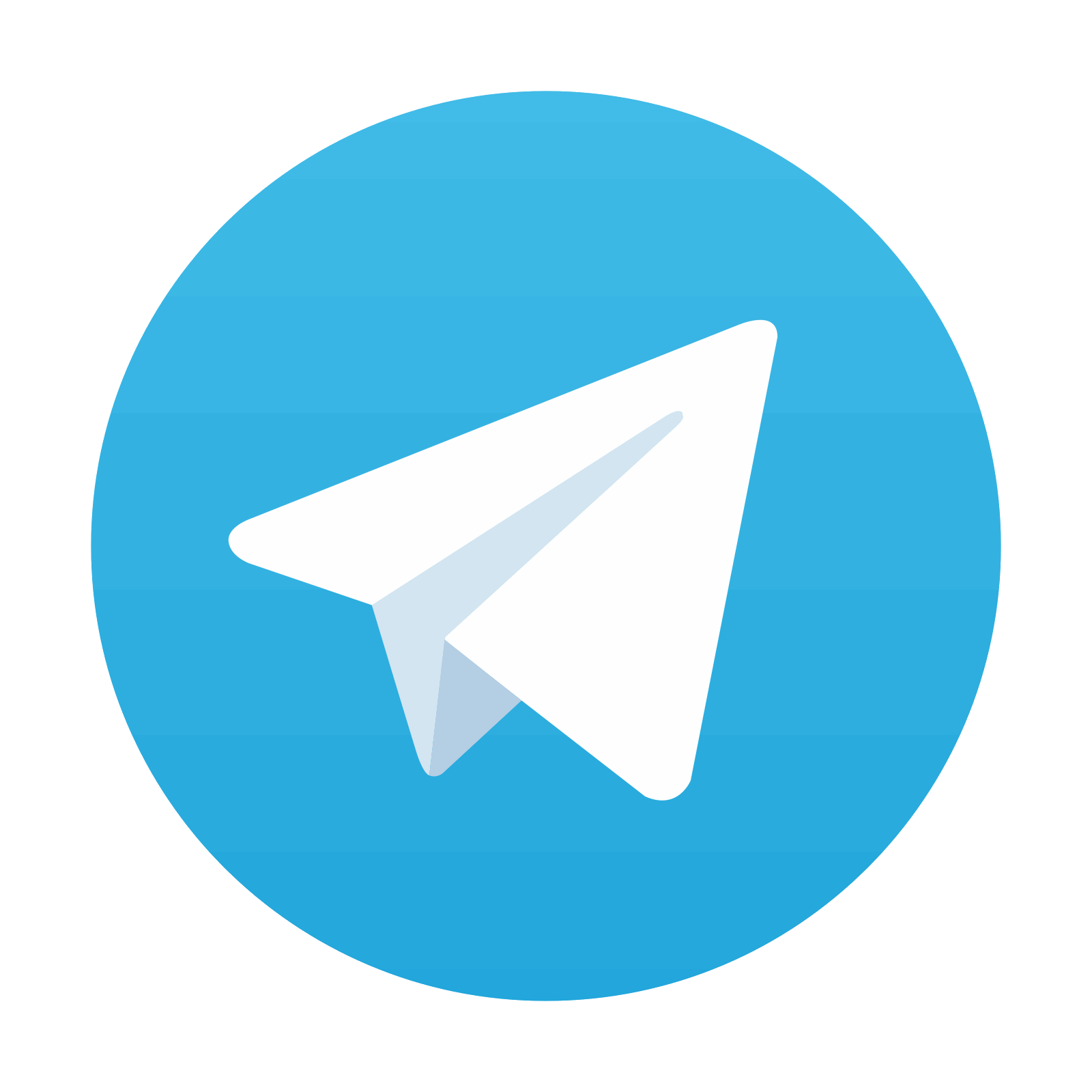
Stay updated, free articles. Join our Telegram channel

Full access? Get Clinical Tree
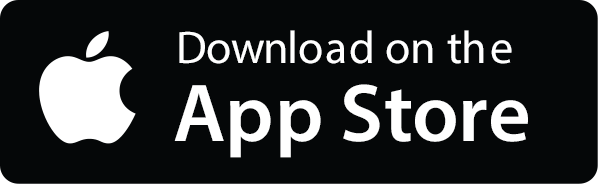
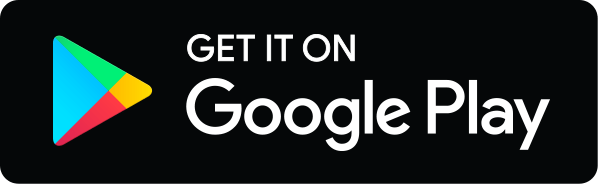
