Pressure Measurements
Mauro Moscucci, MD, MBA
Calin V. Maniu, MD
INTRODUCTION
The beginning of invasive hemodynamics can be traced back to the early 1700s, when Rev. Stephen Hales measured the blood pressure of a horse by inserting a brass rod in the femoral artery (FA), and observing a column of blood rising into a 9 foot glass tube connected to the rod.1,2 He was surprised to see that the horse barely moved and appeared to be well afterward. Stephen Hales later proceeded to define what we know today as cardiac output, by measuring the amount of blood going through the heart in 1 minute. About a century later, in 1844, the first invasive cardiac catheterization was performed by Claude Bernard. Again, the subject was a horse, and both the right and left ventricles were entered by retrograde approach from the jugular vein and from the carotid artery. In 1861, Chauveau and Marey published their work regarding the cardiac cycle and their discovery that ventricular systole and apical beat are simultaneous. In addition, they were able to measure simultaneously left ventricular (LV) and central aortic (Ao) pressure. Over the following century, the field of cardiac catheterization continued to expand.2 In 1929, Werner Forssmann performed the first right-sided heart catheterization of a human heart on himself3 (FIGURE 7.1), and in 1931, he proceeded to demonstrate that it was possible to inject contrast material in the human heart.4 In the same year, O’Klein in Prague measured cardiac output in man according to Fick principle.5 Further progress ensued with the pioneering work of Cournand and Richards, who performed cardiac catheterization in many clinical conditions including hypertension, circulatory shock, and chronic lung disease.6,7,8,9,10,11,12,13,14,15,16,17 As Cournand elegantly said in his Nobel Prize acceptance speech, the cardiac catheter was “only the key that opened the lock”.18 However, the lock that was open has been critical in understanding the pathophysiology of many cardiovascular conditions.2
While the past 2 decades have been characterized by an expansion of noninvasive assessment of cardiac function, with the development of the new field of catheter-based interventions for structural heart disease, invasive hemodynamic assessment remains a mainstay of cardiac catheterization and interventional cardiology. The objective of this chapter is to provide a brief review of classic hemodynamic measurements and tracings.
PRESSURE WAVEFORMS
As shown in FIGURES 7.2, 7.3, 7.4 and 7.5 the pressure waveform is the summation of forward pressure, forward flow waves, and reflected waves.19,20,21,22 Various conditions can affect the magnitude of reflected waves. For example, pressure reflections decrease during the strain phase of the Valsalva maneuver and increase during the release phase.23 They decrease in the setting of hypovolemia and in response to vasodilators, while they are higher in patients with hypertension, heart failure,20 and aortic or iliofemoral obstruction.24 The interaction between forward flow waves, forward pressure, reflected waves, and the time needed for pressure and flow waves to travel in the arterial tree will result in different timing and shape of the arterial waveform at different sites of the arterial tree19,22,25 (FIGURES 7.4, 7.5 and 7.6).
Ventricular Pressure
The ventricular pressure waveform can be divided into 4 major phases: isovolumic contraction, ejection, isovolumic relaxation, and diastolic filling. With the beginning of ventricular systole and closure of the atrioventricular mitral valve, intraventricular pressure rises without a change in volume (isovolumic contraction) until opening of the aortic valve. On opening of the aortic valve, ventricular ejection begins and continues until closure of the semilunar valve. This phase is followed by isovolumic relaxation, during which LV pressure decreases progressively without a change in volume. At the end of this phase, the atrioventricular valve opens and rapid filling begins. As relaxation continues during rapid filling, the ventricular diastolic pressure continues to fall. After the minimum pressure is reached, ventricular filling results in a progressive increase of LV diastolic pressures, followed by a positive wave corresponding to the atrial contribution to the ventricular filling26 (FIGURES 7.7, 7.8 and 7.9). Diastasis corresponds to a specific phase of diastole between rapid filling and atrial contraction, during which the progressive rise in LV diastolic pressure is not accompanied by a significant increase in volume.
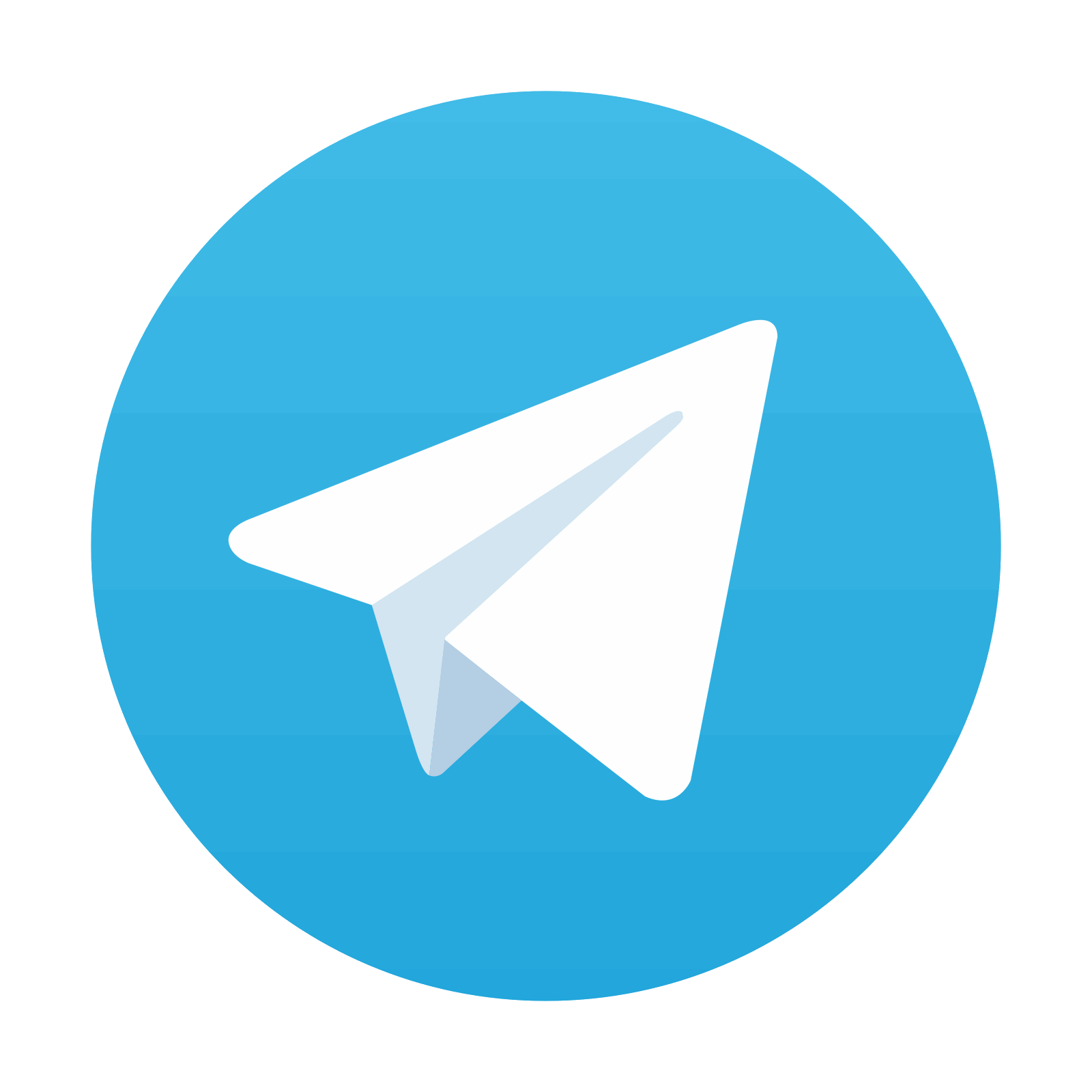
Stay updated, free articles. Join our Telegram channel

Full access? Get Clinical Tree
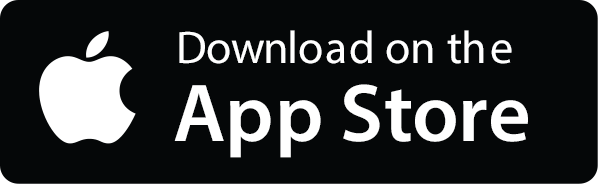
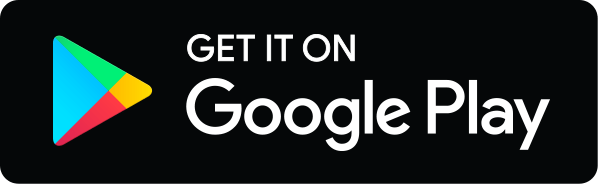