Preexcitation Syndromes
Preexcitation exists when, in relation to atrial events, all or some part of the ventricular muscle is activated by the atrial impulse sooner than would be expected if the impulse reached the ventricles only by way of the normal atrioventricular (A-V) conduction system.1 The clinical significance of the preexcitation syndromes relates primarily to the high frequency of associated arrhythmias and to the various bizarre and often misleading associated electrocardiographic patterns. Understanding the pathophysiologic basis for arrhythmias in these disorders provides much of our knowledge concerning the mechanism of reentrant arrhythmias.
The preexcitation syndromes previously were classified on the basis of proposed anatomic connections described by the eponyms Kent fibers, James fibers, and Mahaim fibers. The major objection to this classification was that it was imprecise and did not allow sufficient flexibility in explaining accumulated electrophysiologic and pathologic observations. As a consequence, many of these eponyms were inappropriately applied to various forms of preexcitation. Consequently, the European Study Group for Preexcitation devised a new classification of the preexcitation syndromes, based on their proposed anatomic connections.2 These connections are (a) A-V bypass tracts forming direct connections between the atria and ventricles,3,4,5,6,7 (b) nodoventricular fibers connecting the A-V node to the ventricular myocardium,4,8,9,10 (c) fasciculoventricular connections from the His–Purkinje system to the ventricular myocardium,11 and (d) A-V nodal bypass tracts, direct communications from the atrium to the His bundle,3,12 or from the atrium to the lower A-V node via a specialized internodal tract,9 or via specialized intranodal tracts with rapid conduction.13,14 Subsequently, connections defined pathophysiologically as atriofascicular and nodofascicular (i.e., from the atrium or A-V node to the right bundle branch or adjacent myocardium) have been described and distinguished from nodoventricular fibers.15,16,17,18,19,20 Pathologic examination of a surgically excised specimen revealed what appeared to be an accessory A-V nodal–His-like conducting system structure.21,22 A schema of these pathways is shown in Figure 10-1.
Physiologic (to be distinguished from anatomic) A-V connections produce the classic Wolff–Parkinson–White (WPW) syndrome; nodoventricular, atriofascicular, nodofascicular, and fascicular ventricular connections (which were frequently referred to as “Mahaim fibers”) produce WPW variants, and A-V nodal bypass tracts may produce the so-called Lown–Ganong–Levine syndrome. Of note is that many of the fibers actually described by Mahaim have been demonstrated to exist anatomically in the absence of electrophysiologic function.2,4,10 Hence, anatomic descriptors will be retained but applied only when the accessory pathways demonstrate electrophysiologic function. Because these pathways appear to represent developmental abnormalities, it is not surprising that multiple types of accessory pathways may exist in any individual patient.
Atrioventricular Bypass Tracts
The A-V bypass tract is the most frequently encountered type of preexcitation, and it is the only type for which a reproducible correlation has been demonstrated between electrophysiologic function and anatomic structure. Functioning A-V bypass tracts produce the typical WPW ECG pattern of a short P-R interval (≤0.12 seconds), a slurred upstroke of the QRS complex (delta wave), and a wide QRS complex (≥0.12 seconds). The first accurate description of the mechanism of the WPW Syndrome was by Wolferth and Wood in 1933.23 They beautifully described the mechanism of the QRS complex and the SVT in the disorder. The typical QRS pattern results because the atrial impulse bypasses the normal delaying site (A-V node) and initiates ventricular depolarization earlier than expected. The length of the P-R interval and the degree of preexcitation (which may be variable) depend on several factors: (a) A-V nodal and His–Purkinje conduction time; (b) conduction time of the sinus impulse to the atrial insertion of the bypass tract, which in turn depends on the distance between the bypass tract and the sinus node as well as on intra-atrial conduction and refractoriness; and (c) conduction time through the bypass tract, which is a function of its structure (length and thickness), the quality of input to the bypass tract, and the spatial–geometric arrangement between the atrium and ventricles, which determines the quality of the electrical input and output of the bypass tract.21,24 Hence, the P-R interval occasionally exceeds 0.12 sec if intra-atrial conduction delay or prolonged conduction over the bypass tract is present. Moreover, if these delays are marked, preexcitation of the ventricles can occur after the onset of the QRS complex
and fail to produce the classic delta wave or wide QRS complex, yet still activate that part of the ventricles in which the bypass tract inserts earlier than it would have been activated over the normal pathway. Delayed input into the bypass tract resulting in this apparent paradox is most likely to occur in left lateral A-V bypass tracts. Enhanced A-V nodal conduction and/or slow conduction over the bypass tract are additional mechanisms for inapparent preexcitation. An example of this phenomenon is shown in Figure 10-2. During sinus rhythm (Fig. 10-1A), the P-R interval and QRS complex are normal; no evidence of preexcitation is apparent. However, during two beats of a junctional rhythm at a cycle length of 600 msec, with the same H-V interval, the QRS complex differs significantly, with diminution of the Q wave in lead 1, the appearance of a Q wave and loss of the slurred upstroke in lead aVF, and a decrease in the amplitude of the R wave in V1. Earliest retrograde activation is seen in the coronary sinus, which is concordant with a left-sided anterograde conduction over a left-sided bypass tract (exaggerated q in lead I and r in V1). The shorter V-A than A-V suggests that antegrade conduction over the bypass tract is rather slow. These findings suggest that during sinus rhythm part of the ventricle is activated earlier than expected but occurs simultaneously with or just after the initial activation of the ventricles over the normal pathway. The presence of preexcitation is recognized only when the QRS in sinus rhythm is compared to the QRS during SVT, which is produced by ventricular activation solely over the normal A-V conducting system. Therefore, any combination of delayed input to the bypass tract, slow conduction over the bypass tract, or fast conduction over the A-V conducting system may result in inapparent preexcitation.
and fail to produce the classic delta wave or wide QRS complex, yet still activate that part of the ventricles in which the bypass tract inserts earlier than it would have been activated over the normal pathway. Delayed input into the bypass tract resulting in this apparent paradox is most likely to occur in left lateral A-V bypass tracts. Enhanced A-V nodal conduction and/or slow conduction over the bypass tract are additional mechanisms for inapparent preexcitation. An example of this phenomenon is shown in Figure 10-2. During sinus rhythm (Fig. 10-1A), the P-R interval and QRS complex are normal; no evidence of preexcitation is apparent. However, during two beats of a junctional rhythm at a cycle length of 600 msec, with the same H-V interval, the QRS complex differs significantly, with diminution of the Q wave in lead 1, the appearance of a Q wave and loss of the slurred upstroke in lead aVF, and a decrease in the amplitude of the R wave in V1. Earliest retrograde activation is seen in the coronary sinus, which is concordant with a left-sided anterograde conduction over a left-sided bypass tract (exaggerated q in lead I and r in V1). The shorter V-A than A-V suggests that antegrade conduction over the bypass tract is rather slow. These findings suggest that during sinus rhythm part of the ventricle is activated earlier than expected but occurs simultaneously with or just after the initial activation of the ventricles over the normal pathway. The presence of preexcitation is recognized only when the QRS in sinus rhythm is compared to the QRS during SVT, which is produced by ventricular activation solely over the normal A-V conducting system. Therefore, any combination of delayed input to the bypass tract, slow conduction over the bypass tract, or fast conduction over the A-V conducting system may result in inapparent preexcitation.
The incidence of A-V bypass tracts detected electrocardiographically has been variously reported as 0.1 to 3.1/1,000, and it has been noted in people of all ages, although its incidence decreases with increasing age.25,26,27 However, there appears to be an increased familial incidence of the disorder. Recent study by Vidaillet et al.28 demonstrated a 3.4% incidence of accessory pathways in first-degree relatives of patients with preexcitation, an incidence significantly higher than that of the general population (p < 0.0001). The incidence may even be higher if one could obtain evidence of concealed accessory pathways (see Chapter 8). In fact, we have seen several families in whom various members have evidence of overt preexcitation while other relatives have concealed bypass tracts. This is not surprising, considering that bypass tracts are thought to result from developmental abnormalities of the A-V ring. It is important to recognize that evidence of preexcitation may disappear. In a longitudinal study of patients with symptomatic WPW, Chen et al.29 noted a loss of antegrade preexcitation of 22.5% over a 10-year follow-up. Whether or not the likelihood of loss of preexcitation is higher in asymptomatic patients is unknown.
Functioning A-V bypass tracts are associated with certain congenital abnormalities, particularly Ebstein’s anomaly of the tricuspid valve. Patients with Ebstein’s anomaly have a 10% incidence of preexcitation, which invariably have at least one anatomic right-sided (to the anatomic right ventricle) insertion.5,30,31 This remains the case with corrected transposition, in which Ebstein’s anomaly of the left (tricuspid) valve is asssociated with bypass tracts to the functioning systemic ventricle (anatomic right ventricle). Furthermore, multiple bypass tracts are frequently observed in Ebstein’s anomaly. Conversely, of patients with WPW syndrome presenting with SVT early in childhood, only 5% have Ebstein’s anomaly, despite the fact that it is the most common form of congenital heart disease associated with WPW. Interestingly, Deal et al.30 found that children with left-sided bypass tracts infrequently have structural heart disease (5%), while in contrast, 45% of patients with right-sided bypass tracts have associated heart disease. In their study, structural heart disease was present in only 20% of the patients presenting with the WPW syndrome in the first 4 months of life.
In adults, an association with the left-sided bypass tracts and mitral valve prolapse has been noted.32,33 Left-sided bypass tracts appear to be the most common, however, and because mitral valve prolapse is also common in the patient population, their association may in fact represent the coexistence of two relatively common conditions. Although several other disorders have been said to be associated with the WPW syndrome, the true incidences are unknown because of the lack of electrophysiologic studies in all these patient populations. For example, of 30 patients with hypertrophic obstructive cardiomyopathy (HOCM) specifically referred for electrophysiology study because of surface ECGs suggestive of WPW, none had electrophysiologic evidence of A-V bypass tracts. In fact, I have seen only two patients with HOCM who had the WPW syndrome, and both patients presented with SVT. Recently other forms of cardiomyopathy have been described with “WPW” syndrome, but these are not associated with previously mapped and reported defects associated with classic HOCM. The most well studied are mutations in PRKAG2, an enzyme modulating glucose uptake and glycolysis. Blair et al.,34 Golub et al.,35 and Arad et al.36 have described a glycogen storage hypertrophic cardiomyopathy associated with WPW in patients with PRKAG2 mutations. The ECGs presented in some of these patients do not appear to be
classic AV bypass tracts. More recently Yang et al.37 described a skeletal myopathy and LVH associated with WPW due to mutations in the gene encoding the lysosome-associated protein-2 (LAMP-2). Nevertheless, in the vast majority of young patients with A-V bypass tracts, no heart disease is present.
classic AV bypass tracts. More recently Yang et al.37 described a skeletal myopathy and LVH associated with WPW due to mutations in the gene encoding the lysosome-associated protein-2 (LAMP-2). Nevertheless, in the vast majority of young patients with A-V bypass tracts, no heart disease is present.
As noted, the clinical significance of WPW syndromes is the high frequency of arrhythmias and the sometimes-confusing electrocardiographic patterns that can mimic bundle branch block and infarction. Forty to eighty percent of patients with A-V bypass tracts manifest tachyarrhythmias,
the most common of which is a circus movement tachycardia (orthodromic SVT) using the normal A-V conducting system as the antegrade limb and the bypass tract as the retrograde limb of the reentrant circuit.32,38 A reversed pattern of circus movement tachycardia (antidromic SVT) occurs when antegrade conduction proceeds over the bypass tract and retrograde conduction occurs over the normal A-V pathway or, in many instances, an additional bypass tract.38,39,40,41,42 Clinically, antidromic tachycardias are much less frequent than orthodromic tachycardias (see following discussion). Atrial flutter and fibrillation are less common presenting arrhythmias but are potentially more life threatening, because they can result in extremely rapid ventricular rates that precipitate ventricular tachycardia and/or fibrillation.32,43,44 Atrial flutter-fibrillation may be the presenting arrhythmia in 5% to 10% of patients with A-V bypass tracts and occurs even more commonly when orthodromic or antidromic tachycardia also is present. As many as 50% of patients with symptomatic arrhythmias have been reported to develop atrial fibrillation of variable duration at some time.45,46 It is much lower in my experience. The incidence of atrial flutter and/or fibrillation does appear to be higher in patients with A-V bypass tracts than in the normal population, the explanation of which is not understood. We have not found hemodynamic differences during tachycardias, atrial activation sequences that are related to bypass tract location, or tachycardia rate to be critically important in developing atrial fibrillation. Another interesting observation is that atrial fibrillation appears to be five times more common when overt preexcitation (i.e., WPW) is present than in patients with concealed bypass tracts at similar locations and similar rates of tachycardias. Patients with atrial fibrillation have a higher incidence of inducible atrial fibrillation than those without the arrhythmia. Others have made similar observations.45,46 The mechanisms of atrial fibrillation and tachycardias are discussed later in the chapter. In patients who are asymptomatic the incidence of sudden cardiac death (assumed secondary to atrial fibrillation) is virtually nil.29,47 While patients over 40 years who have never had symptomatic arrhythmias related to their accessory pathway remain asymptomatic, for those younger than 40 there is a 30% chance of developing symptoms, that is, SVT.47
the most common of which is a circus movement tachycardia (orthodromic SVT) using the normal A-V conducting system as the antegrade limb and the bypass tract as the retrograde limb of the reentrant circuit.32,38 A reversed pattern of circus movement tachycardia (antidromic SVT) occurs when antegrade conduction proceeds over the bypass tract and retrograde conduction occurs over the normal A-V pathway or, in many instances, an additional bypass tract.38,39,40,41,42 Clinically, antidromic tachycardias are much less frequent than orthodromic tachycardias (see following discussion). Atrial flutter and fibrillation are less common presenting arrhythmias but are potentially more life threatening, because they can result in extremely rapid ventricular rates that precipitate ventricular tachycardia and/or fibrillation.32,43,44 Atrial flutter-fibrillation may be the presenting arrhythmia in 5% to 10% of patients with A-V bypass tracts and occurs even more commonly when orthodromic or antidromic tachycardia also is present. As many as 50% of patients with symptomatic arrhythmias have been reported to develop atrial fibrillation of variable duration at some time.45,46 It is much lower in my experience. The incidence of atrial flutter and/or fibrillation does appear to be higher in patients with A-V bypass tracts than in the normal population, the explanation of which is not understood. We have not found hemodynamic differences during tachycardias, atrial activation sequences that are related to bypass tract location, or tachycardia rate to be critically important in developing atrial fibrillation. Another interesting observation is that atrial fibrillation appears to be five times more common when overt preexcitation (i.e., WPW) is present than in patients with concealed bypass tracts at similar locations and similar rates of tachycardias. Patients with atrial fibrillation have a higher incidence of inducible atrial fibrillation than those without the arrhythmia. Others have made similar observations.45,46 The mechanisms of atrial fibrillation and tachycardias are discussed later in the chapter. In patients who are asymptomatic the incidence of sudden cardiac death (assumed secondary to atrial fibrillation) is virtually nil.29,47 While patients over 40 years who have never had symptomatic arrhythmias related to their accessory pathway remain asymptomatic, for those younger than 40 there is a 30% chance of developing symptoms, that is, SVT.47
Electrophysiologic Properties of A-V Bypass Tracts
Unlike the A-V node, conduction over an A-V bypass tract usually behaves in an all-or-none fashion. Rapid pacing or atrial premature depolarizations (APDs) either conduct without delay (no change in P-to-delta wave) or block suddenly (Fig. 10-3). Progressive slowing of conduction over an A-V bypass tract in response to APDs or atrial pacing has been documented in patients with assumed rapidly conducting bypass tracts (P-to-delta ≤0.12 seconds) or in whom a diagnosis of atriofascicular, nodofascicular, or nodoventricular bypass tracts was suspected.18,21,22,24,48,49 Slowly conducting atrioventricular bypass tracts are rare (<1%) in patients with preexcitation over an apparent atrioventricular bypass tract. These are most commonly found at the right anterior free wall. Decremental conduction (i.e., prolonged P–delta wave) in response to APDs or atrial pacing with persistence of preexcitation can also be due to intra-atrial conduction delay between the site of stimulation and the bypass tract. Wenckebach-type second-degree block in a bypass tract in response to pacing is rarely observed. When it does occur, it is most commonly in the presence of an atriofascicular bypass tract (see subsequent discussion of bypass tracts with decremental conduction).
Not uncommonly, intermittent conduction over a bypass tract is observed. This is to be distinguished from inapparent preexcitation that is due to prolonged intra-atrial conduction, accelerated A-V conduction, prolonged conduction over a bypass tract, or any combination of these. Four specific conditions can produce intermittent preexcitation (i.e., presence and absence of preexcitation on the same tracing during sinus rhythm or pacing at 60 to 100 beats per minute [bpm]): (a) acceleration-dependent and deceleration-dependent (phase 4) block in the accessory pathway (Fig. 10-4);50,51,52 (b) A-V bypass tracts with long refractory periods and supernormal conduction;53,54 (c) antegrade or retrograde concealed conduction produced by APDs, ventricular premature depolarizations (VPDs), or atrial tachyarrhythmias55,56,57 and a long bypass tract refractory period with a gap phenomenon in response to APDs.
To recognize the phase 4 block and phase 3 block, one must demonstrate absence of preexcitation at long cycle lengths followed by evidence of preexcitation at shorter paced cycle lengths and sudden block again at even shorter paced cycle lengths (Fig. 10-4). One must exclude rapid A-V conduction over the normal A-V conduction system during slow rhythms that would reach the ventricles before the bypass tract, thereby preventing manifest preexcitation. Subsequently, pacing at
increasing rates produces prolonged A-V conduction time over the normal pathway, which allows preexcitation to become manifest. A second piece of evidence that supports the presence of phase 4 block is observation of premature complexes with a similar configuration of the preexcited complex (Fig. 10-5).50 These premature complexes are a direct manifestation of automaticity in the bypass tract.
increasing rates produces prolonged A-V conduction time over the normal pathway, which allows preexcitation to become manifest. A second piece of evidence that supports the presence of phase 4 block is observation of premature complexes with a similar configuration of the preexcited complex (Fig. 10-5).50 These premature complexes are a direct manifestation of automaticity in the bypass tract.
For diagnosis of supernormal conduction, one should demonstrate preexcitation at long cycle lengths followed by block at shorter (but still relatively long) cycle lengths, and long bypass tract refractory periods. At even shorter coupling intervals or shorter paced cycle lengths, preexcitation once again appears. No change should occur in the P-to-delta intervals during both periods of preexcitation (i.e., at long and short cycle lengths). This criterion is needed to exclude “pseudo”-supernormal phenomena that are due to a gap phenomenon, as discussed in Chapter 6. If gap phenomenon was responsible for “pseudo-supernormal” conduction, the conducted beat at a shorter cycle length should have a longer P to delta due to proximal to ventricle delay in the bypass tract. Although most cases of intermittent preexcitation have not been well studied, the clinical implications are that they generally have long refractory periods and are incapable of rapid repetitive conduction down the bypass tract, hence slower ventricular response, during atrial flutter-fibrillation.38,58 Of note, some patients with intermittent preexcitation can have improved antegrade conduction over the bypass tract, causing persistent preexcitation, following administration of isoproterenol, which shortens the refractory period of the bypass tract.38,59 Shortening of the refractory period of the bypass tract may allow conduction to resume at cycle lengths 200 msec shorter, but these cycle lengths are still in the range of 300 to 400 msec, and thus, not potentially lethal. Demonstration of concealed conduction causing absence of preexcitation requires demonstration of APD that is nonconducted over the bypass tract or a VPD influencing antegrade conduction over the bypass tract on subsequent beats. This is quite common as a mechanism for runs of overt preexcitation followed by runs of absent preexcitation during atrial fibrillation.
The vast majority of A-V bypass tracts conduct both anterogradely and retrogradely. Less than 5% of patients with preexcitation have bypass tracts that conduct only antegradely.60 This is much less common than the converse situation of retrogradely conducting bypass tracts in the absence of antegrade preexcitation (i.e., so-called concealed bypass tracts). In patients who manifest only antegrade conduction over their bypass tract, spontaneous circus movement
tachycardia, either antidromic or orthodromic, is not usually observed, but when it is, it is antidromic. The primary rhythm disturbance they manifest is atrial fibrillation, in which case, the bypass tract is not related to the mechanism of the fibrillation but serves as a conduit of rapid conduction to the ventricles.60 A caveat to this estimate of bypass tracts that conduct only antegradely is that patients studied in electrophysiology laboratories are a selected group of patients. It is estimated by some that if a populational study of all asymptomatic patients with a WPW ECG were studied, the percent of patients with antegrade only conduction might be as high as 40% to 50%.61
tachycardia, either antidromic or orthodromic, is not usually observed, but when it is, it is antidromic. The primary rhythm disturbance they manifest is atrial fibrillation, in which case, the bypass tract is not related to the mechanism of the fibrillation but serves as a conduit of rapid conduction to the ventricles.60 A caveat to this estimate of bypass tracts that conduct only antegradely is that patients studied in electrophysiology laboratories are a selected group of patients. It is estimated by some that if a populational study of all asymptomatic patients with a WPW ECG were studied, the percent of patients with antegrade only conduction might be as high as 40% to 50%.61
![]() FIGURE 10-5 Enhanced automaticity of bypass tract. Surface leads 1, aVF, and V1 are shown with proximal (HBEp), mid- (HBEm) and distal (HBEd) HBEs. An accelerated regular wide-complex rhythm is seen during atrial fibrillation. The morphology of this wide complex is similar to that of preexcited complexes shown in Figure 10-3. This most likely represents enhanced automaticity in the bypass tract. See text for discussion. |
As noted earlier, over time antegrade conduction over an atrioventricular bypass tract may disappear. Chen et al.29 noted a loss of preexcitation in one-fifth of symptomatic patients with WPW. Only 7.8% lost retrograde conduction. Spontaneous loss of preexcitation has been observed in one-fifth to one-half of children with WPW.30,62 In my experience, spontaneous loss of preexcitation occurs in less than 10% of adults (i.e., >18 years old), and loss of retrograde conduction is noted in <2% of patients. Data from our laboratory is limited by selection bias, which is a result of our university status and our early use of surgery and catheter ablation. The long-term natural history of patients with the WPW syndrome is currently virtually impossible to define in the Western world because of early intervention.
Electrophysiologic Evaluation in Patients with Wolff–Parkinson–White Syndrome
Electrophysiologic studies in patients with WPW are useful for (a) confirming the diagnosis; (b) studying the mode of initiation of tachycardias; (c) localizing the bypass tract; (d) demonstrating that the bypass tract participates in the tachycardias; (e) evaluating the refractoriness of the bypass tract and its implication for risk of life-threatening arrhythmias; (f) terminating tachycardias; and (g) aiding the development of pharmacologic, pacing, or ablative therapy for arrhythmias associated with WPW syndrome.
Diagnosis of an A-V Bypass Tract
By definition, if an A-V bypass tract is present, activation of some part of the ventricles begins earlier than expected, so that the H-V interval (in this case, the His-to-delta-wave interval) is less than normal at rest and/or can be made less than normal by various maneuvers (Fig. 10-6). Because the QRS complex is a fusion complex of conduction (usually all-or-none) down a rapidly conducting bypass tract and conduction down the A-V node–His–Purkinje system, slowing of
conduction down the normal pathway results in an increasing degree of preexcitation. Such slowing can make preexcitation manifest when it was inapparent during sinus rhythm. This can be achieved pharmacologically, by carotid sinus massage, or by atrial stimulation. Typically, atrial pacing or APDs, which prolong A-V nodal conduction, cause a greater degree of preexcitation because while the P-to-delta-wave interval remains constant, the P-to-His increases, resulting in a smaller component of ventricular depolarization initiated over the normal A-V conducting system and a greater component over the bypass tract (Figs. 10-7 to 10-9). While atrial pacing at progressively decreasing cycle lengths from any atrial site increases the amount of preexcitation, the degree of preexcitation is maximal and the P-to-delta is shortest at comparable paced cycle lengths if atrial pacing is performed at or near the atrial insertion site of the bypass tract. This is discussed later in this chapter as a means to locate a bypass tract.
conduction down the normal pathway results in an increasing degree of preexcitation. Such slowing can make preexcitation manifest when it was inapparent during sinus rhythm. This can be achieved pharmacologically, by carotid sinus massage, or by atrial stimulation. Typically, atrial pacing or APDs, which prolong A-V nodal conduction, cause a greater degree of preexcitation because while the P-to-delta-wave interval remains constant, the P-to-His increases, resulting in a smaller component of ventricular depolarization initiated over the normal A-V conducting system and a greater component over the bypass tract (Figs. 10-7 to 10-9). While atrial pacing at progressively decreasing cycle lengths from any atrial site increases the amount of preexcitation, the degree of preexcitation is maximal and the P-to-delta is shortest at comparable paced cycle lengths if atrial pacing is performed at or near the atrial insertion site of the bypass tract. This is discussed later in this chapter as a means to locate a bypass tract.
The ability to change the degree of preexcitation by increasing A-V nodal conduction time in response to atrial stimulation supports the concept that the QRS complex is a fusion complex. The ultimate expression of dual ventricular activation is demonstrated in Figure 10-10, in which two ventricular responses are shown to result from a single APD: the first is over the bypass tract, and the second is over the normal A-V conducting system after marked antegrade A-V nodal conduction delay.63 This phenomenon is almost always associated with dual A-V nodal pathways (see Chapter 8). This phenomenon is a potential mechanism for the development of either orthodromic or, rarely, A-V nodal reentrant tachycardia (AVNRT) which may be associated with anterograde conduction over an innocent bystander bypass tract (see discussion of preexcited tachycardias later in this chapter). Failure to increase the degree of preexcitation by atrial pacing or atrial extrastimuli may be due to (a) an additional A-V nodal bypass tract or markedly enhanced A-V nodal conduction, which prevents A-V nodal delay; (b) pacing-induced block in the A-V bypass tract that is due to a long refractory period exceeding that of the A-V node; or (c) total preexcitation that is present in the basal state as a result of prolonged or absent A-V conduction over the normal pathway.
Pharmacologic and/or physiologic maneuvers that can alter A-V nodal conduction may also be used to alter the degree of preexcitation, therefore confirming the presence of a bypass tract and the concept of a fusion complex. Isoproterenol and atropine can decrease the degree of preexcitation by shortening A-V nodal conduction. This allows more of the ventricles to be activated over the normal pathway owing to enhanced A-V conduction and consequently reducing the component of ventricular activation over the bypass tract. Carotid sinus pressure, digoxin, beta blockers, calcium blockers, or adenosine can increase the degree of preexcitation by prolonging A-V nodal conduction so that most of the ventricles are activated over the bypass tract. Carotid sinus pressure may also result in transient A-V nodal block with a His bundle escape rhythm. This escape rhythm should manifest a normal (narrow) QRS complex if preexcitation were caused by an A-V connection (Fig. 10-11), but would not alter preexcitation if a bypass tract originated below the A-V node, as it would in a fasciculoventricular bypass tract (see discussion of Fasciculoventricular Bypass Tracts). Similar findings (i.e., normal QRS) would also be observed during His bundle pacing or during spontaneous His bundle extrasystoles (Fig. 10-12). Normalization of the QRS during a His bundle rhythm, particularly in the presence of drug or in response to a physiologic maneuver (e.g., carotid sinus pressure induced A-V block), confirm the origin of the bypass tract above the site of impulse formation of the escape rhythm, that is, in the high A-V node or in the atrium, with direct connection to the ventricle. While adenosine is widely used to produce transient A-V slowing or block in order to maximize preexcitation, it may induce atrial fibrillation, which may be associated with a rapid ventricular response.
Mode of Initiation Of Tachycardias
As noted, the most common tachycardias associated with the WPW syndrome are the circus movement tachycardias, 95% of which are orthodromic; that is, they conduct antegradely down the normal A-V conducting system and retrogradely up the bypass tract. The relationship of conduction and refractoriness of the normal A-V conducting system and the bypass tract, as well as the site of stimulation, determine both the ability to initiate circus movement tachycardia and, theoretically, the type of circus movement tachycardia.38,64,65 Conduction and refractoriness of the bypass tracts in most cases behave like working muscle; therefore, bypass tracts demonstrate rapid conduction, and have refractory periods that tend to shorten at decreasing paced cycle lengths. The WPW syndrome allows one to actually see all the requirements for
a reentrant rhythm: (a) two anatomic or functionally determined pathways of conduction; (b) unidirectional block in one of the pathways (in this instance, either in the accessory pathway or in the A-V nodal His pathway); (c) sufficient slowing in part of the circuit to overcome refractoriness ahead of the circulating impulse; and (d) conduction time of the impulse must exceed the longest effective refractory period of any component in the circuit. Both antegrade and retrograde refractory periods of the accessory pathway are major determinants of (a) the ability to initiate and sustain circus movement SVT, and (b) the ventricular responses to atrial tachyarrhythmias (e.g., atrial fibrillation, atrial flutter, and atrial tachycardia).
a reentrant rhythm: (a) two anatomic or functionally determined pathways of conduction; (b) unidirectional block in one of the pathways (in this instance, either in the accessory pathway or in the A-V nodal His pathway); (c) sufficient slowing in part of the circuit to overcome refractoriness ahead of the circulating impulse; and (d) conduction time of the impulse must exceed the longest effective refractory period of any component in the circuit. Both antegrade and retrograde refractory periods of the accessory pathway are major determinants of (a) the ability to initiate and sustain circus movement SVT, and (b) the ventricular responses to atrial tachyarrhythmias (e.g., atrial fibrillation, atrial flutter, and atrial tachycardia).
ORTHODROMIC TACHYCARDIA
The most common tachyarrhythmia in patients with WPW is orthodromic circus movement SVT in which antegrade conduction proceeds down the normal A-V conducting system and retrograde conduction occurs over the bypass tract, with intervening atrial and ventricular tissue completing the reentrant circuit. This is the identical circuit observed in reentrant SVT using a concealed bypass tract (described in detail in Chapter 8). As with patients with concealed bypass tracts, patients with WPW have orthodromic tachycardia initiated most commonly in response to spontaneous or stimulated APDs. The only difference between orthodromic tachycardias in patients with concealed bypass tracts and those with overt preexcitation is that antegrade block is already present in patients with concealed bypass tracts. In patients with WPW, the APD serves a dual purpose: (a) It produces block in the accessory pathway, and (b) it conducts slowly enough down the normal A-V conducting system and ventricles to allow that bypass tract and the atrium to recover excitability. Thus, initiation of orthodromic tachycardia by APDs requires that the antegrade refractory period of the bypass tract exceed that of the A-V conducting system and that the retrograde
refractory period of the bypass tract and the refractory period of the atrium recover by the time the ventricle is activated antegradely over the A-V conducting system. This sequence is schematically shown in Figure 10-13.
refractory period of the bypass tract and the refractory period of the atrium recover by the time the ventricle is activated antegradely over the A-V conducting system. This sequence is schematically shown in Figure 10-13.
The coupling interval of APDs required to initiate SVT in the WPW syndrome is somewhat shorter than that required for induction of circus movement tachycardia in patients with concealed bypass tract. This is a consequence of the fact that block in the bypass tract is required for initiation of the tachycardia. Because block is already present in patients with concealed bypass tracts, APDs delivered at longer coupling intervals may be sufficient to produce the requisite delay for reentry to occur. In our experience, the antegrade effective refractory period of the bypass tract (determined at a mean paced cycle length of 600 msec) in the majority of patients presenting with orthodromic tachycardia lies between 250 and 350 msec with a mean of just over 300 msec and a range of 180 to 600 msec. These data are comparable to those of Wellens and Brugada.38 In patients with bypass tracts demonstrating long antegrade effective refractory periods, particularly those with intermittent preexcitation, tachycardias can be more readily induced by late coupled APDs. Once block in the bypass tract is accomplished and antegrade conduction proceeds to the ventricle over the normal A-V conducting system, it is primarily the retrograde refractory period of the bypass tract and recovery of atrial refractoriness at the atrial insertion site of the bypass tract that determines whether or not a circus movement tachycardia will be initiated. For orthodromic tachycardia to occur, the retrograde refractory period of the bypass tract must be less than the conduction time over the A-V conducting system and through the ventricles. In addition, the refractory period of the atrium at the atrial site of insertion of the bypass tract must be shorter than that of the conduction time through the normal A-V conduction system, ventricle, and bypass tract to complete the circuit. Perpetuation of the tachycardia requires that the refractory period of any part of the tachycardia circuit be shorter than that of the tachycardia cycle length.
With this in mind, initiation of orthodromic SVT by APDs obviously requires some degree of A-V delay to allow recovery of the bypass tract, the atrium into which it is inserted, and the A-V node. As expected, the site of critical delay required
for initiation is most commonly in the A-V node; however, the delay may also occur anywhere in the His–Purkinje system, bundle branches, or ventricular myocardium. Most often, enough delay is already present at the coupling interval at which the bypass tract blocks for initiation of the tachycardia (Fig. 10-14). If A-V delay is not sufficient to allow the bypass tract to recover, further shortening of atrial coupling intervals is required to initiate the tachycardia (Fig. 10-15). However,
as noted, because the coupling intervals of APDs required to produce block in the bypass tract are usually short, enough A-V nodal delay is usually present so that the tachycardia is initiated at the onset of block. This is particularly true in patients who have dual A-V nodal pathways but no A-V nodal reentry. Early coupled APDs conduct over the “slow” pathway, providing more than enough A-V delay to initiate the tachycardia (Fig. 10-16). The critical requirement of His–Purkinje delay to initiate the tachycardia is shown in Figure 10-17. The importance of bundle branch block in initiation of tachycardias has been discussed in detail in Chapter 8 and is discussed again subsequently in this chapter. Basically, block in the bundle branch ipsilateral to a free wall bypass tract provides an additional amount of intramyocardial conduction delay, which allows the bypass tract or its atrial insertion time to recovery excitability. Thus, the site of delay is unimportant; all that matters is that all tissues in advance of the circulating impulse have recovered excitability.
for initiation is most commonly in the A-V node; however, the delay may also occur anywhere in the His–Purkinje system, bundle branches, or ventricular myocardium. Most often, enough delay is already present at the coupling interval at which the bypass tract blocks for initiation of the tachycardia (Fig. 10-14). If A-V delay is not sufficient to allow the bypass tract to recover, further shortening of atrial coupling intervals is required to initiate the tachycardia (Fig. 10-15). However,
as noted, because the coupling intervals of APDs required to produce block in the bypass tract are usually short, enough A-V nodal delay is usually present so that the tachycardia is initiated at the onset of block. This is particularly true in patients who have dual A-V nodal pathways but no A-V nodal reentry. Early coupled APDs conduct over the “slow” pathway, providing more than enough A-V delay to initiate the tachycardia (Fig. 10-16). The critical requirement of His–Purkinje delay to initiate the tachycardia is shown in Figure 10-17. The importance of bundle branch block in initiation of tachycardias has been discussed in detail in Chapter 8 and is discussed again subsequently in this chapter. Basically, block in the bundle branch ipsilateral to a free wall bypass tract provides an additional amount of intramyocardial conduction delay, which allows the bypass tract or its atrial insertion time to recovery excitability. Thus, the site of delay is unimportant; all that matters is that all tissues in advance of the circulating impulse have recovered excitability.
The site of stimulation may also be important, particularly if the limiting factor for initiation of the tachycardia is atrial refractoriness (i.e., atrial refractoriness prohibits the impulse from traversing the fully excitable bypass tract and activating the atrium). To obviate this limitation, one must stimulate near the atrial insertion of the bypass tract. This facilitates initiation for two reasons: (a) the closer the stimulation to the bypass tract, the easier it is to encroach on the refractory period of the bypass tract and achieve block; and (b) the earlier the atrium (or the bypass tract) is activated, the earlier it will recover, thereby facilitating reentry. Thus, one may actually require less antegrade delay if recovery of excitability is shifted earlier in time. This is shown in Figure 10-18, in which stimulation from the high-right atrium had failed to initiate a tachycardia, but stimulation from the coronary sinus initiated the tachycardia with minimal prolongation of A-V nodal conduction (A-H = 130 msec).
Occasionally, single atrial extrastimuli cannot induce block and/or enough delay to initiate tachycardia, and other modes of initiation are required. These include the induction of sinoatrial, intra-atrial, or A-V nodal echoes, which then either block in the bypass tract and/or produce enough conduction delay down the normal pathway to reenter the atria via the bypass tract. Similarly, rapid atrial pacing or the use of multiple extrastimuli can produce sudden block in the bypass tract and initiate SVT where single extrastimuli cannot. In some patients, drug-induced block in the bypass tract or drug-induced prolongation of the bypass tract refractory periods enables an increase in sinus rate to induce block in the bypass tract. This latter mechanism may lead to incessant SVT. Finally, atrial extrastimuli also can initiate tachycardias by a variety of 1:2 conduction phenomena (see Fig. 10-10); an atrial impulse conducts both over the bypass tract and over the normal A-V system. In Figure 10-19, stimulation at the distal coronary sinus (not shown) conducts over the bypass tract and through the A-V node, but conduction down the A-V conducting system initially cannot occur because either the His–Purkinje system or ventricles are encountered during
a refractory state. When the coupling interval is reduced to 250 msec, both A-H and H-V prolongation allow conduction to proceed to the ventricles, with the subsequent development of reentry over the left-sided bypass tract. Stimulation from the coronary sinus also allowed the left atrial insertion site of the bypass tract to recover excitability earlier than if right atrial stimulation had been employed. This was an additional factor in facilitating initiation of circus movement tachycardia.
a refractory state. When the coupling interval is reduced to 250 msec, both A-H and H-V prolongation allow conduction to proceed to the ventricles, with the subsequent development of reentry over the left-sided bypass tract. Stimulation from the coronary sinus also allowed the left atrial insertion site of the bypass tract to recover excitability earlier than if right atrial stimulation had been employed. This was an additional factor in facilitating initiation of circus movement tachycardia.
Initiation of orthodromic circus movement tachycardia by ventricular stimulation is possible in 80% of patients. Modes of initiation and incidence of initiation are identical to tachycardia induction by ventricular stimulation in patients with concealed bypass tracts (see Chapter 8). Several patterns of V-A conduction can be observed during ventricular pacing and ventricular extrastimuli:38,66,67 (a) Conduction over the accessory pathway alone (the most common pattern at short paced cycle lengths and short coupling intervals); the V-A conduction time is the same over a wide range of VPD coupling intervals and paced cycle lengths in the absence of intraventricular conduction delay or the presence of additional bypass tracts; (b) conduction over both the accessory pathway and the A-V conducting system; this is particularly common when right ventricular pacing is performed at long drive cycle lengths or right ventricular extrastimuli are delivered at long coupling intervals in patients with left-sided bypass tracts. This occurs because it is easier to engage the right bundle branch and conduct retrogradely through the A-V node than it is to reach a distant left-sided bypass tract. The atrial activation pattern depends on the relative refractoriness and conduction times over both pathways and usually exhibits a variable degree of atrial fusion. If the retrograde refractory period and/or conduction time of any of the components of the A-V conducting system exceeds that
of the bypass tract, retrograde atrial activation over the bypass tract will be favored; (c) conduction over the A-V conducting system alone; that is, no retrograde conduction occurred over the bypass tract, resulting in a normal pattern of V-A conduction; (d) absent V-A conduction over both the normal A-V conducting system and the bypass tract; rapid ventricular pacing, which produces progressive decremental conduction in the A-V node and prolongation of retrograde A-V nodal refractoriness, almost always results in retrograde atrial activation over the accessory pathway or none at all.
of the bypass tract, retrograde atrial activation over the bypass tract will be favored; (c) conduction over the A-V conducting system alone; that is, no retrograde conduction occurred over the bypass tract, resulting in a normal pattern of V-A conduction; (d) absent V-A conduction over both the normal A-V conducting system and the bypass tract; rapid ventricular pacing, which produces progressive decremental conduction in the A-V node and prolongation of retrograde A-V nodal refractoriness, almost always results in retrograde atrial activation over the accessory pathway or none at all.
Orthodromic SVT can be initiated by ventricular stimulation only if retrograde conduction to the atrium proceeds solely over the bypass tract, and the A-V node or His–Purkinje system can recover from any retrograde concealed conduction produced by ventricular stimuli, to conduct antegradely.
Because retrograde conduction over the bypass tract is all-or-none and retrograde refractory periods of the bypass tract are usually extremely short, the prime determinant for developing orthodromic SVT in response to ventricular stimulation is the extent of retrograde conduction and/or concealment in the normal pathway. Exclusive retrograde atrial activation over the bypass tract is necessary to initiate SVT, while three potential responses can occur in a normal A-V conducting system: (a) block in the His–Purkinje system; (b) block with or without concealment in the A-V node; or (c) block in the bypass tract with retrograde conduction over the normal conducting system to the His bundle resulting in a bundle branch reentrant complex (see Chapter 2) which subsequently leads to the initiation of SVT. The most common mode of initiation with ventricular extrastimuli is pattern 1, in which block in the His–Purkinje system occurs.66 Ventricular stimulation during sinus rhythm or at long paced cycle lengths almost invariably results in block in the His–Purkinje system with retrograde conduction over the bypass tract. Conduction to the ventricle over the A-V conducting system then will depend on antegrade conduction time over the A-V conducting system and ventricular refractoriness. Because block in the His–Purkinje system occurs in response to the ventricular extrastimulus, the atrial response will return to the ventricle over the normal A-V conducting system with a short A-H interval. In this situation the H-V must be long enough to allow for recovery of ventricular refractoriness for the ventricle to be reexcited. When prolongation of the H-V interval is required to initiate SVT, it is almost invariably associated with a QRS manifesting left bundle branch block (LBBB) during RV stimulation and right bundle branch block (RBBB) during LV stimulation (Figs. 10-20 to 10-22). While the H-V prolongation in Figure 10-20 provides enough time to allow the ventricles to
recover, the shorter amount of H-V prolongation shown in Figures 10-21 and 10-22 suggests that the intraventricular conduction delay produced by LBBB aberration associated with the first echo provides the critical amount of delay, which allows for atrial reexcitation over the bypass tract to initiate the tachycardia. In Figure 10-20 perpetuation of the SVT is ensured by marked A-V nodal conduction delay associated with dual A-V nodal pathways. In Figure 10-22 perpetuation of the tachycardia probably also requires the LBBB pattern, because both the A-H and H-V intervals remain fairly short.
Whenever the tachycardia is initiated by development of block in the His–Purkinje system, the first A-H of the tachycardia will be shorter than subsequent A-Hs because no concealment occurred in the A-V node during the initiating impulse.
recover, the shorter amount of H-V prolongation shown in Figures 10-21 and 10-22 suggests that the intraventricular conduction delay produced by LBBB aberration associated with the first echo provides the critical amount of delay, which allows for atrial reexcitation over the bypass tract to initiate the tachycardia. In Figure 10-20 perpetuation of the SVT is ensured by marked A-V nodal conduction delay associated with dual A-V nodal pathways. In Figure 10-22 perpetuation of the tachycardia probably also requires the LBBB pattern, because both the A-H and H-V intervals remain fairly short.
Whenever the tachycardia is initiated by development of block in the His–Purkinje system, the first A-H of the tachycardia will be shorter than subsequent A-Hs because no concealment occurred in the A-V node during the initiating impulse.
At shorter paced cycle lengths, with or without ventricular extrastimuli, penetration into the A-V node usually occurs, producing some retrograde A-V nodal concealment. In such cases, when the impulse goes over the bypass tract to the atrium and then reexcites the ventricle over the normal A-V conducting system, A-V nodal delay will occur, and the first A-H interval of the tachycardia will be longer than subsequent A-H intervals. This uncommonly occurs with ventricular extrastimuli delivered at paced drive cycle lengths ≥500 msec. During rapid ventricular pacing, one can see retrograde block in the normal conducting system either in the His–Purkinje system or the A-V node. When block occurs at the initiation of pacing, it is frequently in the His–Purkinje system, because the first or second paced complex usually acts as a long short interval producing V-H delay and/or block. This is clearly shown in Figure 10-23. Pacing is initiated at a cycle length of 400 msec, but the first paced complex occurs 800 msec following the last sinus complex. The second paced complex is associated with a long V-H interval owing to block in the right bundle branch retrogradely with conduction over the left bundle branch system (see Chapter 2). Simultaneously, the ventricular stimulus conducts solely over a left-sided bypass tract to the atrium. SVT does not develop because of retrograde concealment in the A-V node. Following the third paced complex, complete block in the His–Purkinje system occurs, and an antegrade His bundle deflection follows atrial activation, which resulted from conduction over the bypass tract. The fourth paced complex preempted the ability to see SVT, which would have been initiated at that time, but orthodromic SVT is clearly observed following the fourth paced complex because its rate was faster than the pacing rate.
Initiation of orthodromic SVT by mechanism 3, that is, following bundle branch reentry, is very common, particularly at long ventricular paced cycle lengths. In this instance, retrograde block usually occurs in the bypass tract and conduction proceeds over the normal A-V conducting system to induce a bundle branch reentrant complex. The long H-V often associated with this complex plus the LBBB pattern facilitate induction of SVT using a left-sided bypass tract (Fig. 10-24). This mode of initiation can be seen in approximately one-third of patients. Multiple modes of initiation may be observed in patients with orthodromic SVT. This depends on the paced cycle lengths used, the sites of atrial and/or ventricular stimulation, and the conduction velocity and refractoriness of the bypass tract and normal A-V conducting systems at the time of the study.38,68 Although His bundle stimulation cannot be, and has not been, systematically studied as a mode of initiation of tachycardias in patients with WPW, spontaneous initiation of orthodromic tachycardia has been observed with His bundle extrasystoles.39 In this instance, the His bundle extrasystole blocks retrogradely in the A-V node and conducts antegradely to the ventricles to retrogradely conduct over the bypass tract, reexcite the atrium, and return to the ventricles over the normal A-V conducting system. In this case, owing to retrograde concealment, the first A-H interval of the tachycardia will usually be slightly longer than that of subsequent complexes (Fig. 10-25).
Preexcited Tachycardias
Preexcited circus movement tachycardias are much less frequent, perhaps occurring spontaneously in 5% to 10% of
patients. Even this small percentage may be an overestimate, because not all regular wide-complex tachycardias associated with the WPW syndrome are due to antidromic tachycardia. In fact, Benditt et al.40 have shown that nearly 60% of regular wide-complex tachycardias are due to atrial flutter. Our experience is virtually identical. Moreover, many of these wide-complex tachycardias are not studied in the electrophysiology laboratory, and even when those patients with wide-complex tachycardias are evaluated, proof that the mechanism is circus movement antidromic tachycardia is not always available. Initiation of preexcited tachycardias in the laboratory is at least twice as frequent as their spontaneous occurrence. The causes of regular preexcited tachycardias are given in Table 10-1. Antidromic tachycardia is the most common mechanism of preexcited tachycardias in which the accessory pathway participates in the reentrant circuit. This tachycardia uses the accessory pathway anterogradely and the normal A-V conducting system retrogradely. Classically, initiation of true antidromic tachycardia by an APD requires (a) intact conduction over the bypass tract, (b) antegrade block in the A-V node or His–Purkinje system, and (c) intact retrograde conduction over the His–Purkinje system and A-V node, which assumes recovery of excitability of the A-V node for retrograde conduction following partial antegrade penetration. This latter requirement is a limiting factor for this “classic” mode of initiation of this arrhythmia by APDs. This limitation suggests that several mechanisms of initiation and types of preexcited tachycardias must be operative and include the following:
patients. Even this small percentage may be an overestimate, because not all regular wide-complex tachycardias associated with the WPW syndrome are due to antidromic tachycardia. In fact, Benditt et al.40 have shown that nearly 60% of regular wide-complex tachycardias are due to atrial flutter. Our experience is virtually identical. Moreover, many of these wide-complex tachycardias are not studied in the electrophysiology laboratory, and even when those patients with wide-complex tachycardias are evaluated, proof that the mechanism is circus movement antidromic tachycardia is not always available. Initiation of preexcited tachycardias in the laboratory is at least twice as frequent as their spontaneous occurrence. The causes of regular preexcited tachycardias are given in Table 10-1. Antidromic tachycardia is the most common mechanism of preexcited tachycardias in which the accessory pathway participates in the reentrant circuit. This tachycardia uses the accessory pathway anterogradely and the normal A-V conducting system retrogradely. Classically, initiation of true antidromic tachycardia by an APD requires (a) intact conduction over the bypass tract, (b) antegrade block in the A-V node or His–Purkinje system, and (c) intact retrograde conduction over the His–Purkinje system and A-V node, which assumes recovery of excitability of the A-V node for retrograde conduction following partial antegrade penetration. This latter requirement is a limiting factor for this “classic” mode of initiation of this arrhythmia by APDs. This limitation suggests that several mechanisms of initiation and types of preexcited tachycardias must be operative and include the following:
![]() FIGURE 10-24 Initiation of orthodromic tachycardia by bundle branch reentry. At a paced cycle length of 600 msec, a ventricular extrastimulus delivered at an S1-S2 of 250 msec results in retrograde block in a left lateral bypass tract and initiation of a bundle branch reentrant complex (see Chapter 2). The long H-V and LBBB morphology associated with the bundle branch reentrant complex allow the left-sided bypass tract time to recover, allowing retrograde excitation and initiation of SVT. LLRA, low lateral right atrium. |
TABLE 10-1 Mechanisms of Regular Preexcited Tachycardias | |
---|---|
|
An APD that blocks in the A-V node with antegrade conduction down a bypass tract and subsequent retrograde conduction through the normal A-V conducting system can occur. We have performed A-V pacing at a cycle length of 600 msec with an A-V interval of 120 msec and introduced APDs that block in the node. When we introduced a right ventricular extrastimulus with an A-V interval of 120 msec following the APD that blocked the node, retrograde conduction to the atrium never occurred. The right ventricular extrastimulus had to be delivered at A-V intervals of ≥200 msec for the A-V node to recover to allow retrograde conduction to the atrium (Fig. 10-26). Perhaps ventricular stimulation at a site farther from the His–Purkinje system would have been associated with a longer V-H interval, and retrograde conduction would have occurred. This may in fact be the case during antegrade preexcitation because ventricular excitation begins at the ventricular insertion site at the mitral or tricuspid annuli, which are farther from the conduction system than when stimulation is performed at the right ventricular apex. This may provide an additional 50 msec delay to allow the A-V node to recover for retrograde conduction, but this may not be enough time unless the A-V node has a short retrograde refractory period and/or rapid conduction. Nevertheless, because in antidromic SVT, the A-V interval remains relatively short, this first mechanism of initiation must be uncommon except with left lateral bypass tracts, which would potentially provide enough V-H delay to allow retrograde conduction. Alternatively, induction of antidromic tachycardia by APDs would be facilitated by a short retrograde A-V nodal refractory period (see subsequent discussion), a common finding in these tachycardias.
Lehmann et al.69 looked at a selected population with rapid retrograde A-V nodal conduction and reached similar conclusions. However, although we did not observe (V-A) conduction when we delivered right ventricular extrastimuli at ≥120 msec after the APD that blocked in the A-V node, early APDs that block proximally in the A-V node (near the atrial-A–V node junction) may have allowed the tissue to recover excitability and to support antidromic reentry. Alternatively, the same study performed at long drive cycle lengths could have resulted in proximal intraventricular and/or interventricular delays that allowed earlier A-V nodal recovery. As a result of long cycle lengths during sinus rhythm, an APD that blocks in the A-V node and conducts antegradely over a bypass tract will likely manifest “effective” retrograde His–Purkinje delay. The prolonged V-H intervals that usually are observed may represent intraventricular and/or interventricular conduction delay as well as delay in the ipsilateral or contralateral bundle branch used for retrograde conduction. This delay provides the A-V node time to recover. In our experience as well as that of Lehmann et al.69 and Packer et al.,70 the delay from the atrial insertion of the bypass tract to the retrograde His deflection must be at least 150 msec for initiation
of antidromic tachycardia. This is most likely to occur with left lateral bypass tracts, which are the sites of bypass tracts most frequently involved in true antidromic tachycardias (Fig. 10-27). Another potential mechanism of induction of a preexcited tachycardia with a His deflection inscribed shortly after the QRS is that the APD blocks below the His and conducts anterogradely down a contralateral accessory pathway. Retrograde conduction over an ipsilateral pathway would complete the circuit. His–Purkinje refractoriness would limit the ability to return over the normal conducting system. It is always important to prove that the recorded His potential is retrogradely activated; this is confirmed by
a fixed V-H interval during incremental atrial pacing and/or a similar V-H interval when pacing from the ventricular insertion site of the bypass tract (Fig. 10-27B and C).
Retrograde A-V nodal conduction in these patients therefore must be excellent. In fact, in patients with true antidromic tachycardia, retrograde A-V nodal conduction is remarkably good, with the majority of patients manifesting true antidromic tachycardia exhibiting 1:1 retrograde conduction over the A-V node at paced cycle lengths of 300 msec. Packer et al.70 found that all patients with true antidromic tachycardia had 1:1 retrograde conduction over the A-V node at paced cycle lengths of 360 msec; 23/30 had 1:1 conduction at paced cycle lengths ≤300 msec; and 16/30 had 1:1 conduction at paced cycle lengths of 260 msec. In addition, we, and they have observed that retrograde A-V nodal conduction is frequently faster than antegrade A-V nodal conduction during orthodromic tachycardia (Table 10-2). Unlike Packer et al.,70 however, we have found that dual A-V nodal physiology is common in these patients and that retrograde conduction over the fast pathway is better than antegrade slow pathway conduction, as it is in patients with AV nodal reentry. Retrograde conduction can also proceed over the slow pathway, resulting in a longer V-A interval and slower tachycardia. We also have documented several cases of AV nodal reentrant SVT with an innocent bystander accessory pathway in whom the mechanism had been assumed to be antidromic tachycardia.42,71 (This will be discussed subsequently.)
An APD that blocks in the node and conducts antegradely down one bypass tract and returns retrogradely over a different bypass tract to initiate a preexcited tachycardia (Fig. 10-28): Subsequent complexes may conduct anterogradely down or retrogradely up the normal A-V conducting system. Changing tachycardia cycle lengths may relate to whether retrograde conduction proceeds up a second bypass tract or up the A-V node (i.e., different routes of retrograde conduction).
TABLE 10-2 Electrophysiologic Substrate of Antidromic Tachycardia
- Good retrograde A-V nodal conduction
- Bypass tract is usually free wall
- Cycle length usually shorter than CMT using two bypass tracts except when retrograde conduction occurs over a slow A-V nodal pathway
- Good retrograde A-V nodal conduction
An APD that conducts over the bypass tract and simultaneously over the slow pathway of a dual A-V nodal pathway situation: Conduction beyond the His bundle to the ventricles is not possible owing to ventricular inexcitability, yet an A-V nodal echo to the atrium can occur. This atrial echo can then go down the bypass tract antegradely, when the ventricles will have recovered excitability, and initiate a preexcited tachycardia. An example of an APD with such 1:2 conduction owing to dual pathways initiating A-V nodal reentry with an innocent bystander bypass tract is shown in Figure 10-29. A-V nodal reentry may or may not persist or be preempted by retrograde conduction up the fast A-V nodal pathway caused by premature ventricular excitation over the bypass tract. In that situation the location of His potentials would depend on whether or not they were antegrade or retrograde. While this situation is theoretically possible, I have never seen it. One could distinguish A-V nodal reentry from classic antidromic reentry, which uses the His–Purkinje system retrogradely, by analyzing the H-A intervals during documented V-A conduction over the normal pathway during ventricular pacing and that during initiation and maintenance of the tachycardia. One must recognize, however, that if ventricular pacing is initiated during sinus rhythm, the H-A interval may not be due to retrograde conduction over the node but may be due to retrograde conduction exclusively over the bypass tract or fusion over the normal system and the bypass tract. Therefore, I prefer to analyze the H-A interval during entrainment of the tachycardia to eliminate the possibility of a fusion of atrial activation. As noted in Chapter 8, the H-A interval during A-V nodal echoes or A-V nodal reentry would be shorter than that resulting from retrograde V-A conduction over the normal conduction system in response to ventricular pacing (Fig. 10-30). In our experience, H-A intervals of ≤60 msec were never seen in true antidromic tachycardias. A more sensitive and specific criterion is the difference in the H-A interval observed during ventricular pacing (in sinus rhythm or during entrainment of the preexcited tachycardia) and the H-A interval during the preexcited tachycardia.71 In A-V nodal reentry, the difference is always >0 msec, while in other preexcited tachycardias, it is always ≤0 msec (Fig. 10-31). Another finding consistent with AVNRT is the failure of an APD delivered during a preexcited tachycardia at the time the atrium at the A-V junction (AVJ) is refractory to affect the H-H and A-A even though it advances the preexcited V (Fig. 10-32). This response is also seen in atrial tachycardia. When true antidromic tachycardia or a preexcited tachycardia using two accessory pathways is present, a similarly timed APD will advance the V and subsequently A, demonstrating the A is caused by the V (Fig. 10-33). Electrophysiologic features of preexcited tachycardias due to A-V nodal reentry are shown in Table 10-3.
Furthermore, in my experience, when atrial stimulation induces preexcited tachycardias, multiple bypass tracts are often operative. Whether or not they are operative throughout the tachycardia (i.e., integral components of the reentrant circuit) depends on (a) the relative retrograde activation times over additional bypass tracts and the normal A-V conducting system and (b) the varying degrees of antegrade and/or retrograde concealment into the additional bypass tracts and/or the normal A-V conduction system during the tachycardia.
APDs with 1:2 conduction with A-V nodal echoes provide an initiating mechanism of preexcited tachycardias that has heretofore been unrecognized. Initiation of A-V nodal reentry with innocent bystander preexcited tachycardias by ventricular premature complexes (VPCs) is also possible (Fig. 10-34). The ability to induce A-V nodal reentry by VPDs in these patients is higher than in the general population of patients with A-V nodal reentry (see Chapter 8). This, however may represent a patient selection bias of patients with preexcited tachycardia, since the only reason the tachycardia was seen was because it was inducible. In addition, in the presence of a bypass tract, if A-V nodal reentry is induced with block below the His, a preexcited tachycardia can ensue (Fig. 10-34). The actual frequency of apparent antidromic tachycardia that is due to A-V nodal reentry with passive conduction over a bypass tract is unknown. Proof of A-V nodal reentry as the mechanism of preexcited tachycardias may be difficult. The most clear-cut proof would be demonstration of A-V nodal reentry following spontaneous or drug-induced block in the bypass tract at the same cycle length, with the same H-A interval, and the same retrograde activation sequence as during the preexcited tachycardia (Fig. 10-35). Other criteria supporting A-V nodal reentry are the induction of A-V nodal reentry by atrial or ventricular extrastimuli with subsequent conduction over the bypass tract owing to His–Purkinje delay or block, with no change in the atrial activation sequence or H-A. A more detailed description of A-V nodal reentry with innocent bystander bypass tract is given later in this section.
Although APDs producing antegrade block in the node, antegrade conduction over the bypass tract, and retrograde conduction over the normal His–Purkinje system with long V-H intervals can initiate antidromic tachycardia,41 this has been surprisingly uncommon as the mechanism of preexcited circus movement tachycardias. Approximately 50% of our patients in whom atrial stimulation has induced a preexcited tachycardia have had true antidromic tachycardias. The remainder have had either preexcited tachycardias using multiple bypass tracts or A-V nodal reentry with an innocent bystander bypass tract. An example of an APD initiating a preexcited tachycardia using multiple bypass tracts is shown in Figure 10-28. In this patient, an anterior paraseptal bypass tract is present during sinus rhythm. Atrial stimulation from the high-right atrium is initiated at a paced cycle length of 400 msec. The first atrial extrastimulus blocks in the right anterior paraseptal bypass tract and conducts over a left lateral bypass tract. Before the next stimulus, retrograde conduction is manifest over the previously blocked right anterior bypass tract. The presence of His potentials during this tachycardia probably represents simultaneous antegrade conduction over
the normal A-V conducting system as conduction proceeds over the left lateral bypass tract. This is supported by the fact that the fourth atrial extrastimulus terminates the tachycardia by retrograde block in the right anterior bypass tract owing to the premature capture of the ventricles over the left-sided pathway (in the absence of a His deflection) and anterograde concealment into the right-sided pathway. The failure to see a His deflection implies that the extrastimulus either blocked in the AV node or was associated with AV nodal delay resulting in obscuration of the His by the preexcited QRS.
the normal A-V conducting system as conduction proceeds over the left lateral bypass tract. This is supported by the fact that the fourth atrial extrastimulus terminates the tachycardia by retrograde block in the right anterior bypass tract owing to the premature capture of the ventricles over the left-sided pathway (in the absence of a His deflection) and anterograde concealment into the right-sided pathway. The failure to see a His deflection implies that the extrastimulus either blocked in the AV node or was associated with AV nodal delay resulting in obscuration of the His by the preexcited QRS.
In our experience, “classic” antidromic tachycardias using a single A-V bypass tract can also be initiated by ventricular
stimulation. In this instance, retrograde block in the bypass tract must occur and retrograde conduction proceeds only over the normal A-V conduction system. This is manifested by a prolonged V-H-A activation sequence, which subsequently initiates the tachycardia with antegrade conduction down the bypass tract and repetitive retrograde conduction up the normal pathway (Fig. 10-36). This mechanism is most likely to occur in patients with an antegrade conducting only pathway or one with poor retrograde conduction. The V-A and, more specifically, H-A times in “classic” antidromic tachycardia are longer than in typical A-V nodal reentry with an innocent bystander bypass tract. As noted earlier, we have never observed H-A intervals ≤60 msec in true antidromic tachycardia, whereas one sees such H-A intervals in the majority of A-V nodal tachycardias. The value of the difference in H-A intervals during ventricular pacing and the tachycardia has been discussed earlier (see Figs. 10-30 and 10-31).
stimulation. In this instance, retrograde block in the bypass tract must occur and retrograde conduction proceeds only over the normal A-V conduction system. This is manifested by a prolonged V-H-A activation sequence, which subsequently initiates the tachycardia with antegrade conduction down the bypass tract and repetitive retrograde conduction up the normal pathway (Fig. 10-36). This mechanism is most likely to occur in patients with an antegrade conducting only pathway or one with poor retrograde conduction. The V-A and, more specifically, H-A times in “classic” antidromic tachycardia are longer than in typical A-V nodal reentry with an innocent bystander bypass tract. As noted earlier, we have never observed H-A intervals ≤60 msec in true antidromic tachycardia, whereas one sees such H-A intervals in the majority of A-V nodal tachycardias. The value of the difference in H-A intervals during ventricular pacing and the tachycardia has been discussed earlier (see Figs. 10-30 and 10-31).
![]() FIGURE 10-33 Response of antidromic tachycardia to APDs. A preexcited tachycardia using a left lateral bypass tract is present in the same patient shown in Figure 10-32, but in this case the QRS is maximally preexcited. The APD is again delivered from the distal CS, but it results in an exact capture of the V and A, providing a link between retrograde and antegrade conduction. This is consistent with true antidromic tachycardia or a preexcited tachycardia using a second bypass tract for the retrograde limb. (see text for discussion) |
TABLE 10-3 Characteristics of A-V Nodal Reentry with an Innocent Bystander Atrioventricular Bypass Tract | |
---|---|
|
I believe that AV nodal reentry with innocent bystander bypass tract is a more frequent cause of preexcited reentrant
tachycardia than previously recognized. Features are listed in Table 10-3. We have documented A-V nodal reentry as the mechanism of 13 of 56 cases of preexcited tachycardias, unrelated to atrial tachyarrhythmias which I have studied over the last 40 years. The diagnosis of A-V nodal reentry as the mechanism of the tachycardia primarily depended on the persistence of typical A-V nodal reentry with and without activation over a bypass tract. In six cases, this was due to block in the bypass tract by antiarrhythmic agents with persistence of a tachycardia with an identical H-A interval and
atrial activation sequence, all of which were compatible with A-V nodal reentry (Fig. 10-35). In other instances, typical A-V nodal reentry, induced by APDs or VPDs, subsequently developed into a preexcited tachycardia with the appearance of delay and/or block below the His bundle with persistence of the preexcited tachycardia having an identical H-A interval and atrial activation sequence (10–34). In addition, the initiation of A-V nodal reentry and subsequent preexcited tachycardia with 1:2 conduction in response to an atrial premature beat, as demonstrated in Figure 10-29, provides supportive evidence of bystander bypass tract. Other indirect evidence supporting A-V nodal reentry follows: (a) the demonstration that the H-A interval during ventricular pacing, either in sinus rhythm or, preferably, during entrainment of the preexcited tachycardia, exceeds the H-A interval during the tachycardia (Fig. 10-37) and (b) initiation of preexcited tachycardia by ventricular extrastimuli with a longer H-A interval than during the preexcited tachycardia despite the longer H-H interval before initiation of the tachycardia (Fig. 10-38).
tachycardia than previously recognized. Features are listed in Table 10-3. We have documented A-V nodal reentry as the mechanism of 13 of 56 cases of preexcited tachycardias, unrelated to atrial tachyarrhythmias which I have studied over the last 40 years. The diagnosis of A-V nodal reentry as the mechanism of the tachycardia primarily depended on the persistence of typical A-V nodal reentry with and without activation over a bypass tract. In six cases, this was due to block in the bypass tract by antiarrhythmic agents with persistence of a tachycardia with an identical H-A interval and
atrial activation sequence, all of which were compatible with A-V nodal reentry (Fig. 10-35). In other instances, typical A-V nodal reentry, induced by APDs or VPDs, subsequently developed into a preexcited tachycardia with the appearance of delay and/or block below the His bundle with persistence of the preexcited tachycardia having an identical H-A interval and atrial activation sequence (10–34). In addition, the initiation of A-V nodal reentry and subsequent preexcited tachycardia with 1:2 conduction in response to an atrial premature beat, as demonstrated in Figure 10-29, provides supportive evidence of bystander bypass tract. Other indirect evidence supporting A-V nodal reentry follows: (a) the demonstration that the H-A interval during ventricular pacing, either in sinus rhythm or, preferably, during entrainment of the preexcited tachycardia, exceeds the H-A interval during the tachycardia (Fig. 10-37) and (b) initiation of preexcited tachycardia by ventricular extrastimuli with a longer H-A interval than during the preexcited tachycardia despite the longer H-H interval before initiation of the tachycardia (Fig. 10-38).
All our patients with A-V nodal reentry demonstrated shorter H-A intervals during the preexcited tachycardia than during ventricular pacing at similar rates. In our patients, atrial stimulation during the preexcited tachycardia could advance the preexcited QRS but was always associated with an apparent change in the V-A interval (Fig. 10-32). The V-A interval should change for one of several reasons: (a) “pseudo” prolongation of the VA due to a constant AA (i.e., AV nodal tachycardia remained unaffected); this is the most common mechanism. It can be easily demonstrated by delivering the APD at a time when the AVJ is refractory. This will result in advancement of the ventricle with a preexcited QRS without affecting the AV nodal tachycardia; (b) extremely premature atrial extrastimulus penetrates the A–V node, producing slower conduction down the slow pathway before resumption of the tachycardia, which, in the presence of a fixed A-V interval in response to the APD, would lead to a longer V-A interval; (c) the preexcited QRS complex could theoretically retrogradely enter the His–Purkinje system, capture the His retrogradely, and conduct up the fast pathway and reset A-V nodal reentry in that manner. If that were the case, for
the same V-A interval to be present, the retrograde conduction time (the V-H interval) of the preexcited QRS complex plus the H-A interval in response to this complex should add up to the same V-A interval of an undisturbed A-V nodal reentry. I personally have never seen the last mechanism, which is analogous to failure of a single, late VPD to affect a rapid AV nodal tachycardia (see Chapter 8). Even if this unlikely event occurred, the H-A interval of that preexcited complex would necessarily exceed that during the rest of the tachycardia. Observations that could exclude A-V nodal reentry are shown in Table 10-4.
the same V-A interval to be present, the retrograde conduction time (the V-H interval) of the preexcited QRS complex plus the H-A interval in response to this complex should add up to the same V-A interval of an undisturbed A-V nodal reentry. I personally have never seen the last mechanism, which is analogous to failure of a single, late VPD to affect a rapid AV nodal tachycardia (see Chapter 8). Even if this unlikely event occurred, the H-A interval of that preexcited complex would necessarily exceed that during the rest of the tachycardia. Observations that could exclude A-V nodal reentry are shown in Table 10-4.
![]() FIGURE 10-37 Use of ventricular pacing during preexcited tachycardia to recognize A-V nodal reentry. Surface leads 1, 2, and V1 are displayed with multiple intracardiac recordings. (See Fig. 10-32.) During ventricular pacing (first five complexes) the H-A interval is 55 msec. While on resumption of the preexcited tachycardia the H-A is 35 msec with an identical actual activation sequence. A longer H-A during RV pacing than during the tachycardia supports A-V nodal reentry with innocent bystander bypass tract as the mechanism of the preexcited tachycardia. See text for discussion. |
Cycle length alterations dependent on changing V-H intervals – thereby demonstrating requisite participation of the His-Purkinje system in the tachycardia circuit – are not uncommon in true antidromic tachycardias.41 This is one of the diagnostic features of true antidromic tachycardias and rules out AVNRT with an innocent bystander accessory pathway. We never saw this phenomenon in any of our patients with A-V nodal reentry and innocent bystander bypass tracts. Documentation of A-V nodal reentry as the underlying mechanism of a preexcited tachycardia is of critical importance in planning ablative therapy to cure the arrhythmia. As noted above the mere presence of an H-A interval of <70 msec should suggest A-V nodal tachycardia.
Thus, preexcited tachycardias have multiple mechanisms. The term antidromic tachycardia should be reserved for tachycardias that use an A-V bypass tract antegradely and the normal A-V conducting system retrogradely. A schema of some of the potential mechanisms of preexcited tachycardias
is given in Figure 10-39. The difficulty in recording clear retrograde or antegrade His potentials during atrial or ventricular stimulation in the presence of preexcitation makes establishment of the exact initiating and sustaining mechanisms of preexcited tachycardias difficult to ascertain. Nevertheless, in our experience, ∼23% of preexcited tachycardias are due to A-V nodal reentry with an innocent bystander bypass tract. In addition, many (almost 40%) of our patients with spontaneous reentrant preexcited tachycardias incorporating a bypass tract as the anterior limb have multiple bypass tracts (concealed or manifest), whether or not they are used as the retrograde limb. This is also true of preexcited tachycardias using an atriofascicular pathway. This is in concordance with the incidence of multiple bypass tracts reported by Gallagher et al.32 and Benditt et al.40
is given in Figure 10-39. The difficulty in recording clear retrograde or antegrade His potentials during atrial or ventricular stimulation in the presence of preexcitation makes establishment of the exact initiating and sustaining mechanisms of preexcited tachycardias difficult to ascertain. Nevertheless, in our experience, ∼23% of preexcited tachycardias are due to A-V nodal reentry with an innocent bystander bypass tract. In addition, many (almost 40%) of our patients with spontaneous reentrant preexcited tachycardias incorporating a bypass tract as the anterior limb have multiple bypass tracts (concealed or manifest), whether or not they are used as the retrograde limb. This is also true of preexcited tachycardias using an atriofascicular pathway. This is in concordance with the incidence of multiple bypass tracts reported by Gallagher et al.32 and Benditt et al.40
TABLE 10-4 Methods to Exclude A-V Nodal Reentry as a Cause of a Preexcited Tachycardia | |
---|---|
|
It is of interest, however, that patients with antidromic tachycardias, or more accurately, preexcited circus movement tachycardias, have shorter effective refractory periods of their A-V node and manifest accessory pathway, both antegradely and retrogradely, than a comparable group of control patients.70 Almost all patients with preexcited reciprocating tachycardias demonstrate antegrade conduction down a lateral (left or right) bypass tract (Figs. 10-27, 10-28, 10-32–10-35, 10-37, 10-38, 10-40). Retrograde conduction may occur over another bypass tract (either lateral or septal) or over the A-V node. We have never documented, nor have we encountered in literature, a “classic” antidromic tachycardia with antegrade conduction solely over a septal bypass tract. In our experience, antegrade conduction over a true septal bypass tract has occurred only during preexcited tachycardias using multiple bypass tracts. Tachycardia cycle lengths also appear to be shorter during preexcited tachycardias than during orthodromic tachycardias using the same bypass tract. In most of our patients with “classic antidromic tachycardia,” the cycle length was longer than the orthodromic tachycardia in the same patient. This may not be the case if dual A-V nodal physiology is present and if the orthodromic tachycardia uses the slow pathway antegradely while the antidromic
tachycardia uses the fast pathway retrogradely. This situation may explain why Packer et al.70 found the cycle length of antidromic tachycardia to be shorter than orthodromic tachycardia in the same patient. In our experience, preexcited tachycardias using two or more bypass tracts tend to have longer cycle lengths than orthodromic tachycardias or the “classic” antidromic tachycardia. This is due to the fact two bypass tracts are typically in opposite chambers and are incorporated in a larger reentrant circuit than one involving a midline, rapidly conducting A-V node.
tachycardia uses the fast pathway retrogradely. This situation may explain why Packer et al.70 found the cycle length of antidromic tachycardia to be shorter than orthodromic tachycardia in the same patient. In our experience, preexcited tachycardias using two or more bypass tracts tend to have longer cycle lengths than orthodromic tachycardias or the “classic” antidromic tachycardia. This is due to the fact two bypass tracts are typically in opposite chambers and are incorporated in a larger reentrant circuit than one involving a midline, rapidly conducting A-V node.
TABLE 10-5 Rate Changes During Preexcited Tachycardias Due to Changes in Retrograde Conduction | |
---|---|
|
Finally, it is important to recognize that preexcited tachycardias may be irregular, particularly classic antidromic tachycardia. Rate changes are most commonly due to changes in retrograde conduction (Table 10-5). This is often due to changing retrograde conduction over different fascicles, with different V-H intervals, in patients with classic antidromic tachycardia (Fig. 10-41).41 Obviously, one also may see such irregularities whenever different routes of retrograde and/or antegrade conduction use different bypass tracts. However, if the change in cycle length can be ascribed to a change in V-H and/or subsequent H-A intervals, it suggests that retrograde conduction occurs over the normal A-V conducting system (Fig. 10-42). This is evidence diagnostic of classic antidromic tachycardia. More rarely, rate changes can occur due to a change in A-V intervals; this may relate to decremental antegrade conduction of the bypass tract (Table 10-6). Carotid sinus stimulation may be of help in distinguishing the mechanism of preexcited tachycardias if the rhythm terminates (Table 10-7).
TABLE 10-6 Rate Changes During Regular Preexcited Tachycardias Due to Changes in Anterograde Conduction | |
---|---|
|
The site of stimulation not only plays an important role in inducibility of circus movement tachycardia (see preceding discussion) but also may determine the type of circus movement tachycardia. Wellens and Brugada38 (demonstrated that APDs at the same coupling intervals could induce antidromic or orthodromic tachycardia, depending on the stimulation site (Fig. 10-43). The closer the stimulation site is to the bypass tract, the more likely block in the bypass tract will occur and orthodromic tachycardia result. Conversely, antidromic tachycardia is more likely to occur with APDs delivered close to the A-V node.
Atrial Fibrillation
Atrial fibrillation occurs in approximately 50% of patients with WPW syndrome, and in approximately 10% is the presenting arrhythmia (although perhaps not the initial arrhythmia). Its clinical importance is the fact that extremely rapid
rates can occur over the bypass tract leading to ventricular fibrillation (Fig. 10-44A). In our experience, no clinical features or bypass tract locations distinguish patients with preexcitation and atrial fibrillation from those without atrial fibrillation. Although patients with atrial fibrillation have somewhat shorter bypass tract refractory periods than those with orthodromic SVT only (260 vs. 305 msec), overlap is considerable. Only those patients who present with a cardiac arrest have significantly shorter antegrade refractory periods (mean, 225 msec). Whereas patients with atrial fibrillation have a higher incidence of inducible circus movement tachycardia than those who are asymptomatic, the cycle length of induced tachycardias is similar in both groups. We also have not found atrial pressure during circus movement tachycardia to differ in the two groups. As stated earlier, for unclear reasons, patients with overt preexcitation do have a higher incidence of atrial fibrillation than those with concealed bypass tracts despite comparable bypass tract locations and tachycardia cycle lengths. Although this suggests a relationship between specific atrial insertion sites of the bypass tract and the genesis of atrial fibrillation, we have not found this to be the case.
rates can occur over the bypass tract leading to ventricular fibrillation (Fig. 10-44A). In our experience, no clinical features or bypass tract locations distinguish patients with preexcitation and atrial fibrillation from those without atrial fibrillation. Although patients with atrial fibrillation have somewhat shorter bypass tract refractory periods than those with orthodromic SVT only (260 vs. 305 msec), overlap is considerable. Only those patients who present with a cardiac arrest have significantly shorter antegrade refractory periods (mean, 225 msec). Whereas patients with atrial fibrillation have a higher incidence of inducible circus movement tachycardia than those who are asymptomatic, the cycle length of induced tachycardias is similar in both groups. We also have not found atrial pressure during circus movement tachycardia to differ in the two groups. As stated earlier, for unclear reasons, patients with overt preexcitation do have a higher incidence of atrial fibrillation than those with concealed bypass tracts despite comparable bypass tract locations and tachycardia cycle lengths. Although this suggests a relationship between specific atrial insertion sites of the bypass tract and the genesis of atrial fibrillation, we have not found this to be the case.
TABLE 10-7 Effect of Carotid Sinus Pressure on Preexcited Tachycardias | |
---|---|
|
The most common mechanism by which atrial fibrillation spontaneously develops is degeneration from circus movement tachycardia (Fig. 10-44B). This may explain the similarity of bypass tract refractory periods of patients presenting with SVT and atrial fibrillation. Of interest, in most cases, initiation of atrial fibrillation begins at sites usually far removed from the atrial insertion of the bypass tract. This observation has been observed by others.72,73,74,75 Recent observations in our laboratory suggest that the circus movement tachycardias induce pulmonary vein or other focal triggers of atrial fibrillation. This makes one wonder what the real role of the bypass tract is in atrial fibrillation.
Induction of atrial fibrillation in the laboratory with atrial premature beats is similar to induction of atrial fibrillation in
patients with atrial fibrillation without preexcitation. The electrophysiologic substrate (prolonged intra-atrial conduction in response to APDs, failure of refractoriness to adapt to changes in cycle length, and higher percent of fractionated electrograms) is discussed in Chapter 9. Vulnerability to induction of >1 minute of atrial fibrillation by one or two APDs is observed in 50% to 85% of patients presenting with atrial fibrillation compared to <10% in patients without atrial fibrillation.45,46 As noted above, conduction delays in response to late coupled APDs are similar to those observed in patients with lone atrial fibrillation in the absence of preexcitation. The significance of a shorter anterograde refractory period of the bypass tract in patients with atrial fibrillation may have to do with the necessity of earlier APDs required to produce block in the bypass tract to initiate SVT. The earlier APD would be associated with greater atrial conduction delays and initiation of pulmonary vein triggers, which would predispose to atrial fibrillation. Circus movement orthodromic tachycardia is also associated with cannon A waves which may result in pulmonary vein triggers and enhanced vagal discharge. An interestingly common mode of induction of atrial fibrillation in patients with the WPW syndrome is by rapid ventricular pacing. In fact, in our experience, atrial fibrillation is more commonly induced by ventricular pacing than atrial pacing at comparable rates. For this particular mode of induction, intra-atrial pressure may play a role in induction of the arrhythmia. Because spontaneous induction of the arrhythmia from circus movement SVT is the most common mechanism, surgical and catheter ablation of the bypass tract apparently cures atrial fibrillation (at least over a 5-year period) in the vast majority (>90%) of
patients. Of some concern is the persistent vulnerability to atrial fibrillation that has been noted by Haissaguerre et al.46 of 56%. This may be due to easier formation of pulmonary vein triggers in these patients. Others have also noted persistent vulnerability.45 We have also observed that the response to APDs described above is also unaltered by surgical or catheter ablation. This is also consistent with the hypothesis that pulmonary vein triggers require an appropriate substrate in order to initiate atrial fibrillation; cure of SVT which appears to be a trigger of pulmonary vein firing may be what prevents atrial fibrillation in the short term. However removal of the initiating trigger does not affect the underlying substrate. Perhaps these people will develop lone atrial fibrillation at a later age. The answer to this problem will require a 20- to 40-year follow-up. In the patients who are not cured by ablative procedures, primary atrial abnormalities may be responsible for atrial fibrillation in a similar fashion as they are in patients without preexcitation. A study is required to evaluate whether the ability to initiate atrial fibrillation predicts a substrate that will not be changed by cure of WPW ablative therapy and hence, likely recurrence of fibrillation during long-term follow-up.
patients with atrial fibrillation without preexcitation. The electrophysiologic substrate (prolonged intra-atrial conduction in response to APDs, failure of refractoriness to adapt to changes in cycle length, and higher percent of fractionated electrograms) is discussed in Chapter 9. Vulnerability to induction of >1 minute of atrial fibrillation by one or two APDs is observed in 50% to 85% of patients presenting with atrial fibrillation compared to <10% in patients without atrial fibrillation.45,46 As noted above, conduction delays in response to late coupled APDs are similar to those observed in patients with lone atrial fibrillation in the absence of preexcitation. The significance of a shorter anterograde refractory period of the bypass tract in patients with atrial fibrillation may have to do with the necessity of earlier APDs required to produce block in the bypass tract to initiate SVT. The earlier APD would be associated with greater atrial conduction delays and initiation of pulmonary vein triggers, which would predispose to atrial fibrillation. Circus movement orthodromic tachycardia is also associated with cannon A waves which may result in pulmonary vein triggers and enhanced vagal discharge. An interestingly common mode of induction of atrial fibrillation in patients with the WPW syndrome is by rapid ventricular pacing. In fact, in our experience, atrial fibrillation is more commonly induced by ventricular pacing than atrial pacing at comparable rates. For this particular mode of induction, intra-atrial pressure may play a role in induction of the arrhythmia. Because spontaneous induction of the arrhythmia from circus movement SVT is the most common mechanism, surgical and catheter ablation of the bypass tract apparently cures atrial fibrillation (at least over a 5-year period) in the vast majority (>90%) of
patients. Of some concern is the persistent vulnerability to atrial fibrillation that has been noted by Haissaguerre et al.46 of 56%. This may be due to easier formation of pulmonary vein triggers in these patients. Others have also noted persistent vulnerability.45 We have also observed that the response to APDs described above is also unaltered by surgical or catheter ablation. This is also consistent with the hypothesis that pulmonary vein triggers require an appropriate substrate in order to initiate atrial fibrillation; cure of SVT which appears to be a trigger of pulmonary vein firing may be what prevents atrial fibrillation in the short term. However removal of the initiating trigger does not affect the underlying substrate. Perhaps these people will develop lone atrial fibrillation at a later age. The answer to this problem will require a 20- to 40-year follow-up. In the patients who are not cured by ablative procedures, primary atrial abnormalities may be responsible for atrial fibrillation in a similar fashion as they are in patients without preexcitation. A study is required to evaluate whether the ability to initiate atrial fibrillation predicts a substrate that will not be changed by cure of WPW ablative therapy and hence, likely recurrence of fibrillation during long-term follow-up.
Localization of the Bypass Tract
Although the initial suggestion of Rosenbaum et al.76 that bypass tracts could be divided into Type A and Type B (based on the major QRS forces in V1 and V2), the results of catheter endocardial and intraoperative epicardial mapping suggested a variety of locations of bypass tracts exists. Based on the results of epicardial mapping, Gallagher et al.32 and Tonkin et al.77 proposed 8 to 12 locations of bypass tracts, which could be recognized by the initial 40-msec vector of the delta wave during maximal preexcitation. Similar complex algorithms have been proposed in the “ablation” era to attempt to precisely localize the accessory pathway to 12 sites around the A-V valves as well as epicardial sites within the coronary sinus.78,79,80,81,82 They have actually attempted to distinguish mid-septal (right and left), para-Hisian, and anteroseptal bypass tracts, which arise within 1.5 cm of each other using ECG criteria.81,83 Although these electrocardiographic and vector-cardiographic criteria may be useful during maximal preexcitation, for the most part, during sinus rhythm, the QRS complex is a fusion and total preexcitation is not present. One cannot arbitrarily use the initial 20 or 40 msec of the QRS or the ratio of R/S in any lead in the absence of total preexcitation to pinpoint the location of a bypass tract. The site of transition of the precordial leads is also flawed by variable lead placement, variations of body shape and/or size, and variations in heart size, location in the chest, and extent of fibrosis or other disease process. As such, I believe a simple approach to regionalize accessory pathways is most reasonable. Lindsay et al.84 and Milstein et al.85 have developed more practical ECG criteria to localize the general region of bypass tracts. Milstein et al.85 divided bypass tracts into four general locations, which represent the four surgical approaches (anteroseptal, right free wall, posteroseptal, and left free wall) used to cure WPW. I prefer to divide the location of bypass tracts into five regions: an anteroseptal region, right free wall region, posteroseptal region, a posterior free wall region, and left lateral region. I do this because of significant differences in the ECG of left posterior free wall and true left lateral bypass tract. One could potentially divide the right free wall into two areas – anterior and lateral – but these bypass tracts are insufficiently common to justify this. The schema of locations that we use is shown in Figure 10-45. Basically, left lateral bypass tracts are characterized by negative delta waves in leads 1 and L and by positive delta waves in inferior leads and all the precordial leads. Left posterior (inferior) free wall bypass tracts usually have isoelectric delta waves in lead 1, negative delta waves in inferior leads, isoelectric or slightly positive (closer to posteroseptal location) delta in aVL, and strongly positive delta waves in the right precordial leads. This group may be difficult to distinguish from left posteroseptal bypass tracts, which have positive delta waves in 1 and L. Right posteroseptal bypass tracts are characterized by negative delta waves in the inferior leads (although lead 2 may be isoelectric or biphasic), a left superior axis, and an R/S ratio less than 1 in V1. In general the more negative the delta in lead 2 the more leftward the location. While Arruda et al.78 state that a negative delta in lead 2 suggests an epicardial CS location, this is not true in the majority of cases. I have seen many right inferior free wall accessory pathways with negative delta waves in leads 2, 3, and aVF. It is, however, true that the larger the negative delta wave in lead 3 relative to lead 2, the more likely this will be a posteroseptal bypass tract that is approachable from the right side. Right free wall bypass tracts generally have biphasic or negative delta waves in both V1 and V2, and positive delta waves in leads 1 and 2, with usually a variably negative delta wave in lead 3 depending on superior versus inferior location. Anteroseptal bypass tracts have positive delta waves in lead 1, inferiorly directed delta waves that are positive in lead 2 and aVF greater than lead 3, and precordial leads with primarily negative or biphasic (40 msec) delta waves. I agree
with Haissaguerre et al.83 that a purely negative delta wave in leads V1 and V2, when associated with a His deflection at the earliest site of ventricular activation (see below), and positive delta waves in leads 2 and aVF suggests a para-Hisian location of the accessory pathway; however, considerable overlap exists with anteroseptal bypass tracts. Right free wall pathways have negative or biphasic delta waves in V1–V3 and transition a little later than anteroseptal accessory pathways (V3).
with Haissaguerre et al.83 that a purely negative delta wave in leads V1 and V2, when associated with a His deflection at the earliest site of ventricular activation (see below), and positive delta waves in leads 2 and aVF suggests a para-Hisian location of the accessory pathway; however, considerable overlap exists with anteroseptal bypass tracts. Right free wall pathways have negative or biphasic delta waves in V1–V3 and transition a little later than anteroseptal accessory pathways (V3).
Analysis of retrograde P-wave morphology during SVT is also helpful in localizing the atrial insertion site of the bypass tracts used for retrograde conduction during SVT. Posteroseptal bypass tracts have inverted P waves in the inferior leads and positive P waves in aVR and aVL. Posterior left free wall bypass tracts have a simliar P-wave morphology in the inferior leads, but the P wave in aVR is more positive than in aVL (which is often isoelectric) and the P wave in lead 2 is more negative than the P wave in lead 3. Left lateral bypass tracts have negative P waves in leads 1 and aVL with a tendency for positive P waves in lead 3 to be greater than those in lead aVF, which are greater than those in lead 2 as the location of the bypass tracts moves more superiorly. In left superior atrial insertion sites the P wave will be more positive in lead 3 than in lead 2, while the opposite is true for right anterior free wall bypass tracts.
Despite claims as to the accuracy of a variety of schemas to precisely localize the ventricular, they all have many limitations and pitfalls. These include the influence of prior infarction, conduction defects, hypertrophy, drug and/or electrolyte imbalance, congenital heart disease, and postoperative changes, which can all influence the electrocardiogram. Moreover, the potential for multiple bypass tracts, the absence of maximal preexcitation, variable lead positions across the precordium, and variations in the position of the heart relative to the recording electrodes, make precise localization merely a guess. Nevertheless, the ECG can help regionalize the site of the bypass tract. Other techniques are required to more accurately and precisely identify the location of the bypass tract.
Several intracardiac mapping and stimulation techniques have been used: (a) determination of the relationship of the delta wave to local ventricular electrograms, (b) pacing from multiple atrial sites, (c) analysis of the retrograde atrial activation pattern during orthodromic tachycardia and/or ventricular stimulation, (d) evaluation of the effect of bundle branch block on V-A conduction during SVT, and (e) direct recording of electrical potentials from the bypass tract.
Relation of Local Ventricular Electrograms to Delta Wave
Theoretically, if one could map the ventricles along the mitral and tricuspid rings, the earliest site of ventricular activation and, therefore, the ventricular insertion of the bypass tract could be localized. Aside from technical considerations that may limit this approach, particularly in the left ventricle, whether the bypass tract is epicardial (they usually are) or truly endocardial may influence the relative timing of the local ventricular electrogram, because conduction across the muscle wall will be markedly influenced by anisotropic properties of the ventricle. Because the right ventricle bypass tracts are in some instances endocardial,86 analysis of multiple-site right ventricular electrograms extending from just cephalad to the tricuspid valve at the His bundle recording site and moving anterolaterally, laterally, and inferoposteriorly can provide considerable information. Use of a Halo catheter or other multipolar catheter to record circumferentially around the tricuspid annulus is extremely useful in regionalizing the bypass tract. Analysis of left ventricular electrograms in the coronary sinus recording provides similar information. We used quadripolar, hexapolar, octapolar, decapolar, and occasionally, dodecapolar catheters for coronary sinus recordings. When the entire coronary sinus cannot be recorded by the electrodes on a single catheter, we move the catheter within the coronary sinus to record a total of 8 to 12 sites. We record bipolar electrograms using either 2-mm or 5-mm interelectrode distances. We usually use all poles for adjacent recordings (i.e., we obtain recordings from poles 1 and 2, 2 and 3, 3 and 4, 4 and 5, and 5 and 6 in a hexapolar catheter) when electrodes have a 5-mm interelectrode distance. Unipolar recordings (unfiltered and filtered) are used to assess which pole is closest to the bypass tract. This is of particular value in guiding the ablation catheter. An example of this technique is shown in Figure 10-46, in which we used a decapolar catheter
(tenth pole not shown) in the coronary sinus. One can clearly see that the second bipolar pair provides the earliest electrogram in the filtered signal, and the unipolar recordings reveal that the second pole is the closest to the ventricular insertion site. Although the theoretical advantage of unipolar electrograms is the ability to distinguish contributions of individual poles, frequently the electrograms are so large, and the intrinsicoid deflections not easily determinable, that the ability to distinguish the fastest of a slow-moving waveform is problematic. This is particularly relevant when the coronary sinus is away from the annulus, a frequent problem in the posteroseptal and posterior region (on the atrial side of the annulus) and the distal coronary sinus (ventricular side of the annulus). The variability of the anatomic relationship of the coronary sinus and mitral annulus is an important limitation to the use of electrograms recorded in the coronary sinus to identify the atrial insertion site of the accessory pathway.87 The relationship of the earliest atrial activation and earliest ventricular activation also must be determined to determine the presence or absence of a slanted bypass tract (see subsequent discussion). As such, ultimately the recordings from the ablation catheter determine the site of ablation. As stated above, the use of both unipolar and bipolar recordings is important to precisely localize the ventricular insertion of the bypass tract for catheter ablation. It is critical to demonstrate that the tip electrode of the ablation/mapping catheter is recording the earliest activity since it will be the electrode through which radiofrequency energy is delivered. Recordings from the LV mapping electrode are compared to those in the coronary sinus to ensure recording of the earliest atrial activity and earliest ventricular activity. The ability to use the coronary sinus catheter to assess left ventricular preexcitation should not be neglected. The limitations imposed by anatomic variations apply for the ventricular insertion site as well, but are less significant. Normally, the basal aspects of the left ventricle recorded from the coronary sinus are activated late in the normal QRS complex. In the presence of a left-sided bypass tract, the base of the heart is activated early, simultaneously with the delta wave (Fig. 10-46). Early activation usually can be distinguished in one bipolar electrogram with an accuracy of 1 cm if 5-mm interelectrode distances are used. The accuracy improves to <5 mm when unipolar recordings are used. Obviously, the most precise method of determining the site of ventricular preexcitation is via a left ventricular mapping catheter. The ventricular insertion site usually has a close relationship with the atrial insertion site, as shown by comparing the site of antegrade preexcitation with retrograde atrial activation mapping (Fig. 10-47), although they may be disparate by up to 2 cm if the bypass tract crosses the A-V sulcus at an angle (see Chapter 14). Changes in local V-A interval due to changes in the wavefront of activation suggest a slanted bypass tract. In my experience, when a slanted bypass tract is present, the ventricular insertion is inferior to the atrial insertion when the bypass tracts are located at the lower half of the mitral and tricuspid annuli and superior to the atrial insertion when located superiorly (viewed in the LAO position). In both cases, the ventricular insertion is usually more medial than the atrial insertion. This has also been observed by Jackman (personal communication; Fig. 10-48). This is most often seen with left-sided bypass tracts when local activation in the CS is compared during LBBB, normal QRS, and RBBB. With LBBB the A and V merge, and with normal or RBBB QRSs’ they separate (Fig. 10-49 and see Chapter 14).
(tenth pole not shown) in the coronary sinus. One can clearly see that the second bipolar pair provides the earliest electrogram in the filtered signal, and the unipolar recordings reveal that the second pole is the closest to the ventricular insertion site. Although the theoretical advantage of unipolar electrograms is the ability to distinguish contributions of individual poles, frequently the electrograms are so large, and the intrinsicoid deflections not easily determinable, that the ability to distinguish the fastest of a slow-moving waveform is problematic. This is particularly relevant when the coronary sinus is away from the annulus, a frequent problem in the posteroseptal and posterior region (on the atrial side of the annulus) and the distal coronary sinus (ventricular side of the annulus). The variability of the anatomic relationship of the coronary sinus and mitral annulus is an important limitation to the use of electrograms recorded in the coronary sinus to identify the atrial insertion site of the accessory pathway.87 The relationship of the earliest atrial activation and earliest ventricular activation also must be determined to determine the presence or absence of a slanted bypass tract (see subsequent discussion). As such, ultimately the recordings from the ablation catheter determine the site of ablation. As stated above, the use of both unipolar and bipolar recordings is important to precisely localize the ventricular insertion of the bypass tract for catheter ablation. It is critical to demonstrate that the tip electrode of the ablation/mapping catheter is recording the earliest activity since it will be the electrode through which radiofrequency energy is delivered. Recordings from the LV mapping electrode are compared to those in the coronary sinus to ensure recording of the earliest atrial activity and earliest ventricular activity. The ability to use the coronary sinus catheter to assess left ventricular preexcitation should not be neglected. The limitations imposed by anatomic variations apply for the ventricular insertion site as well, but are less significant. Normally, the basal aspects of the left ventricle recorded from the coronary sinus are activated late in the normal QRS complex. In the presence of a left-sided bypass tract, the base of the heart is activated early, simultaneously with the delta wave (Fig. 10-46). Early activation usually can be distinguished in one bipolar electrogram with an accuracy of 1 cm if 5-mm interelectrode distances are used. The accuracy improves to <5 mm when unipolar recordings are used. Obviously, the most precise method of determining the site of ventricular preexcitation is via a left ventricular mapping catheter. The ventricular insertion site usually has a close relationship with the atrial insertion site, as shown by comparing the site of antegrade preexcitation with retrograde atrial activation mapping (Fig. 10-47), although they may be disparate by up to 2 cm if the bypass tract crosses the A-V sulcus at an angle (see Chapter 14). Changes in local V-A interval due to changes in the wavefront of activation suggest a slanted bypass tract. In my experience, when a slanted bypass tract is present, the ventricular insertion is inferior to the atrial insertion when the bypass tracts are located at the lower half of the mitral and tricuspid annuli and superior to the atrial insertion when located superiorly (viewed in the LAO position). In both cases, the ventricular insertion is usually more medial than the atrial insertion. This has also been observed by Jackman (personal communication; Fig. 10-48). This is most often seen with left-sided bypass tracts when local activation in the CS is compared during LBBB, normal QRS, and RBBB. With LBBB the A and V merge, and with normal or RBBB QRSs’ they separate (Fig. 10-49 and see Chapter 14).
Pacing from Multiple Atrial Sites
As discussed earlier, the closer the stimulation site is to the atrial insertion of the bypass tract, the more rapidly the impulse will reach the bypass tract relative to the A-V node. Thus, the greater the proximity to the atrial insertion site of the bypass tract during atrial pacing, the greater the degree of preexcitation and the shorter the P-to-delta-wave interval will be.32,88 This response occurs because the effects of intra-atrial and interatrial conduction delays are minimized, particularly when dealing with left-sided bypass tracts. For example, if activation of the atrial insertion of the bypass tract begins 80 msec after the high-right atrial depolarization, pacing the atrium at or near the site of the bypass tract will shorten that interval by approximately 80 msec. This will decrease the P-to-delta-wave time by approximately 80 msec and will produce a greater degree of ventricular preexcitation because the ventricles will begin depolarization earlier via the bypass tract. An example of how this technique can be used to localize the bypass tract is shown in Figure 10-50 in which high-right atrial pacing results in preexcitation with a P-to-delta-wave interval of 95 msec, while coronary sinus pacing produces a shortening of the P-to-delta-wave interval to 50 msec and a greater degree of preexcitation. This effect is particularly valuable in localizing the bypass tract if it is incapable of retrograde conduction, thereby prohibiting localization by atrial mapping during SVT or ventricular pacing (see following discussion).
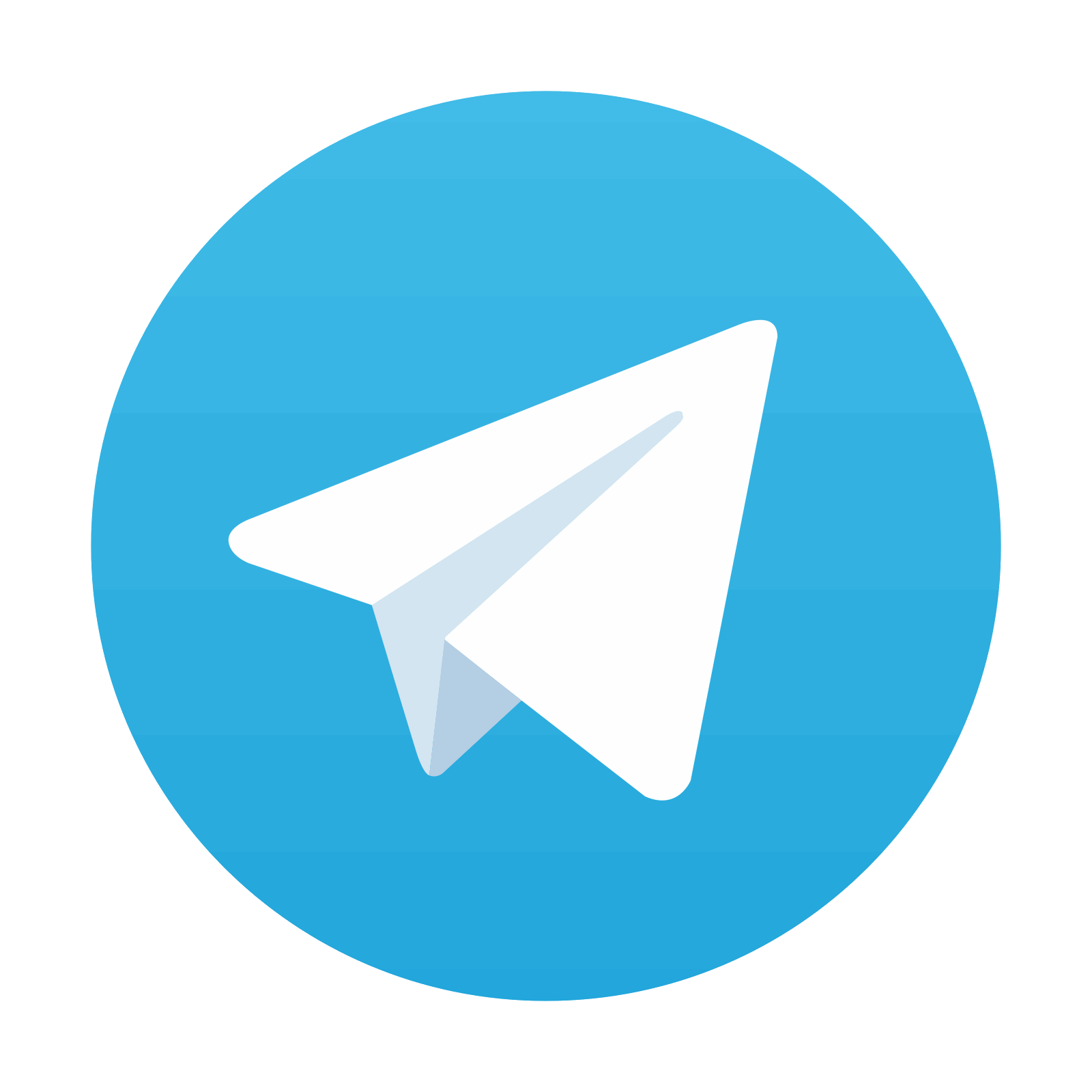
Stay updated, free articles. Join our Telegram channel

Full access? Get Clinical Tree
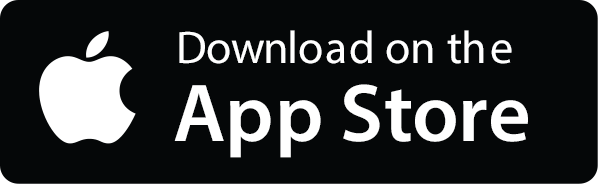
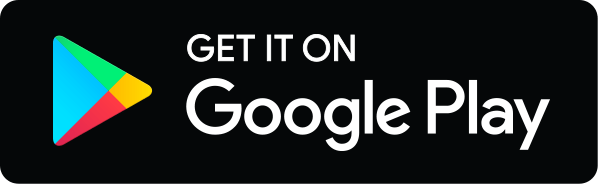